Pulmonary Stenosis
Lourdes R. Prieto
Larry A. Latson
Congenital obstruction to right ventricular outflow is most commonly due to pulmonary valve stenosis, but may also be intracavitary or supravalvar and involve the main and branch pulmonary arteries. Pulmonary stenosis at some level, with and without other associated lesions, occurs in 25% to 30% of all patients with congenital heart disease. This chapter discusses all levels of pulmonary stenosis with intact ventricular septum.
Isolated Valvar Pulmonary Stenosis
Isolated pulmonary valve stenosis is found in 80% to 90% of all patients with right ventricular outflow obstruction. It was described in 1761 by John Baptist Morgagni (1) and is found in 8% to 10% of patients with congenital heart disease.
Familial occurrence of pulmonary stenosis has been reported. Campbell (2) found a 2.1% incidence of cardiac disease, usually pulmonary stenosis or tetralogy of Fallot, in siblings of patients with pulmonary stenosis. In the Second Natural History Study of Congenital Heart Defects, the occurrence of definite and possible congenital cardiac defects in 1,356 siblings of 449 patients with valvar pulmonary stenosis was 1.1% and 2.1%, respectively (3).
Embryology and Pathology
The process of cardiac valve development begins with migration of a subset of endothelial cells lining the inner layer of the developing heart tube into the extracellular matrix that separates the inner endothelial layer from the outer layer of myocardium, forming cardiac cushions precisely in the areas overlying the future atrioventricular canal and outflow tract. The cells forming these cushions continue to proliferate and differentiate into mesenchymal cells. Whereas the mitral and tricuspid valves are derived only from endocardial cushion tissue, the final development of the aortic and pulmonary valves involves migration of neural crest cells from the branchial arches to the distal outflow tract where aortopulmonary septation will take place (4,5). Further remodeling of these cushions culminates in the formation of thin, tapered leaflets with a single endothelial cell layer and a central matrix of collagen, elastin, and glycosaminoglycans.
Normal valve development involves several signaling pathways that tightly regulate endothelial cell differentiation and remodeling, and is also dependent on the interaction between these endothelial cells, the extracellular matrix, and the surrounding myocardium (6). The complex interaction between these pathways can be disrupted at various levels, resulting in a malformed valve. For example, deletion of CXCR7, a G-protein–coupled receptor found in cushion mesenchymal cells in the developing heart, resulted in aortic and pulmonary valve stenosis in mouse embryos due to excessive proliferation of mesenchymal valve cells associated with increased bone morphogenetic protein signaling (7). Deletion of PTPN11 exon 2, which encodes the protein tyrosine phosphatase SHP-2 involved in another signaling pathway, results in dysplastic outflow valves (8). Notably, PTPN11 mutations are found in patients with Noonan syndrome and LEOPARD syndrome, both manifesting pulmonary valve stenosis (9,10,11). Research to date has elucidated disruptions that affect the development of both the pulmonary and aortic valves, but factors resulting specifically in pulmonary valve abnormalities have yet to be recognized. The pathways controlling the later stages of valve remodeling that would affect one or the other semilunar valve are not yet known.
In the classic form of pulmonary valve stenosis, the valve is conical or dome shaped, and two to four raphes may be visible, but there is no separation into valve leaflets (Fig. 39.1) (12). Less commonly, the valve may be diffusely thickened with one, two, or three leaflets and commissural fusion. A distinct pathology termed pulmonary valve dysplasia has been described in 10% to 20% of patients (13). Dysplastic valves are trileaflet with markedly thickened cusps composed of disorganized myxomatous tissue and little, if any, fusion (Fig. 39.2). The valve annulus is usually hypoplastic. This entity is found in most patients with Noonan syndrome and may be seen in nonfamilial cases.
Secondary changes in the right ventricle (RV) and pulmonary arteries can occur as a result of pulmonary valve obstruction. The RV, particularly the infundibular region, becomes diffusely hypertrophied. Hypertrophy of the infundibulum can produce dynamic subvalvar obstruction (see Fig. 39.1). At autopsy, small myocardial infarctions frequently have been seen in the subendocardium of the right ventricular free wall and papillary muscles in patients with severe pulmonary stenosis. Thickening of the tricuspid valve and chordal attachments may be present, and the valve may become regurgitant. The right atrium may be thick and dilated as a result of the increased pressure necessary to fill the hypertrophic RV. In many cases, a patent foramen ovale or, less often, an atrial septal defect is seen.
Most patients develop poststenotic dilation of the pulmonary artery trunk, sometimes extending to the proximal left pulmonary artery (LPA). One notable exception to this finding is in patients with dysplastic pulmonary valves. The degree of dilation is not necessarily proportional to the severity of obstruction, often being more pronounced in mild cases. Poststenotic dilation may result from the high-velocity jet of flow ejected through the small valve orifice.
Physiology
The main physiologic effect of valvar pulmonary stenosis is a rise in right ventricular pressure proportional to the severity of obstruction. This elevation of right ventricular pressure is accompanied by an increase in muscle mass that occurs by one of two mechanisms, depending on the stage of development. Work in animal models has shown that the fetal and neonatal myocardium responds to increased afterload by hyperplasia of the muscle cells with a concomitant increase in the number of capillaries (14). In contrast, the adult myocardium responds with hypertrophy of the existing fibers, with no change in the capillary network. Thus, the neonatal myocardium may be better adapted to generate the high pressures necessary to overcome severe obstruction. Increased muscle mass may enable the hypertensive RV to maintain a normal stroke volume. If the size of the stenotic orifice remains fixed, however, the degree of obstruction becomes relatively more severe as the patient grows. The RV eventually may dilate and fail. This process is exacerbated by the development of tricuspid regurgitation in many patients with severe pulmonary stenosis. Right ventricular failure may occur in infancy if severe neonatal obstruction is present. As right ventricular output decreases with a failing ventricle, adequate tissue oxygenation can be maintained only by increasing tissue oxygen extraction. Any increase in oxygen demand, such as during exercise, may result in frank peripheral cyanosis. In patients with a patent foramen ovale or atrial septal defect, central cyanosis is observed as a result of right-to-left atrial shunting when the right atrial pressure exceeds the left atrial pressure. Progressive hypertrophy and decreased compliance of the RV, or myocardial failure with subsequent dilation, may lead to central cyanosis in some initially well-compensated patients.
When the degree of valvar pulmonary stenosis is severe enough to cause a decrease in fetal right ventricular output, a larger than normal atrial right-to-left shunt is established in utero. This condition has been termed critical pulmonary stenosis (15). The RV is often hypoplastic because of severe hypertrophy and the effects of reduced flow through the RV during development (Fig. 39.3, Video 39.1). At birth, affected infants are cyanotic and have systemic or suprasystemic right ventricular pressure. Even if the stenosis is relieved, right-to-left atrial shunting and cyanosis often persist
for months after the stenosis is relieved, until there is a decrease in the right ventricular hypertrophy and an increase in right ventricular size.
for months after the stenosis is relieved, until there is a decrease in the right ventricular hypertrophy and an increase in right ventricular size.
Manifestations
Clinical Features
Most patients with valvar pulmonary stenosis are asymptomatic, and the diagnosis usually is made when a pathologic murmur is detected on routine examination. Symptoms are rarely present in childhood but become more common with increasing age in patients with moderate to severe stenosis. Initial symptoms usually consist of exertional dyspnea and fatigue due to inability of the RV to increase its output in response to exertion. If the stenosis is not relieved, right heart failure may ensue. Cyanosis may be observed in patients with an atrial communication. Occasionally, patients with moderate to severe stenosis may experience chest pain, syncope, and even sudden death with strenuous exercise. Decreased myocardial perfusion caused by inadequate cardiac output during exercise, leading to ischemia and ventricular arrhythmias, is thought to be the mechanism for these events.
Children with valvar pulmonary stenosis usually exhibit normal growth and development regardless of the severity of obstruction. Squatting is extremely rare even in patients with significant central cyanosis. If squatting is present, other diagnoses, especially tetralogy of Fallot, should be sought.
Infants with critical pulmonary stenosis are cyanotic at birth, and the cyanosis may be severe enough to be life threatening (15). Although the right ventricular cavity is often relatively hypoplastic, the atrial communication is usually large enough to maintain cardiac output and prevent right heart failure at the expense of cyanosis. Symptoms of right-sided heart failure may be seen in some newborns with significant tricuspid regurgitation or may develop in untreated infants if the atrial communication becomes inadequate with growth.
The auscultatory findings in valvar pulmonary stenosis are quite distinctive, often allowing the diagnosis to be made based only on the physical examination (Fig. 39.4) (16). The first heart sound is normal, and in patients with mild or moderate stenosis, it is followed by a pulmonary ejection click. The click corresponds to the time when the doming pulmonary valve reaches its open position. The more severe the stenosis, the earlier in systole the click occurs, until it merges with the first heart sound and becomes inaudible. The intensity of the click varies with respiration, decreasing during inspiration and increasing during expiration.
The systolic murmur of valvar pulmonary stenosis is ejection in quality and maximal at the upper left sternal border. It may radiate over the entire precordium and neck, but characteristically is heard in the back. In general, the intensity of the murmur increases with the severity of obstruction. Mild stenosis is associated with murmurs of grade 3 or lower, and moderate to severe stenosis with grade 4 or louder. Patients with severe stenosis and right heart failure may have an unusually soft murmur because of low cardiac output. The length of the murmur is proportionately related to the duration of right ventricular ejection, which is determined primarily by the severity of obstruction. With more severe obstruction, the murmur peaks later in systole. In mild stenosis, the murmur is relatively short and peaks at or before midsystole (Fig. 39.4). In moderate stenosis, the murmur ends at or slightly after the aortic component of the second heart sound, which remains audible. With severe obstruction, the murmur extends beyond the aortic closure sound, which may become inaudible. A soft, early diastolic murmur of mild pulmonary insufficiency is rarely heard and usually results from progressive pulmonary trunk dilation.
Patients with mild pulmonary valve stenosis have normal “a” waves and therefore normal jugular venous pulsations. With more severe obstruction, the “a” wave becomes progressively larger, and abnormal pulsations may be felt both in the jugular venous pulse and in the liver. In infants and children, jugular venous pulsations are often difficult to appreciate, even in the presence of large “a” waves.
A prominent right ventricular systolic impulse and a systolic thrill almost always can be palpated in patients with severe pulmonary stenosis. Typically, the thrill is located at the second to third intercostal space, but it may also be felt at the suprasternal notch. The thrill may be absent in young infants with severe stenosis and in patients with congestive heart failure and low cardiac output. When the stenosis is mild, the precordium is quiet and a thrill is not present.
The second heart sound in pulmonary stenosis is usually split, and the degree of splitting is proportional to the degree of stenosis. The split may become fixed in severe stenosis as a result of a fixed stroke volume. The intensity of the pulmonary component of the second heart sound typically decreases with increasing obstruction, which may make the splitting difficult to appreciate. Occasionally, in mild stenosis, the pulmonary closure sound is louder than normal because of marked dilation of the pulmonary artery trunk. A fourth heart sound often is heard at the lower left sternal border in patients with severe stenosis. When a third heart sound is heard, the presence of an atrial septal defect should be suspected.
The cardiac examination in infants with critical pulmonary stenosis may differ from that of older patients with severe obstruction. The systolic murmur of pulmonary stenosis may be deceptively soft as a result of decreased flow across the pulmonary valve in the presence of an atrial right-to-left shunt. A holosystolic murmur of
tricuspid regurgitation may be present lower along the left sternal border, or a patent ductus murmur may be audible along the mid to upper sternal border. Either of these may be the predominant murmur. The pulmonary closure sound is typically absent. Significant cardiomegaly may be detected by precordial palpation, most commonly due to right atrial enlargement.
tricuspid regurgitation may be present lower along the left sternal border, or a patent ductus murmur may be audible along the mid to upper sternal border. Either of these may be the predominant murmur. The pulmonary closure sound is typically absent. Significant cardiomegaly may be detected by precordial palpation, most commonly due to right atrial enlargement.
Electrocardiographic Features
The electrocardiogram can be somewhat useful in assessing the severity of obstruction in patients with pulmonary valve stenosis. As many as 40% to 50% of patients with mild stenosis have a normal electrocardiogram. Slight rightward deviation of the QRS frontal axis is often the only abnormality. The R wave amplitude in the right precordial leads rarely exceeds 10 to 15 mm. A right ventricular conduction delay is commonly present.
In moderate pulmonary stenosis, the electrocardiogram is almost always abnormal, with only 10% of patients having a normal tracing. Right axis deviation is usually present. The R:S ratio in V1 is usually >4:1, and the R wave is typically <20 mm. The T waves in the right precordial leads are upright in approximately 50% of patients.
In severe pulmonary stenosis, the electrocardiogram is rarely normal (Fig. 39.5). The mean frontal QRS axis is usually >110 degrees, and not uncommonly, extreme right axis deviation is seen. A pure R, RS, or QR is the usual pattern in the right precordial leads, and the R wave is usually >20 mm. The R:S ratio in V6 may be <1.0. The T waves may be upright or inverted in the right precordial leads, and the P waves are abnormally tall and peaked in lead 2 and in the right precordial leads, indicating right atrial enlargement.
It is possible to estimate the right ventricular pressure in patients between 2 and 20 years of age if a pure R wave is present in lead V4R or V1. The height of the R wave in millimeters, multiplied by 5, approximates the right ventricular systolic pressure in millimeters of mercury (14). Occasionally, infants with severe stenosis, in whom the RV may be hypoplastic, have a more leftward axis than expected (in the range of +30 to +70 degrees) as well as evidence of left ventricular hypertrophy. A superior axis, sometimes accompanied by a conduction abnormality of the left bundle, also has been described in some patients with pulmonary stenosis. There may be a correlation between these findings and Noonan syndrome, with its associated cardiomyopathy.
Radiographic Features
The most distinctive feature in valvar pulmonary stenosis is a prominent main pulmonary artery segment resulting from poststenotic dilation of the pulmonary trunk and sometimes the LPA (Fig. 39.6). This finding is present in 80% to 90% of cases, but it may be absent in infants, in patients with dysplastic pulmonary valve, and in cases of rubella syndrome. The apex of the heart is usually rounded and points downward. The right atrial segment may be prominent, more commonly in patients with associated
tricuspid regurgitation or atrial septal defect. A left aortic arch is virtually always present.
tricuspid regurgitation or atrial septal defect. A left aortic arch is virtually always present.
Heart size and pulmonary vascularity are usually normal in patients with mild to moderate stenosis. In the absence of right ventricular failure, even with severe obstruction, only mild cardio megaly is seen. When heart failure develops, marked cardiomegaly results due to right atrial and right ventricular enlargement, and pulmonary vascularity is decreased as a result of a reduction in pulmonary flow. Cardiomegaly is commonly present in infants with severe or critical pulmonary stenosis, and pulmonary vascularity is severely reduced because of the large atrial right-to-left shunt (Fig. 39.7).
Two-Dimensional Echocardiography
The 2-D echocardiogram clearly demonstrates the typical features of the stenotic pulmonary valve from the standard and high parasternal short-axis and long-axis views as well as the subcostal sagittal views (Fig. 39.8, Video 39.2). The valve leaflets usually appear prominent because of thickening. Systolic motion is restricted, with inward curving of the tips of the leaflets, known as doming. Associated features, such as poststenotic dilation of the main and branch pulmonary arteries, also are easily recognized. Right ventricular hypertrophy, contractility of the RV, as well as anatomy and function of the tricuspid valve should be assessed (Video 39.3). Evidence of dynamic subpulmonary stenosis should be sought, but the severity may be impossible to estimate in the presence of more than mild valvar stenosis.
The diagnosis of dysplastic pulmonary valve usually can be ascertained by echocardiography (Video 39.4). The leaflets appear thickened and immobile, without the characteristic doming seen in typical cases. The pulmonary valve annulus is hypoplastic, and supraannular narrowing of the proximal main pulmonary artery is often present. The poststenotic pulmonary artery dilation seen in classic cases is absent.
![]() Figure 39.8 A: Parasternal short-axis view from a 1-day-old infant with critical pulmonary valve (PV) (arrow) stenosis (same patient as in Fig. 39.3). Note the thickened, “doming” leaflets in systole. The PV annulus is hypoplastic compared with the aortic valve annulus. There is poststenotic dilation of the main pulmonary artery (MPA). B: Color Doppler through the stenotic PV shows a mosaic pattern indicating high-velocity, turbulent flow originating at the level of the PV. A, anterior; AO, aorta; R, right; RV; right ventricle. |
Doppler Evaluation
The Doppler echocardiogram allows quantitative assessment of severity of pulmonary valve stenosis by estimating the pressure
drop across the pulmonary valve (Fig. 39.9). The simplified Bernoulli equation P = 4V22 is used, where P is the peak instantaneous pressure gradient (mm Hg), across the obstructed pulmonary valve, and V2 is the peak flow velocity (m/s), distal to the obstructive orifice. If significant subpulmonary stenosis coexists, V1 (the peak flow velocity proximal to the obstruction) must be taken into account. The Doppler beam must be aligned parallel with the main pulmonary artery trunk or the direction of the flow jet as seen on color Doppler. If tricuspid regurgitation is present, the Doppler technique can be used to calculate the pressure difference (P) between the right atrium and RV by measuring the peak flow velocity (V) of the tricuspid insufficiency jet. Right ventricular pressure then can be estimated by adding the pressure gradient to the estimated right atrial pressure.
drop across the pulmonary valve (Fig. 39.9). The simplified Bernoulli equation P = 4V22 is used, where P is the peak instantaneous pressure gradient (mm Hg), across the obstructed pulmonary valve, and V2 is the peak flow velocity (m/s), distal to the obstructive orifice. If significant subpulmonary stenosis coexists, V1 (the peak flow velocity proximal to the obstruction) must be taken into account. The Doppler beam must be aligned parallel with the main pulmonary artery trunk or the direction of the flow jet as seen on color Doppler. If tricuspid regurgitation is present, the Doppler technique can be used to calculate the pressure difference (P) between the right atrium and RV by measuring the peak flow velocity (V) of the tricuspid insufficiency jet. Right ventricular pressure then can be estimated by adding the pressure gradient to the estimated right atrial pressure.
Several studies have documented excellent correlation between the Doppler-derived gradient and that obtained by direct pressure measurement at catheterization (17,18). It should be recognized, however, that the Doppler-derived peak instantaneous pressure gradient exceeds the peak-to-peak pressure gradient measured at catheterization by a small amount. In pulmonary valve stenosis, this difference is clinically insignificant, and the two measurements are close enough to obviate the need for diagnostic catheterization in most patients until intervention becomes necessary.
The development of color Doppler 2-D echocardiography has contributed to the diagnostic accuracy of pulmonary valve stenosis by demonstrating an abnormal flow pattern originating at the stenotic valve (see Fig. 39.8). Normal flow is coded as red or blue, depending on whether it is directed toward or away from the transducer, respectively. High-velocity, turbulent flow through stenotic lesions appears as a mosaic jet with green, yellow, and other shades. Visualization of the jet by color also facilitates optimization of the alignment between the Doppler sample volume and the direction of flow, increasing the accuracy of the measured gradient.
Cardiac Catheterization
The refinement of 2-D and Doppler echocardiography over the past three decades has had a dramatic impact on the use of cardiac catheterization in the management of pulmonary valve stenosis. Because diagnosis and exclusion of other significant lesions can now be accomplished noninvasively, the role of catheterization has become largely therapeutic. Balloon pulmonary valvuloplasty has supplanted surgical valvotomy as the treatment of choice for this lesion (19).
Hemodynamics
The most important measurements made at catheterization are the right ventricular pressure compared with systemic arterial pressure and the pressure gradient across the pulmonary valve. A resting right ventricular pressure >30 to 35 mm Hg and a pressure gradient across the pulmonary valve of >10 mm Hg are considered abnormal. An end-hole catheter is used to obtain carefully the withdrawal pressure recordings from the pulmonary artery to the body of the RV to assess the severity and location of any stenoses. In the presence of associated infundibular obstruction, pressure gradients are encountered across the pulmonary valve and also across the infundibulum. In the infundibular chamber, the pressure curve is usually triangularly shaped, with a fall in the late systolic pressure resulting from progressive narrowing of the outflow tract during ventricular contraction (20). The right ventricular end-diastolic pressure may be normal, but usually it is elevated with severe obstruction or right ventricular failure. The right atrial pressure is normal in mild to moderate obstruction, but tall right atrial “a” waves usually are seen with severe obstruction. Pulmonary artery pressure is normal in mild cases, but it is decreased and dampened in severe cases. This depression of pulmonary artery pressure is more marked in the main pulmonary artery just beyond the valve than further distally because of the Bernoulli effect. The increase in flow velocity across the stenotic valve causes more of the total energy to be expressed as kinetic energy, necessitating a drop in pressure to maintain the total energy constant. As blood velocity decreases further downstream, pressure recovery is observed.
In patients with relatively normal cardiac output, classification of severity of pulmonary stenosis is routinely based on measurements of right ventricular pressure and valve gradient. Mild stenosis is characterized by a right ventricular pressure less than half the left ventricular pressure or a valve gradient <35 to 40 mm Hg. In moderate stenosis, the right ventricular pressure is greater than half but <75% of the left ventricular pressure, or the gradient is 40 to 60 mm Hg. Severe stenosis is defined as a right ventricular pressure ≥75% of the left ventricular pressure or a gradient >60 to 70 mm Hg.
During exercise, an increase in the pressure gradient across the stenotic valve occurs primarily because an increase in the volume of flow and the rate of contraction of the RV leads to an increase in flow velocity across the obstruction. Patients with severe stenosis are unable to increase their stroke volume and rely solely on an increase in heart rate to augment cardiac output during exercise. A marked increase in heart rate can be detrimental in patients with severe obstruction because of the resultant shortening of diastolic filling time. The impedance to filling of the hypertrophied, stiff RV can lead to a decrease in cardiac output and systolic blood pressure when marked tachycardia develops. Decreased right ventricular compliance is further evidenced by elevated right ventricular end-diastolic pressure at rest and abnormal increase with exercise observed in patients with severe obstruction (21).
Angiocardiography
Angiocardiography provides information about the location and severity of pulmonary stenosis that is invaluable for diagnostic and therapeutic purposes. The anatomy of the pulmonary valve and associated features can be shown best by right ventricular angiography in the anteroposterior view with the tube-angled cephalad and in the lateral view (Fig. 39.10, Video 39.5). Characteristically, the pulmonary valve leaflets are mildly thickened and dome in systole, returning to the normal position in diastole. A narrow jet of contrast is seen crossing the valve, usually along the anterosuperior margin of the main pulmonary artery, resulting in poststenotic dilation of this vessel. The pulmonary valve annulus is typically of normal size, but it may be hypoplastic in infants with severe stenosis. The right ventricular cavity is usually of normal size, but it may be hypoplastic in newborns with critical pulmonary stenosis, or it may be dilated in the presence of congestive heart failure. The walls usually are thickened with prominent trabeculations. Narrowing of the right ventricular outflow tract (RVOT) as a result of infundibular hypertrophy is usually seen in severe obstruction. Right ventricular function is normal except in severe cases with ventricular failure.
The angiographic features of dysplastic pulmonary valve stenosis differ in several ways from those of typical pulmonary valve stenosis (see Fig. 39.2, Video 39.6) The valve leaflets are markedly thickened and relatively immobile, with little excursion during the cardiac cycle. The annulus is hypoplastic, and this hypoplasia usually extends to the proximal main pulmonary artery. There is often the appearance of tethering of the valve leaflets to the main pulmonary artery wall at the sinotubular junction. Poststenotic dilation of the main or branch pulmonary arteries usually is not seen. Often the origin of the right pulmonary artery (RPA) from the main pulmonary artery has a more proximal than normal position.
Differential Diagnosis
The diagnosis of pulmonary valve stenosis with intact ventricular septum is usually readily made by careful auscultation and supportive electrocardiographic and radiographic features, but certain conditions must be considered in the differential diagnosis. Mild pulmonary stenosis should be differentiated from idiopathic dilation of the main pulmonary artery, atrial septal defect, peripheral pulmonary arterial stenosis, mitral valve prolapse, straight back syndrome, mild aortic stenosis, and innocent murmurs. Moderate to severe pulmonary stenosis without cyanosis should be distinguished from ventricular septal defect (VSD) with or without associated pulmonary stenosis and moderate aortic stenosis. When cyanosis is present in severe stenosis, tetralogy of Fallot and pulmonary atresia with intact ventricular septum must be excluded. Ebstein anomaly of the tricuspid valve occasionally mimics severe pulmonary stenosis in the newborn.
Pulmonary Stenosis Associated with Systemic Diseases
Congenital heart disease is seen in approximately 50% of patients with Noonan syndrome (10). The most common lesion is pulmonary stenosis due to pulmonary valve dysplasia. Hypertrophic cardiomyopathy of the left ventricle with or without pulmonary stenosis is also found in up to 25% of cases. The cardiac examination in these patients is often atypical and does not reflect the severity of the pulmonary stenosis. The ejection click is usually absent, and a soft, relatively short murmur may be heard despite severe stenosis. The electrocardiogram usually shows superior axis deviation even in patients without apparent cardiomyopathy.
Intracardiac tumors or extrinsic lesions compressing cardiac structures are rare causes of pulmonary stenosis. Echocardiography or magnetic resonance imaging (MRI) usually can establish the diagnosis. Multiple lentigines syndrome, or LEOPARD syndrome, has been associated with pulmonary valve and pulmonary artery stenosis. Neurofibromatosis rarely causes right ventricular outflow obstruction, as can deposits from glycogen storage diseases and gout. Carcinoid disease of the bowel may be associated with endocardial fibroelastosis of the RV with involvement of the pulmonary and tricuspid valves, leading to stenosis.
Treatment
Balloon Valvuloplasty
The technique of percutaneous balloon pulmonary valvuloplasty was described initially by Kan et al. (19). Since then, many other workers have reported the successful application of this technique to treat patients with moderate to severe pulmonary valve stenosis. This procedure led the way to the era of pediatric catheter intervention, which has been expanded to a multitude of other lesions over the past three decades.
The technique of balloon valvuloplasty is relatively straightforward (Fig. 39.11). After obtaining appropriate hemodynamic and angiographic information about severity and location of obstruction, an exchange guidewire is introduced through an end-hole catheter and positioned in the distal LPA or RPA. A balloon is chosen that is 20% to 30% larger than the angiographically measured pulmonary valve annulus, and it is positioned over a guidewire with the valve at its midpoint. As the balloon is inflated, a waist from the stenotic valve should be observed initially and disappear at full inflation (Fig. 39.12, Video 39.7). In larger patients with an annulus diameter of more than 20 mm, the double-balloon technique may be necessary, with simultaneous inflation of two angioplasty balloons. A method to calculate the effective diameter of two balloons was described by Radtke et al. (22). Following balloon dilation, a careful pullback with an end-hole catheter is performed to evaluate the degree and site of any residual obstruction. Not infrequently, a gradient is measured across the infundibulum that may have been masked before valvuloplasty by the distal valvar obstruction. This type of dynamic infundibular stenosis typically resolves over time as the hypertrophied muscle regresses.
![]() Figure 39.11 Schematic illustration of the balloon valvuloplasty catheter positioned across the pulmonary valve. The wire should be positioned in the distal left or right pulmonary artery. |
Shortly after the introduction of pulmonary valvuloplasty in children, the procedure was performed successfully in neonates with critical pulmonary valve stenosis (23). Before catheterization, these patients usually require stabilization and initiation of a prostaglandin E1 infusion to maintain ductal patency. Several technical advances, such as the introduction of low-profile balloons, have increased the success and safety of balloon dilation in this group of patients, such that it is now considered the treatment of choice (24,25,26). The use of angled-tip catheters and high-torque wires has facilitated crossing the tiny pulmonary valve orifice. Initial dilation with a small coronary angioplasty balloon to enlarge the orifice and subsequent dilations with progressively larger balloons allow adequate relief of obstruction in most neonates (27). Placement of the guidewire through the patent ductus into the descending aorta, rather than in the LPA, allows a more stable wire position. Creating a “rail” by snaring the wire in the descending aorta can facilitate introduction of the desired balloon catheter through the orifice (Fig. 39.13) (28).
The short- and intermediate-term results of pulmonary valvuloplasty in children and adults with typical pulmonary valve stenosis have been excellent (29,30,31,32,33). The most comprehensive series to date reported on 533 patients with a median follow-up of 33 months and a maximum follow-up of 8.7 years (33). The morphology of the pulmonary valve was typical in 82% of patients, dysplastic in 13%, and complex (postsurgical valvotomy, associated with other significant lesions) in 5%. A good outcome, defined as a residual Doppler gradient at follow-up of 36 mm Hg or lower without the need for repeat procedures, was achieved in 77% of the total group and in 85% of those with typical valve morphology. In contrast, 65% of patients with dysplastic pulmonary valve had a suboptimal outcome. Independent predictors of suboptimal intermediate-term outcome were a small annular size (characteristic of patients with dysplastic valves), a higher immediate residual gradient, an earlier year of the initial valvuloplasty, and smaller balloon: annulus ratio (BAR) in those with typical valves. Balloons used in this study were a mean of 112 ± 20% of the annular diameter. The latter two predictors suggest that gradient reduction, at least for typical pulmonary valve morphology, could be improved by greater experience and the use of BARs >1.2, as has been borne out by smaller studies (31,32). The use of excessively large balloons, however, has been associated with a higher rate of late severe pulmonary insufficiency (32,34).
Long-term outcomes have been reported on smaller series of patients with a mean follow-up of 11.9 years and a range up to 19.3
years (35). In this group of 134 patients, in whom the average BAR was 1.3, the results have been similar with persistent gradient reduction in the majority of patients and a lower success rate in those with dysplastic valves. Freedom from any reintervention at 1, 5, 10, and 15 years were 90%, 83%, 83%, and 77%, respectively. Only 17 patients had surgical intervention at some point during follow-up to relieve valvar, subvalvar, or supravalvar obstruction, and 11 of those had dysplastic valves. Two additional children had surgical intervention for severe tricuspid regurgitation at 11 and 12 years of age. At operation, a flail anterior leaflet was found in both, possibly caused by a tear at the time of valvuloplasty. Repeat balloon valvuloplasty was performed in 11 children, 2 of whom eventually underwent surgery due to the development of subpulmonary stenosis. Risk factors for reintervention were younger age and lower body surface area, a smaller pulmonary valve annular diameter Z-score, a higher pulmonary valve gradient at the initial procedure, and the presence of Noonan syndrome. Clinical status for the entire cohort was excellent at a mean follow-up of 11.9 years, with no reported arrhythmias and only two patients in New York Heart Association (NYHA) class II.
years (35). In this group of 134 patients, in whom the average BAR was 1.3, the results have been similar with persistent gradient reduction in the majority of patients and a lower success rate in those with dysplastic valves. Freedom from any reintervention at 1, 5, 10, and 15 years were 90%, 83%, 83%, and 77%, respectively. Only 17 patients had surgical intervention at some point during follow-up to relieve valvar, subvalvar, or supravalvar obstruction, and 11 of those had dysplastic valves. Two additional children had surgical intervention for severe tricuspid regurgitation at 11 and 12 years of age. At operation, a flail anterior leaflet was found in both, possibly caused by a tear at the time of valvuloplasty. Repeat balloon valvuloplasty was performed in 11 children, 2 of whom eventually underwent surgery due to the development of subpulmonary stenosis. Risk factors for reintervention were younger age and lower body surface area, a smaller pulmonary valve annular diameter Z-score, a higher pulmonary valve gradient at the initial procedure, and the presence of Noonan syndrome. Clinical status for the entire cohort was excellent at a mean follow-up of 11.9 years, with no reported arrhythmias and only two patients in New York Heart Association (NYHA) class II.
The significantly lower success rate for patients with dysplastic pulmonary valves is not surprising given the anatomic features of these valves. The mechanism of obstruction relief in patients with typical, doming pulmonary valves has been shown to be commissural splitting in the majority of cases (36,37). In dysplastic valves, the leaflets may be markedly thickened and myxomatous with little commissural fusion. In addition, the annulus and main pulmonary artery are usually hypoplastic, further limiting the effectiveness of valvuloplasty. Several studies, however, documented adequate relief of obstruction in 35% to 65% of patients with dysplastic valves (30,32,33). Accurate predictors of success before valvuloplasty have not been identified. Thus, although controversy remains, the usual practice is to offer balloon valvuloplasty as a first line of treatment and proceed to surgical valvotomy if balloon valvuloplasty is unsuccessful. In neonates with critical pulmonary valve stenosis, the success of pulmonary valvuloplasty at intermediate-term follow-up also has been lower than in older patients, regardless of valve morphology (24,25,26,34,38,39). With a mean follow-up of approximately 3 to 6 years for most studies, varying success rates have been reported, depending on how success is defined. Early in the experience, procedural failure was often due to an inability to cross the severely stenotic pulmonary valve, but with the availability of preformed catheters, better wires, and lower-profile balloons, dilation can now be accomplished in nearly 100% of patients. If dilation was accomplished, immediate effective gradient reduction usually was achieved in more than 90% of patients. Despite successful relief of obstruction, 5% to 10% of these patients were unable to sustain sufficient forward flow through the pulmonary valve to maintain adequate saturations because of their severely hypertrophic, noncompliant, and sometimes hypoplastic RVs.
If discontinuation of prostaglandin E1 and subsequent ductal constriction are not tolerated immediately after valvuloplasty, these infants can be maintained on prostaglandin for as long as 2 to 3 weeks while intermittently assessing whether constriction of the ductus is tolerated with O2 saturations remaining 70% or greater. If ductal dependency persists after that time, either a surgical aortopulmonary shunt or stenting of the ductus can be done. In rare instances, balloon atrial septostomy is also necessary to ensure adequate cardiac output. Neonates who remain cyanotic following valvuloplasty, with or without a surgical shunt or ductal stent, often demonstrate progressive resolution of their cyanosis over weeks to months as right ventricular compliance improves and the atrial right-to-left shunt diminishes. Ultimately, those in whom a surgical shunt was created can undergo shunt closure either surgically or by transcatheter techniques. Atrial septal defect closure also may be necessary, depending on the size of the atrial communication. Recurrent valvar stenosis necessitating repeat valvuloplasty may occur within months of the initial procedure in about 10% of these patients and subsequently may afford long-term relief of obstruction.
Stenting of the ductus is increasingly accepted as an alternative to a surgical shunt in patients who remain ductal dependant following valvuloplasty. The use of a stent to maintain ductal patency was first reported in the early 1990s (40,41). Available data document gradual narrowing of the stent lumen over a period of months, during which time there is typically sufficient growth of the right heart and improved right ventricular compliance to obviate the need for ductal flow (42,43). Although studies comparing surgical aortopulmonary shunt to ductal stenting have not been performed, a multicenter experience with a fairly large group of patients over the past two decades suggests that ductal stenting should be the preferred approach in this patient population (44). The ductus in patients with critical pulmonary stenosis is horizontal and tubular, which has been shown to be ideal anatomy for stenting (Video 39.8). Transcatheter techniques can also be used to close the atrial septal defect when necessary, potentially eliminating the need for any surgical intervention.
Maintaining an unobstructed pulmonary valve is important in these infants to optimize forward flow through the RV as a stimulus for growth and to allow resolution of the right ventricular hypertrophy. About 15% to 20% of neonates with critical pulmonary stenosis ultimately undergo surgical intervention to relieve either valvar stenosis resistant to dilation or subvalvar obstruction (25,26,39,45). The strongest determinant of the need for surgical intervention has been found to be the presence of subvalvar stenosis, followed by the annular dimension and morphology of the pulmonary valve. A smaller indexed tricuspid valve annulus also confers a higher risk of surgical intervention (39). In a small minority, persistent right ventricular hypoplasia precludes a two-ventricle repair.
The incidence of major complications from pulmonary valvuloplasty is exceedingly low in children and adults but is higher in infants and neonates. The largest study to date from the Valvuloplasty and Angioplasty Registry reported only 2 deaths from a total of 822 patients (0.2%) (29). The causes of death were laceration of the inferior vena cava–iliac vein junction during balloon withdrawal in a 5-day-old infant and tearing of the pulmonary valve annulus during balloon inflation with a reportedly properly sized balloon in a 12-month-old infant. Other major complications occurred in three patients, including perforation of the RVOT resulting in tamponade and tricuspid regurgitation requiring surgical intervention. The incidence of minor complications was 1.3%, including vein thrombosis, vein tears, and arrhythmias. In neonates, mortality was approximately 3% and was due to various causes, including venous injury, myocardial dissection, and development of necrotizing enterocolitis. The incidence of significant morbidity in neonates was on average around 10%. Specific complications reported include wire perforation of the RVOT with or without tamponade, stroke, seizures, necrotizing enterocolitis, endocarditis, septic shock, and abrupt closure of the ductus despite prostaglandin infusion requiring urgent aortopulmonary shunt placement.
Most patients who have been treated with pulmonary valvuloplasty have some degree of pulmonary regurgitation (PR) (31,32,33). The incidence of moderate PR early after valvuloplasty has been variably reported as <5% to as much as 24% at intermediate-term follow-up. This relatively wide range is at least partially a result of the lack of standardized grading criteria for severity of PR. Longer follow-up and improved grading methods have shown greater degrees of PR (35,46), particularly in patients undergoing the procedure as neonates. PR has been shown to progress over time in the same group of patients (35). At a mean follow-up of 0.9 years, 22% of patients had moderate and 2% had severe PR, while at 11.9 years of follow-up, the percentage of patients with moderate PR increased to 40%, and with severe PR to 17%. Severe PR was diagnosed by echocardiogram when there was reverse flow in the branch pulmonary arteries and a nonrestrictive regurgitant Doppler signal across the RV outflow tract. Right ventricular enlargement was significantly greater in children with severe PR. Multivariate analysis identified only smaller body surface area at the time of intervention as being significantly associated with moderate or severe PR in follow-up. Moderate or severe PR developed in 74% of patients who underwent the procedure as neonates, in contrast to 44% of all other patients. Using cardiac MRI to obtain RV volumes, Harrild et al. (46) found that 34% of patients at a median follow-up of 13 years after pulmonary valvuloplasty had a PR fraction >15%, while in 17% of the cohort, the PR fraction was >30%. In this series, PR fraction was associated with younger age at dilation and with BAR, particularly when the BAR was ≥1.4. The median PR fraction in patients with a BAR ≥1.4 was 26%. Other series have also found that a larger BAR and a higher degree of obstruction before dilation, in addition to younger age, are associated with the development of severe PR (32,34).
Compared with surgical valvotomy, patients treated with valvuloplasty appear to have less regurgitation with clinically equivalent relief of obstruction, although duration of follow-up is significantly longer for the surgical patients (31,47), and there is no contemporaneous surgical series. It is well known that significant degrees of PR with no other hemodynamically significant lesions are well tolerated for long periods. However, moderate degrees of surgically induced PR affect the normal hemodynamic response to exercise, resulting in decreased exercise capacity (48). Similarly, it has been found that patients with a PR fraction >15% following balloon dilation have decreased exercise capacity when compared to those with a PR fraction ≤15% (percent predicted peak VO2 85 ± 17% vs. 96 ± 16%) (46). It would seem prudent to accept mild degrees of residual stenosis as opposed to complete relief of obstruction in exchange for avoiding significant PR.
No patient outside of the neonatal group has been reported to have had pulmonary valve replacement following balloon dilation. In contrast, severe PR requiring surgery has been reported in a handful of patients who underwent balloon valvuloplasty as neonates (34,45). In a series of 107 patients, 6 had severe PR at a mean follow-up of 7.2 years (34). All were under 2 months of age at the time of valvuloplasty and had severe or critical obstruction. The postdilation gradient was significantly lower in these six patients (8 mm Hg) than in the whole group (19 mm Hg). One underwent pulmonary valve replacement, and the remaining five are likely to have surgery during childhood. The average BAR used in these six patients was 1.44, in comparison to 1.08 for the group as a whole. Perhaps even greater caution against overly aggressive dilation should be exercised in neonates than in their older counterparts, aiming for a BAR closer to 1.2, and not exceeding 1.3 (49). This approach may lead to repeat balloon valvuloplasty in a slightly larger number of patients with critical pulmonary stenosis, but this is preferable to the need for eventual pulmonary valve replacement.
Surgical Valvotomy
Since the advent of pulmonary balloon valvuloplasty, surgical valvotomy is reserved for patients with dysplastic pulmonary valves resistant to dilation or patients with multiple levels of fixed obstruction. Valvotomy can be achieved using either a closed or open technique through the main pulmonary artery. There is often a persistent pressure gradient immediately after surgery in patients with isolated valvar pulmonary stenosis attributable to dynamic narrowing of the hypertrophied infundibulum (as also observed following balloon valvuloplasty). A reduction in this gradient occurs in the first 24 hours after surgery, and continues at a slower rate as the hypertrophy resolves over the subsequent months. In rare cases, fatal right ventricular failure occurs (suicidal RV) in the immediate postoperative period. Propranolol may be given and may differentiate dynamic from fixed obstruction. When infundibular resection is necessary, it may be accomplished through a transatrial route via the tricuspid valve.
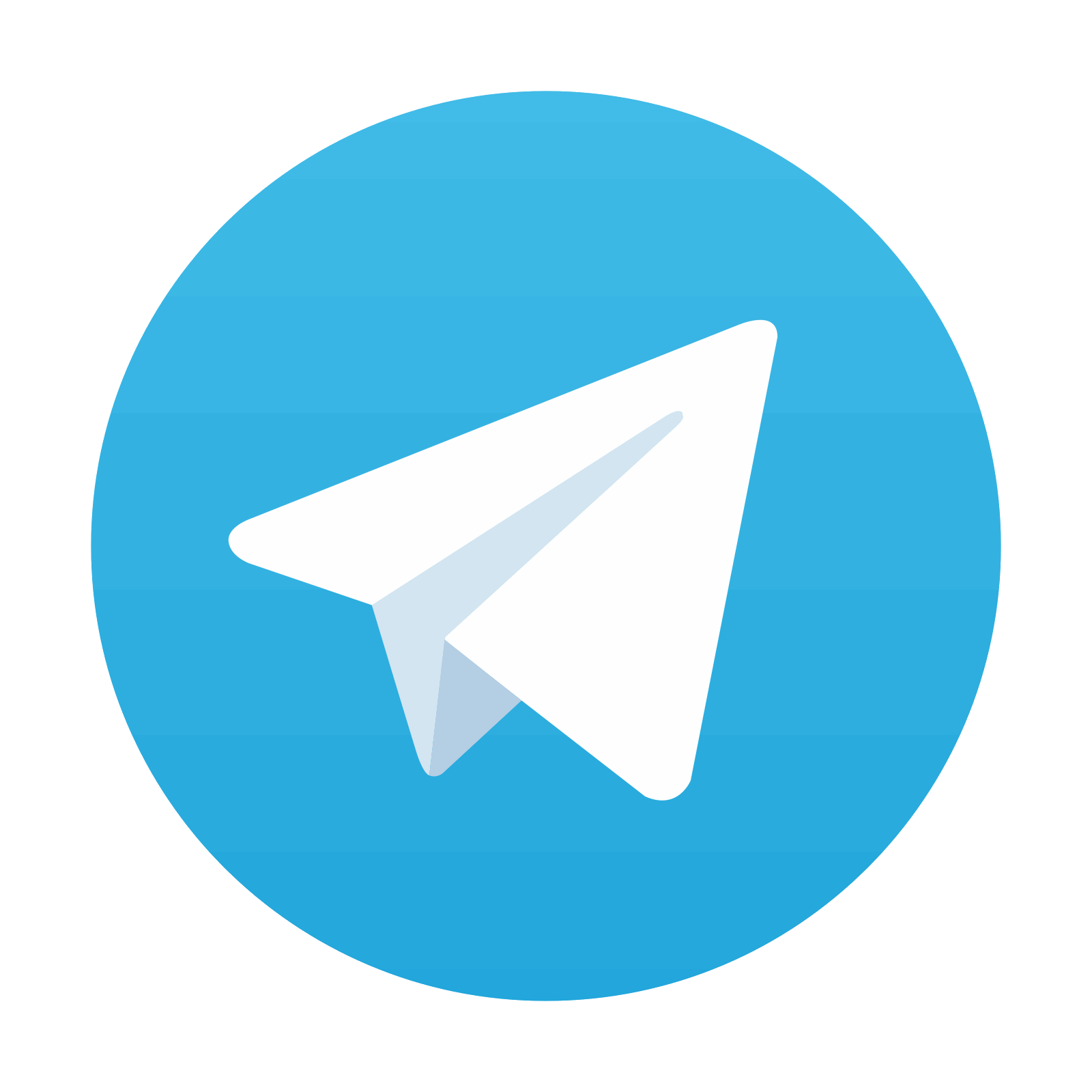
Stay updated, free articles. Join our Telegram channel

Full access? Get Clinical Tree
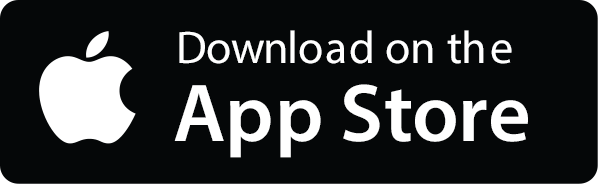
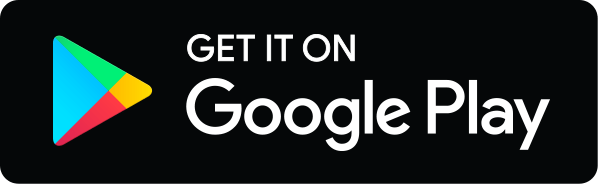
