Chapter 9 Pulmonary Function Testing
Testing in the pulmonary function laboratory allows further classification and quantification of lung disease by adding data from the measurement of lung volumes and assessment of gas exchange through measurement of diffusing capacity and arterial blood gases, and from tests of gas distribution. Special testing is available for prethoracotomy evaluation, assessment of upper airway obstruction, bronchoprovocation challenge testing, and cardiopulmonary exercise response (discussed in Chapter 10). Although even comprehensive lung function testing may not provide a specific diagnosis, the pattern of physiologic derangements guides further assessment, and demonstration of the severity of impairment aids in prognosis.
Spirometry
The Forced Expiratory Volume Maneuver
Assessments of vital capacity (VC), or forced vital capacity (FVC) and airflow are based on the forced expiratory volume maneuver, in which the subject inhales maximally to total lung capacity (TLC) and then exhales forcefully and completely to residual volume (RV). The expiratory flow rate at any point during this maneuver is determined by the driving pressure for airflow and the airway resistance. During a forceful exhalation, the intrathoracic pressure that surrounds the central airways exceeds the intraluminal pressure, causing dynamic compression of the airway (see Chapter 3, Figure 3-12). As a result, the effective driving pressure becomes the difference between alveolar pressure and the pleural pressure that compresses airways. This pressure difference (PA − Ppl) is equivalent to the elastic recoil pressure of the lung tissue. Thus, even during a forceful effort, the intrinsic elastic properties of the lung are a major determinant of airflow. Airway resistance upstream from the point of compression is determined primarily by airway caliber, which varies directly with lung volume. Throughout exhalation from TLC, both recoil pressure and airway caliber progressively decrease, so that airflow rates, after an early peak, also progressively decrease. Although the peak expiratory flow rate varies with the rapidity and forcefulness of the expiratory effort, once dynamic compression begins, the flow rate during the middle to later portions of the maneuver is limited and independent of further effort beyond the threshold needed to begin compression. These flows also are independent of added resistance downstream from the point of flow limitation. This physiologic arrangement aids in making the basic measurements of spirometry quite reproducible on repeated efforts.
To obtain a satisfactory spirometric tracing, the preceding inspiration must be maximal and the forced expiratory volume maneuver must be continued to cessation of flow or, when emptying is slowed, for at least 6 to10 seconds. The resultant information commonly is displayed in one of two formats. The traditional spirogram (Figure 9-1) plots volume versus time, with flow rate indicated by the steepness of the plot. The orientation of the axes varies with equipment, with time moving to the right and exhaled volume plotted either up or down. In the flow-volume display (Figure 9-2), the instantaneous flow rate is measured continuously and directly plotted on the vertical axis with volume on the horizontal axis. Time is not shown on this plot but may be indicated by tick marks. With this display, the reproducibility of successive efforts and some patterns of abnormality may be more easily seen. It is important to recognize that both the traditional spirogram and the expiratory flow-volume display are obtained from the same maneuver but emphasize different aspects of the information thus obtained.
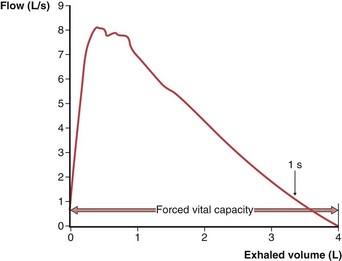
Figure 9-2 Normal expiratory flow-volume curve. The same forced expiratory volume maneuver shown in Figure 9-1 is plotted here as a flow-volume curve. The airflow rate reaches a peak early in the exhalation and then decreases progressively until flow ceases at residual volume.
(Modified from Culver BH: Pulmonary function testing. In Kelly WN, editor: Textbook of internal medicine, Philadelphia, 1988, JB Lippincott.)
Interpretation of Spirometric Abnormalities
Obstructive Impairment
A decrease in airflow is the hallmark of the obstructive diseases; this physiologic diagnosis rests primarily on the demonstration of an FEV1/FVC (or FEV1/VC) value below the age-appropriate LLN. When the FEV1/FVC value is low, even persons with an FEV1 itself as high as 100% of the predicted value (and, necessarily, with a high-normal FVC) are considered to have mild airflow obstruction, which has been shown to be associated with increased morbidity over time. Typically, FVC is normal early in the course of airflow obstruction but is reduced in more severe disease as the RV is increased because of trapped air. The severity of obstructive impairment is best quantified by the decrement in FEV1 as a percent of predicted, because progressive loss of FVC with advanced severity and air trapping limits the reduction in FEV1/FVC. Varying recommendations for categories of severity have been made by different groups, although an apparent consensus suggests that an FEV1 below 50% of the predicted value reflects a “severe” impairment. A simple schema that represents a compromise between one included in the ATS-ERS guidelines and that used in the Global Initiative for Chronic Obstructive Lung Disease (GOLD) guidelines is shown in Table 9-1.
Table 9-1 Suggested Categories of Ventilatory Impairment
Degree of Impairment | % Pred FEV1 |
---|---|
Mild |