Keywords
Fetal circulationArteriovenous fistulasArteriovenous malformationsCongenital heart diseasePre-tricuspid defectsAtrial septal defectVentricular septal defectPatent ductus arteriosusPulmonary hypertensionEisenmenger’s syndromeShunt reversalSelf-feeding flowsThe hemodynamic response to exercise is characterized by a combination of peripheral and central factors brought about by increased metabolic activity. Conversely, there are a number of conditions in which a hyperdynamic state exists in the absence of “metabolic cause”, for example, in congenital heart disease with communication between systemic and pulmonary circulations, in A-V malformations and in traumatic or acquired arteriovenous (AV) fistulas. Because of varied clinical presentation and a wide-ranging symptomatology, the “short circuit” between the arterial and venous circulations has been of interest to physiologists and clinicians alike for well over a century and offers a unique insight into the relationship between central and peripheral circulations. We will first consider the hemodynamic perturbations of AV fistulas.
19.1 Arteriovenous Fistula
A typical adaptive response to the opening of a “mature” (3 weeks post-procedure) femoral AV fistula in a dog is marked by a 5% drop in mean arterial pressure (MAP) and an increase in SV (18%), HR (24%), and CO (37%) [1]. There is a partial transfer of the circulating blood volume from arterial to venous side of the circulation with increase in CVP. Similar changes occur in dogs lacking spinal and autonomic innervation [2]. Creation of a more proximal fistula, i.e., by placement of a 10 mm diameter graft between infra-renal aorta and vena cava in dogs results in an immediate doubling of HR (97–173 beats/min) and CO (250%) and concomitant increase in SV and EDV [3]. Ventricular (load-independent) contractility index (Emax) was maintained in the immediate period of fistula opening but became significantly depressed during a week-long experiment. In spite of the compensatory hypertrophy (an average increase in heart mass of 10%), the animals experienced signs of severe congestive heart failure (ascites, limb edema, high LVEDP, and pulmonary edema) [3]. Fujisawa et al., on the other hand, noted an immediate increase in contractility (Emax) in dogs after opening of a large aorto-caval fistula [4].
When the initially large fistula flows are “within the limits” of cardiovascular compensation, a chronic condition ensues, characterized by a gradual return of HR and MAP to control levels, with concomitant increase in heart mass (hypertrophy) and expansion in extracellular fluid and blood volumes, resulting in progressive hemodilution. Huang and coworkers showed that in rats with large fistula flows (equaling about 50–77% of baseline CO), there was a progressive increase in CO over a 5 week period, reaching values over three times of control; however, when adjusted for increase in weight (due to increased blood volume), the cardiac index (CI) was the same as in the control group. The mean arterial pressure and systemic flow (determined by subtracting fistula flow from CO) initially decreased but gradually returned to normal. The persistence of near normal HR in the face of greatly increased CO indicated a large increase in SV [5]. This observation has been confirmed by several other reports in which increases in SV of up to 80–90% were observed in acute and chronic AV fistulas. With time, the circulation through the fistula continues to increase with further expansion of arterial and venous collaterals, leading to a vicious cycle of escalating cardiac outputs and CHF [6, 7]. In terms of cardiac biochemical and energetic changes, Gibbs and coworkers found only a small increment (13%) in basal metabolic activity and no change in mechanical efficiency in the hearts of rabbits subjected to chronic volume overload (12 weeks of aorto-caval fistula flows). This was in contrast to chronic pressure overload studies in which partial obstruction of the aorta resulted in a marked depression of myocardial metabolic activity, leading the authors to conclude that, “It seems clear from both our pressure- and volume-overload studies that in vivo cardiac failure can occur regardless of whether many of the intrinsic contractile and biochemical processes of cardiac cells are relatively unimpaired” [8].
Conventional explanation of circulatory effects caused by AV fistulae is invariably based on the concept of a drop in peripheral resistance. For example, Guyton and Sagawa initially maintained that LV hypertrophy is a response to increased workload on account of increased venous return and filling pressures [2], whereas in a later study by Guyton’s group the authors submitted that the cause of LV hypertrophy is not completely understood. However, the fact that blood flow to individual organs is eventually normalized, in the face of decreased peripheral resistance, suggests that flows are dictated by the metabolic demands of the tissues [5].

Surgically created forearm arteriovenous fistula of the type used in hemodialysis causes a drop in peripheral resistance and increase in cardiac output equal to fistula flow. Local adaptations to increased flow may include arterialization and flow reversal of the feeding veins and decreased arterial flow to the hand
High-output cardiac failure is a known complication of aorto-caval fistula in experimental animals [7] and in humans [11, 12] and is relatively common in renal patients on hemodialysis [13, 14]. Recent data moreover suggests that dialysis patients with high fistula flows develop pulmonary hypertension (PHT) [15, 16]. It is noteworthy that closure of the fistula in experimental animals [1] and end-stage renal disease (ESRD) patients, say, after receiving of a donor kidney, reverses the changes [17, 18]. Massive diuresis has been reported in a patient after closure of chronic aorto-caval fistula [19].
Several physiologists have remarked on a striking similarity between the acute circulatory response to exercise and the opening of a large AV fistula in the sense that a large decrease in peripheral resistance is met by increased CO (references quoted in [20]). However, as shown in Sect. 14.1, numerically derived “peripheral resistance” from Poiseuille’s equation [20] or Ohmic relationship [10] reflects neither global nor local state of organ perfusion. As mentioned, a surge in CO seen in aerobic exertion is a physiologic stimulus to increased metabolic demands that calls for a parallel rise in pulmonary blood flow and differs markedly from the increased pulmonary flows due to AV fistula. The overall increase in volume flow in AV fistula comes from two sources, namely, from the shunt and from the expanded blood volume due to maldistribution of systemic perfusion, with concomitant increase in plasma levels of rennin and atrial natriuretic peptide (ANP) [5]. The real difference between the two conditions, however, becomes apparent during chronic cardiovascular adaptation to increased fistula flows which invariably lead to increased pulmonary resistance and pulmonary hypertension.
19.2 Eisenmenger Syndrome
In addition to AV fistulas, other congenital cardiac abnormalities exist which lead to pulmonary vascular disease due to increase in pulmonary flows. Eisenmenger syndrome (ES), the most advanced form of pulmonary hypertension, is typically associated with a large communication between the pulmonary and systemic circulations at the atrial, ventricular, or aorto-pulmonary levels. The current interpretation of hemodynamics in ES goes back to 1950 when Paul Wood characterized the underlying pathophysiology of the condition in his classical paper as “pulmonary hypertension due to high pulmonary vascular resistance with reversed or bidirectional shunt” under the collective eponym of the Eisenmenger syndrome [21].

Distribution of cardiac output in fetus at selected checkpoints (percentage values in white). (Data from ref. [22], adapted from Motifolio Inc.)
Of note is the fact that a relatively hypoxic environment of the fetus is critical for the development of fetal lungs and pulmonary vasculogenesis. The near-term pulmonary vasculature actively responds to vasoactive agents and to changes in oxygen tension. Unlike the systemic vessels, which relax in response to hypoxia, the pulmonary vessels react with marked constriction. Hypoxic pulmonary vasoconstriction (HPV) is a physiological response by which the circulating blood is diverted away from hypoxic alveoli, in order to match perfusion with ventilation. The presence of low arterial PO2 in utero increases, while maternal hyperoxia decreases, fetal PVR. Increasing evidence suggests that the fetal pulmonary circulation is actively maintained in a vasoconstricted state by HPV (For review, see [23, 24]).

Oxygen saturation (SpO2 %) of fetal blood (values in green) and oxygen tensions (mmHg, values in black) at selected sites. Note that the fetus lives in a relatively hypoxic environment. (Data from ref. [22], adapted from Motifolio Inc.)

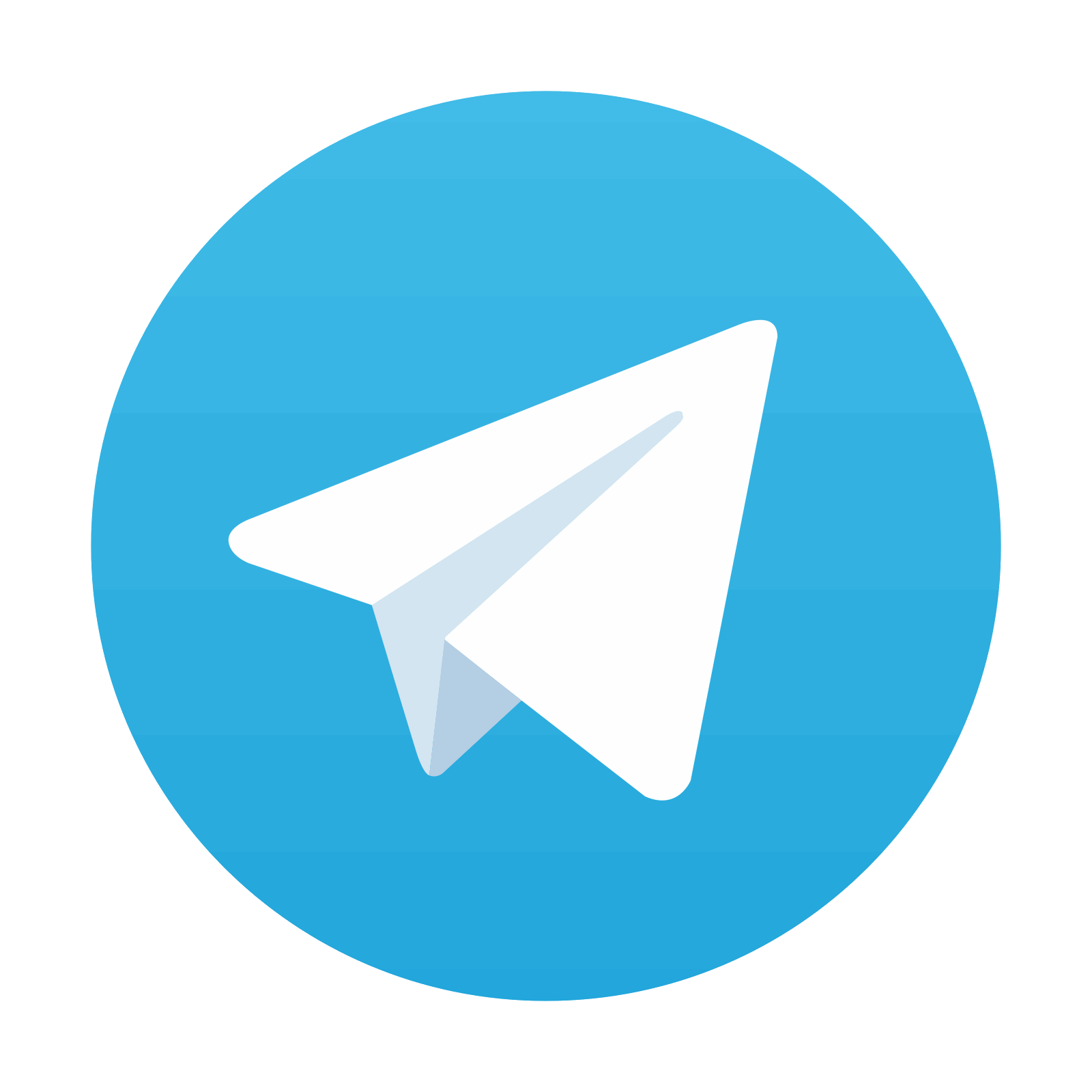
Stay updated, free articles. Join our Telegram channel

Full access? Get Clinical Tree
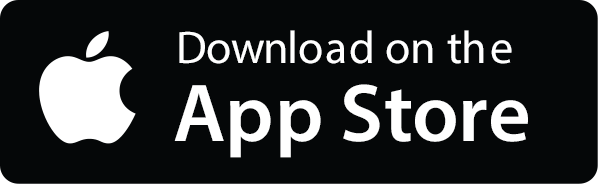
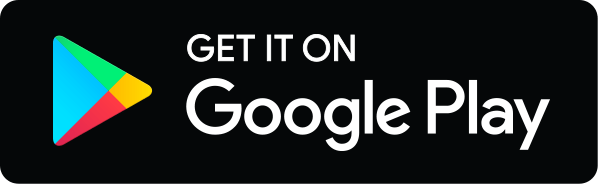