13
Pulmonary Edema
The lung becomes edematous when the extravascular water content exceeds 4 to 5 mL/g of dry blood-free tissue, as a result of either increasing the pressure across the pulmonary microvascular bed (high-pressure edema), damaging the capillaries so that they leak (increased permeability edema), or by some combination of these two mechanisms. Although the sequence of events in these situations is similar, important differences exist.1–3 For example, high-pressure edema can usually be reversed quickly by removing the factor responsible for increasing the pressure. However, edema that follows capillary injury tends to be more prolonged with a greater tendency toward organization of the exudates and progression to chronic lung disease.
The Pulmonary Vasculature
In general, the term microvascular is preferable to capillary when referring to the vessels involved in fluid exchange because pulmonary arterioles and venules as well as capillaries participate in this process.3 Arterioles that are less than 100 μm in external diameter are normally devoid of muscle and consist of a single elastic lamina lined by endothelium. Pulmonary venules of similar size have an identical structure and can be separated from arterioles only by their position in the lobule.4 The capillary density is somewhat variable between species, but the dog has ~80 capillaries per 100 μm of alveolar septum5 and controversy still exists as to whether they dilate or are recruited when pulmonary blood flow is increased.6 The pulmonary capillaries are located both in the flat part of the alveolar wall and in the corners where three alveoli meet (Fig. 13–1). The function of the capillaries at these different locations is interesting because those at the corners are thought to remain open, whereas the capillaries in the flat portion of the wall collapse as alveolar gas pressure rises above intravascular pressure.6
The endothelial cells that line the lung microvessels are joined by tight junctions.7 Normal fluid and solute exchange is now recognized to occur as both an active and passive process, but the “pores” that accommodate the passive movement of fluid are difficult to define anatomically because only 10 6 of 1% of the available endothelial surface area is required for this purpose.8
The Interstitial Space
Alveolar Wall Interstitial Space
The alveolar wall interstitial space lies between the basal lamina of the alveolar epithelial and capillary endothelial cells on the thick side of the alveolar wall (see Chapter 2).9 On the thin side the basal lamina of the alveolar lining cell and that of the capillary endothelial cells fuse, leaving a space estimated at 30 to 50 nm in width. On the thick side, the basement membrane of the endothelium and epithelium separate, leaving a space that is estimated at ~1 μm in diameter. This tiny space normally contains fluid, elastin and collagen fibrils, proteoglycans, fibroblasts, and macrophages, but does not contain lymphatic vessels. This means that fluid leaving the capillaries must traverse the alveolar wall interstitial space to gain access to the lymphatics in the extraalveolar interstitial compartment.
FIGURE 13–1 (A) Appearance of alveolar wall when the capillary bed is fully recruited. (B) Appearance of the alveolar wall when pressures rise and the capillary bed is derecruited. Note that the most prominent vessels appear at the corners where three alveoli meet. (C) High-power of corner vessel showing red blood cells, polymorphonuclear leukocytes, and platelets. (Courtesy of Dr. W. Hulbert.)
Extraalveolar Interstitial Space
The interstitial space of the alveolar wall is continuous with the extraalveolar interstitial space that surrounds the corner capillaries (Fig. 13–1) and communicates centrally with the space formed by the connective tissue that surrounds the pulmonary lobules. The lymphatic microvessels found in this space differ from venous capillaries in that they have valves that provide the capability of unidirectional movement of fluid. The lymph collected by these vessels is returned to the vascular compartment by both the right and left lymphatic ducts, which drain into systemic veins. The extraalveolar interstitial space increases in size as lung volume is increased,10 and under normal circumstances it is taken up by the expansion of the extraalveolar vessels. However, any increase in extravascular fluid tends to collect in this space first.2 As the external surfaces of the lymphatic capillaries are anchored to the connective tissue boundary of the interstitial space,11 they dilate as the space expands and provide much better drainage.
Fluid Movement Across the Microvasculature
Flow through the “pores” in the microvascular wall is governed by the resistance offered by the pore and by the pressure drop across the vessel wall.
When this resistance is expressed as its reciprocal or conductance K, the equation becomes
K is a complex value because it must account for both the surface area and the permeability of the pores. The pressure drop across the capillary wall is also complex in that it is made up of four separate forces originally delineated by Starling.12 These include the hydrostatic pressures in the microvascular lumen and in the interstitial space, and the oncotic pressures generated by the protein in the microvascular lumen and interstitial space.
The most important of these four pressures is the microvascular hydrostatic pressure that results from the action of the heart. When the heart stops, all of the pressures across the microvascular membrane equilibrate so that ΔP becomes 0. The nature of the interstitial hydrostatic pressure surrounding the pulmonary microvasculature is controversial. Staub3 argued that the pressure in the alveolar wall interstitial space is the same as alveolar pressure, whereas Guyton and associates13 argue that the surface pressure is balanced by the combination of a negative interstitial fluid pressure and a positive pressure at contact points between the solid elements in the interstitial space. They suggest that the surface pressure is the appropriate pressure to consider when determining the transmural pressure of the microvasculature, whereas the more negative liquid pressure is an important determinant of fluid exchange from the vessels to the interstitial space.
where M and Is refer to the microvascular and interstitial space, P and φ refer to hydrostatic and oncotic pressures, and σ is the reflection coefficient of the membrane.
Fluid Movement in the Alveolar Wall Interstitial Space
As there are no lymphatics in the alveolar wall, fluid that moves across the vascular membrane must drain to the extraalveolar interstitial space before it can be cleared by the lymphatics. The exact mechanism by which this occurs is unknown,3 but one probable explanation is that the compliance of the alveolar wall interstitial space is low, so that fluid entering it will increase the pressure and provide the force necessary to move the fluid from the alveolar wall to the more compliant extraalveolar interstitial space.
Fluid Movement in the Extraalveolar Interstitial Space
In nearly all forms of edema the excess fluid collects in the extraalveolar space first. It is then drained out of this space via the lymphatics, which empty it back into the venous system. The collection of fluid in the interstitial space lowers the resistance to flow through the lymphatics because they are tethered to the walls of interstitial space11 and dilate as the space expands. Complete filling of the interstitial space lowers the resistance of the lymphatics to the point where fluid entering this compartment appears to drain directly into the lymphatics.
Airspace Flooding
If the interstitial space is not cleared rapidly enough, the excess fluid eventually spills into the airspace. The exact site where it enters the airspace is not clear, in that some investigators think the fluid moves across the alveolar epithelium and others that it leaks near bronchoalveolar junctions.3 As it accumulates in the airspace, it first collects at alveolar corners, then spreads over the free surface, and then fills the alveolar space. The Laplace relationship governs the forces that hold the alveolus open and those that tend to close it:
where P = pressure, T = surface tension, and R = radius. As the alveoli flood, the surface tension increases as the surfactant is replaced by edema fluid and the radius of the alveoli is reduced. When the force generated by this arrangement exceeds transpulmonary pressure, the alveolar volume is reduced and they fill with fluid.
Safety Factors that Prevent Pulmonary Edema
Several safety features restrict the formation of pulmonary edema. For example, the fluid movement caused by a rise in microvascular hydrostatic pressure is balanced by the lowering of interstitial osmotic pressure that results from restriction of the protein in the fluid moving through the pores. Similarly, as fluid moves into the interstitial space, the hydrostatic pressure tends to rise and restore the difference between hydrostatic and interstitial space toward normal. Any increase in lung volume also tends to expand the interstitial space and increase its storage capacity. The lymphatics remove the excess fluid from the interstitium and drain it into the venous system; the alveolar epithelium has the capacity to actively absorb fluid that has leaked into the alveolar space.14–17 Indeed, the reabsorptive capacity may be critical to recovery from lung edema, and studies suggest that cellular disruption may interfere with this process in both normal-and high-pressure edema.18,19
Classification of Pulmonary Edema
Pulmonary edema can be classified based on the physiologic principles outlined elsewhere.20,21 Table 13–1 is a classification of lung edema based on the principles outlined above. It also indicates the types of edema that do not fit easily into either an increased pressure or a damaged membrane category.
Edema Due to Increased Microvascular Pressure
Cardiogenic pulmonary edema occurs as a result of a rise in left atrial pressure that causes pulmonary vascular hydrostatic pressure to increase. This occurs with myocardial failure from any cause, mitral and aortic valve disease, and rarely as a result of occlusion of the mitral valve orifice by an atrial myxoma. Pulmonary microvasculature pressure may also be increased by pulmonary veno-occlusive disease, fibrosing mediastinitis, and a variety of mediastinal masses, but these causes are relatively rare. A common cause of pulmonary edema in hospitalized patients with normal cardiac function is overexpansion of the vascular space by excessive intravenous therapy, particularly in patients with compromised renal function.
Edema Due to Lowered Interstitial Hydrostatic Pressure
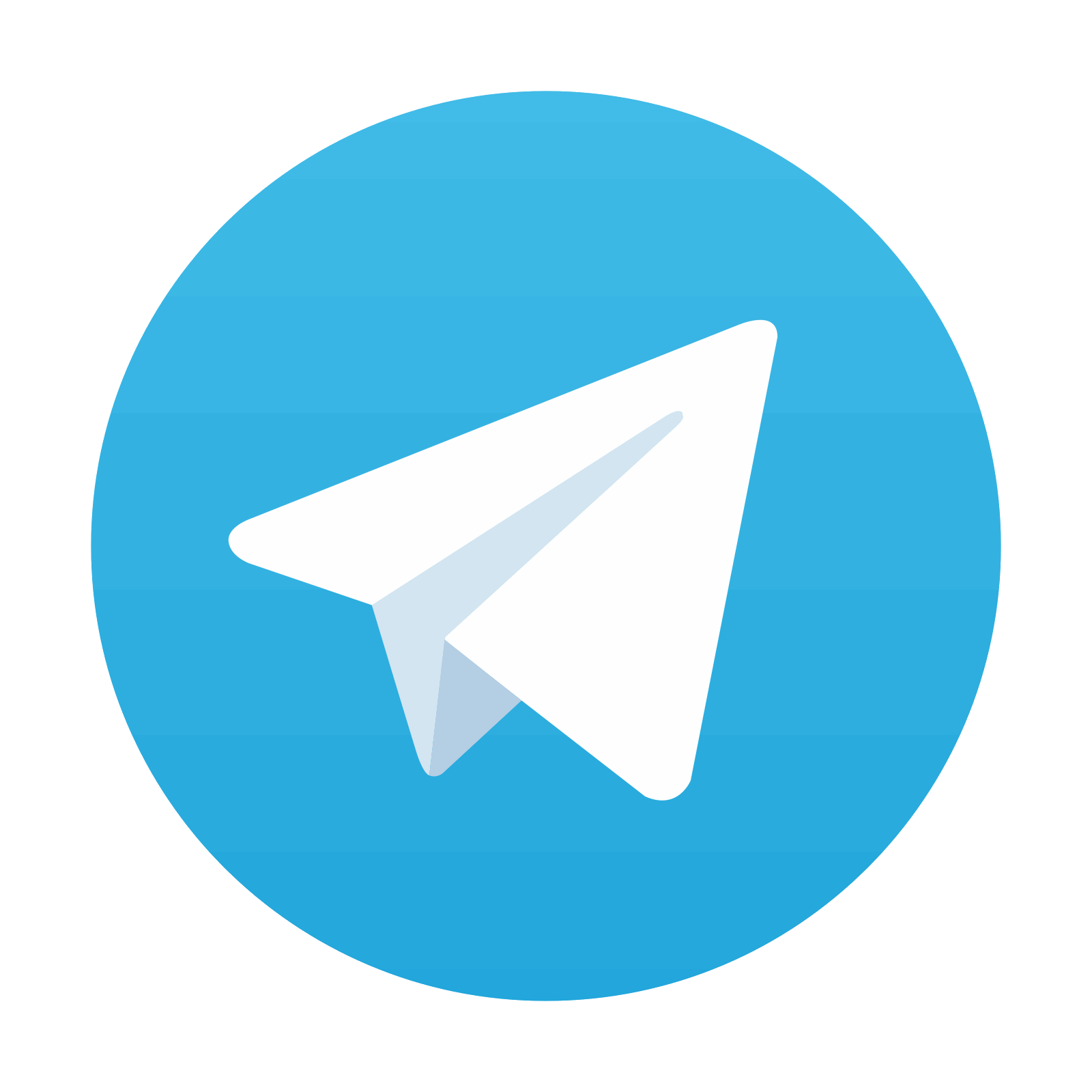
Stay updated, free articles. Join our Telegram channel

Full access? Get Clinical Tree
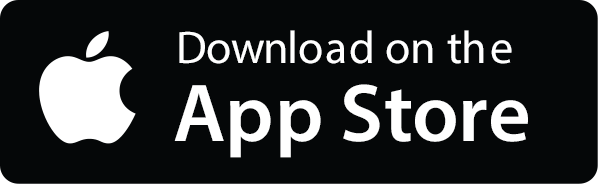
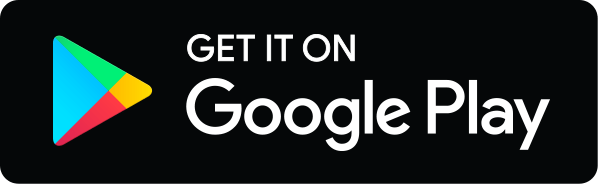