Abstract
Children with acquired immunodeficiency are at risk for many infectious and noninfectious pulmonary complications. This chapter will discuss such complications in children who have had solid organ transplantation, hematopoietic stem cell transplantation, and who have undergone treatment for childhood malignancy. Infectious and noninfectious complications will be discussed as well as an approach to the diagnosis of such respiratory complications in the child with an acquired immunodeficiency.
Keywords
pediatrics, lung disease, immunosuppressed, hematopoietic stem cell transplant, transplant
Introduction
Acquired immunodeficiency in children can be the result of a variety of diseases or their treatments. The majority of acquired immunodeficiency states, however, are the result of medical treatments that include pharmacologic, surgical, and radiotherapeutic regimens for the treatment of oncologic diagnoses. In addition, the expanding population of children receiving solid organ transplants is exposed to immunosuppressive medications to prevent graft rejection. Ablation chemotherapy combined with radiation used to prepare patients for hematopoeitic stem cell transplantation (HSCT) can produce direct toxicity to the lungs while rendering these patients temporarily pancytopenic and thus at risk for infectious complications. As a result, pulmonary disease in these patients is relatively common, and often results in significant morbidity and mortality.
This chapter will discuss pulmonary complications of solid organ transplantation, HSCT, and treatment of childhood oncologic disease. Included in these potential complications are pulmonary infections, and this will be the focus of the initial portion of this chapter. In addition, there are important noninfectious complications that will be addressed; some of these are specific to underlying conditions and their treatments.
Pulmonary complications of lung transplantation, human immunodeficiency virus (HIV)–associated acquired immunodeficiency syndrome (AIDS), and congenital immunodeficiencies are discussed in separate chapters ( Chapter 67 , Chapter 66 , Chapter 63 , respectively). Lung injury caused by pharmacologic agents, including chemotherapeutics, is discussed in Chapter 59 .
Pulmonary Infections in the Immunocompromised Pediatric Host
Immunocompromised pediatric patients include patients with congenital defects of innate host defenses including neutrophil abnormalities, defects affecting lymphocyte function, and defects of humoral immunity. The number of entities leading to such defects is rapidly expanding, with improved recognition of the nearly 300 clinical phenotypes associated with single-gene mutations affecting immune function. The major expansion of the population of immunocompromised children, however, has occurred with increased human stem cell and solid-organ transplantation, successful treatment of childhood malignancies, the AIDS epidemic, and the use of biologic agents such as inhibitors of tumor necrosis factor (TNF)-alpha for a variety of autoimmune diseases of childhood. In addition to increasing the at-risk population, these developments have also led to an increase in the number of identifiable pathogens. The term opportunistic pathogen is usually reserved for an organism typically infecting a patient with abnormal host defenses. The terms immunodeficiency and compromised host were first used in the 1960s and 1970s, for patients with primary immunodeficiency and for those patients who survived childhood malignancies. This portion of the chapter will review some of the more common pulmonary infections in this patient population.
Clinical Presentation of Pulmonary Infection in the Immunocompromised Child
The clinical presentations of pulmonary infection in the immunocompromised child are often nonspecific, and a high index of suspicion for atypical or opportunistic pathogens is required. Childhood cancer therapy, HSCT, solid organ transplantation, primary immunodeficiencies, and AIDS are each associated with specific pulmonary pathogens ( Table 64.1 ).
Bacterial | Fungal | Viral/Protozoal/Other | |
---|---|---|---|
NEUTROPENIA | |||
Chronic | Haemophilus influenzae, Streptococcus pneumoniae, Staphylococcus aureus, Klebsiella spp. | — | — |
Acute | S. aureus | — | — |
Immunosuppressive Therapy | S. aureus, Listeria spp., Mycobacterium tuberculosis | Aspergillus spp., Mucor spp., Histoplasma spp., Pneumocystis jirovecii | Cytomegalovirus (CMV), VZV, Toxoplasma spp., Herpes simplex virus, Cryptococcus spp. |
BONE MARROW TRANSPLANT | |||
Early (<30 days) | Pseudomonas spp., other gram-negative and gram-positive spp. | Candida spp. | — |
Late (>30 days) | S. aureus | Aspergillus spp., P. jirovecii | CMV, Toxoplasma spp., VZV, Epstein-Barr virus, adenovirus |
Late (>100 days) | Encapsulated Gram-positive (H. influenzae, S. pneumoniae) | — | VZV |
In addition, these patients often have an atypical or more severe course even when infected with a “usual” childhood respiratory pathogen. For example, viruses such as varicella-zoster, influenza, and measles are all capable of leading to devastating pulmonary infections in the immunocompromised host. Although adenovirus (AdV) can cause diffuse pneumonia in any child, it can be particularly common and catastrophic in immunocompromised hosts.
The clinical course of opportunistic infections is variable and affected by the type and degree of remaining host defenses. For example, fungal pulmonary infections in patients with chemotherapy-induced neutropenia and defective cell-mediated immunity are often associated with mild clinical symptoms and radiographic findings until the normalization of peripheral blood neutrophil counts leads to significant inflammation, lung destruction, pulmonary cavitation, and clinical deterioration. The interplay of fungal pathogens with the innate and adaptive immune systems is still under investigation. It is clear that fungi can both subvert and exploit the host immune response, allowing for chronic carriage or pathogenicity. This complex response is likely mediated by various molecular interactions involving cytokines as well as cellular elements.
Infectious Risks Shared by Malignancy, Solid Organ Transplantation, and Stem Cell Transplantation
The immune system has multiple components. Disorders of the immune system can be divided into those involving innate and adaptive components. There are physical barriers to infectious agents, including skin and airway epithelial cells. Humoral components of the immune system include immunoglobulins, complement, and other nonspecific antibacterial molecular species (e.g., defensins) important to innate pulmonary defenses. Cellular components include phagocytic cells, particularly neutrophils, and lymphocytes. A more complete description of the development, interactions, and defects of each of these components is presented in Chapters 8 and 63 .
Patients undergoing treatment for malignancy, HSCT, or solid organ transplant have periods of immunosuppression that vary in length and severity. The recovery of the immune system after myeloablative conditioning followed by HSCT, for example, can be divided into three phases. The preengraftment or early phase, from day 0 to day 30 or sooner, encompasses the time of marrow recovery leading to normalization of the peripheral neutrophil count; the postengraftment phase is considered day 30 to day 100 after HSCT; and the late phase follows day 100 post HSCT. For patients with HSCT, each phase is characterized by a susceptibility to certain types of infections correlating with the status of the immune system at that time point. Following solid organ transplantation, many patients initially receive intensive immunosuppressive therapy, leading to a more immunosuppressed state early in the posttransplant period. Patients with malignancies often receive chemotherapy in a cyclic pattern, leading to periods of severe marrow suppression followed by periods of recovery.
Fig. 64.1 demonstrates different phases of immunodeficiency in which certain infectious organisms may predominate. As occurs with individuals who are not immunosuppressed, respiratory viruses and community-acquired bacteria are quite common in the individual who is immunosuppressed. Those who are immunosuppressed, however, have a greater propensity for infection, with a wider variety of organisms (the opportunistic infections). A recent publication reviews radiological findings in children with acquired immunodeficiency states following cancer chemotherapy and is a useful reference for the evaluation of these patients.

Common Pulmonary Infectious Agents in the Immunocompromised Pediatric Host
Viral Pathogens (See Also Chapter 25 )
Cytomegalovirus
Cytomegalovirus (CMV) is a common herpesvirus infection of both neonates and older immunocompromised children. Viral carriage does not always lead to disease, however, and the progression from carriage to disease depends on many factors, including the age and the immune status of the infected individual. The organism can be acquired intrapartum or from breast milk, saliva, or blood (via infected white cells). CMV infection in the immunocompetent hosts rarely results in overt disease, and humoral and cellular immune mechanisms are important in limiting the infective capabilities of CMV. Such hosts that have latent CMV are seropositive, rendering them at-risk for viral reactivation in the event of immunocompromise. Individuals who are CMV-seronegative prior to either immunosuppression following transplantation or chemotherapy are at increased risk of CMV disease through CMV acquisition from de novo infection, from blood products containing virions, or from transplanted organs from CMV-seropositive individuals.
CMV-infected cells typically contain basophilic nuclear inclusions surrounded by a clear halo, giving an “owl eye” appearance; such inclusions are typically seen in alveolar cells. The pathology of CMV pneumonitis varies from diffuse, discrete parenchymal hemorrhagic nodules to diffuse alveolar damage, or chronic interstitial pneumonitis. The radiographic pattern of CMV pneumonia is usually a diffuse reticulonodular pattern that is less “alveolar” than is seen in pneumonia caused by Pneumocystis jirovecii (described later). High-resolution computed tomography (CT) of the chest most commonly reveals patchy or diffuse ground-glass attenuation, and more diffuse pulmonary involvement may be predictive of the progression of disease.
Prior to the availability of effective antiviral therapy, approximately 50% of patients with aplastic anemia or hematologic malignancy treated by allogeneic HSCT developed CMV infection and CMV pneumonia, with up to 90% mortality. CMV may also be a copathogen with other opportunistic organisms, including P. jirovecii, Epstein-Barr virus (EBV) and Aspergillus.
The diagnosis of CMV pneumonia is usually made by demonstration of typical inclusions in lung tissue. Urinary excretion of CMV can be coincident, but not necessarily causally related to disease. The most important methods for the detection of CMV in blood and potentially other fluids such as bronchoalveolar lavage (BAL) are those that estimate the quantity of virus or viral DNA. Of these, determination of CMV DNA using the polymerase chain reaction (PCR) is quite sensitive and widely available. Determination of the presence of the CMV protein pp65 within peripheral white blood cells is also useful to identify those patients harboring the organism. PCR for CMV detection in the blood has become a more commonly used noninvasive test to follow at-risk patients following HSCT, or solid organ transplant recipients, to more accurately predict the development of CMV disease. New standards for measuring the viral load of CMV in body fluids (including BAL) allows for more precision in following the course of the disease. PCR detection of CMV precedes culture isolation of CMV and persists longer than culture-positivity. Other techniques for identifying CMV in BAL include immunofluorescence, DNA hybridization, or microplate culture, combined with CMV antigen detection using monoclonal antibodies. All the techniques described, though highly sensitive, must be interpreted cautiously, since CMV can be a commensal and may be coincidentally present without being the cause of disease. Characteristic pathologic findings as well as a clinical scenario consistent with CMV disease are very important in deciding upon therapy.
The use of CMV-negative blood products has reduced the incidence of CMV pneumonia in seronegative transplant patients. Prophylactic and preemptive strategies for patients at risk utilizing ganciclovir, foscarnet, and high titer anti- CMV IgG have led to a substantial reduction in the incidence and mortality of CMV disease. Valganciclovir, the L-valyl ester and prodrug of ganciclovir, has improved oral bioavailability compared with ganciclovir, and is now the preferred drug for the prevention and treatment of CMV disease.
“Late” CMV has been observed in HSCT recipients with active graft-versus-host-disease (GVHD) who are receiving high doses of steroids (>1 mg/kg per day of prednisone), with low CD4 counts, and with history of prior CMV reactivation or extended use of anti-CMV treatment or prophylaxis. Reduced intensity conditioning regimens for HSCT, or so-called mini-transplants with fludarabine, single-dose radiation, and posttransplant cyclosporine and mycophenolate mofetil, is an emerging treatment for patients who are not candidates for standard myeloablative conditioning. These patients have shorter periods of neutropenia and lowered risk of CMV disease and viremia during the first 100 days, but may subsequently be faced with a delayed onset of CMV disease. Unlike early-onset disease, which is characterized mainly by interstitial pneumonitis, late CMV manifestations include retinitis, marrow failure, and encephalitis.
The interaction of CMV disease and allograft survival has been of particular interest to the lung transplant community, with reports of an increased incidence of bronchiolitis obliterans following CMV infection in lung allograft recipients. The precise mechanism leading to chronic lung allograft dysfunction (CLAD) in lung recipients remains unclear, although immunological mechanisms possibly targeting usually hidden potential antigens resulting in the production of auto-antibodies continue to be a target of investigation. The role played by CMV in the context of this immunologic dysregulation is still under investigation.
Respiratory Syncytial Virus and Other Common Respiratory Viruses
Respiratory syncytial virus (RSV) and other common respiratory viruses such as parainfluenza, influenza, bocavirus, and rhinovirus have long been recognized as important pathogens in adult recipients of HSCT as well as pediatric leukemia patients. Although usually causing upper respiratory tract infections, they commonly progress to involve the lower respiratory tract, carrying significant morbidity and mortality. In addition, RSV and other community-acquired respiratory viruses have been implicated in the subsequent development of CLAD in lung transplant recipients.
With community acquired respiratory viral infections, chest radiographs may have diffuse but nonspecific densities. Nasopharyngeal swabs or washings with cultures or enzyme immunoassays (EIA) are the most commonly used diagnostic tools for most viral pathogens, including RSV. Newer technologies, including reverse-transcriptase PCR (RT-PCR) and direct immunofluorescence assays, may prove to be specific, sensitive, and more rapid for detection, although further clinical studies will be required. Studies of the use of the antiviral agent ribavirin in treating adult recipients with RSV have suggested that early administration of aerosolized ribavirin, before significant lower respiratory disease develops, is superior to treatment with intravenous (IV) ribavirin. However, oral ribavirin, which is much less expensive than aerosolized or IV ribavirin, has been shown to be effective in the treatment of RSV. There is also support for the use of the monoclonal anti-RSV antibody known as palivizumab in patients who develop RSV following HSCT, although the largest study was in adults, and all patients also received inhaled ribavirin. While there is less published data concerning these pathogens in pediatric HSCT recipients, the increased likelihood of childhood exposure in schools and other environments should lead the clinician to consider such pathogens when evaluating an immunosuppressed child with upper or lower respiratory illness of uncertain etiology.
Varicella-Zoster Virus and Herpes Simplex Virus
Varicella-zoster virus (VZV) and herpes simples virus (HSV) are DNA viruses that typically cause infections of the skin and mucous membranes. However, in certain high-risk groups, including neonates, patients with cancer, AIDS, congenital defects of cell-mediated immunity, and recipients of solid organ transplants or HSCT, VZV and HSV can lead to visceral dissemination and pneumonia. Before the availability of specific antiviral therapy, VZV pneumonitis occurred in approximately 85% of cancer patients with visceral dissemination, with a resultant mortality of 85%. Pneumonitis is much less common with reactivation of herpes zoster and HSV, but it is a potentially serious infection following HSCT and in oncology patients.
The clinical presentation of VZV or HSV pneumonitis is nonspecific and includes fever, cough, dyspnea, and chest pain. Patients with VZV who have an increasing number of skin lesions, abdominal or back pain, or persistent fevers should be considered at high risk for dissemination. HSV pneumonitis may be more subtle in its presentation, and pneumonitis can occur in the absence of mucocutaneous lesions in newborns and HSCT recipients. Chest radiographs of herpesvirus pneumonias typically show ill-defined, bilateral, scattered nodular densities, first seen in the peripheral lung fields, with subsequent coalescence into more extensive infiltrates. Microscopically, the infection involves alveolar walls, blood vessels, and small bronchioles. Electron microscopy shows intranuclear viral inclusions. Hemorrhage, necrosis, and extensive alveolar edema are seen in severely affected areas of the lung; hemorrhagic tracheitis and bronchitis can also be seen. Secondary infections with bacterial pathogens were more commonly seen in the preantibiotic era, but with the emergence of resistant organisms such as methicillin-resistant Staphylococcus aureus, this remains a worrisome complication. Interstitial lung disease secondary to HSV infection has been reported following HSCT, but this is relatively rare and far less common than CMV-related interstitial lung disease in this population.
Varicella-zoster immune globulin can modify or prevent VZV infection in high-risk hosts exposed to the virus if given within 48–72 hours of exposure. VZV immune globulin, antiviral therapy with acyclovir and related drugs, and routine varicella vaccination have been effective in reducing the incidence of serious VZV pneumonias in immunocompromised hosts. However, a single varicella vaccination given prior to an acquired immunocompromised state may be insufficient to prevent disseminated VZV. In the case of HSV pneumonitis, acyclovir may not always be protective against respiratory complications. In vitro testing for antiviral sensitivity and the administration of alternative antiviral treatment may be warranted when proven HSV pneumonitis occurs.
Herpesvirus Type 6
Human herpesvirus 6 (HHV-6) is a DNA virus and is the etiologic agent of roseola. It can persist in normal hosts following infection and has been shown to integrate into the host genome in 0.2%–2% of the population. Immunosuppression can lead to reactivation of HHV-6, resulting in fever, hepatitis, bone marrow suppression, encephalitis, and interstitial pneumonia. HHV-6 may also be associated with coinfection by CMV and other pathogens. Antiviral compounds with activity against CMV, such as ganciclovir, foscarnet, and cidofovir, are also active against HHV-6, although increasing resistance to these agents by HHV-6 and other herpesviruses is becoming increasingly common.
Human Metapneumovirus
Human metapneumovirus (hMPV) accounts for a large proportion of cases previously relegated to “undiagnosed” respiratory infections, particularly in young children. Its epidemiology is similar to that of RSV —that is, winter epidemics—with variation in severity from year to year. Martino and colleagues recently described isolation of hMPV as a primary pathogen in 11/177 (6.2%) of nasopharyngeal aspirates from symptomatic adult HSCT recipients. An additional five patients had hMPV as a copathogen, one with Aspergillus, one with Aspergillus and CMV, and three with other respiratory viruses ( AdV, RSV, or influenza ). Fifty percent of the infections were considered nosocomial; pneumonia complicated hMPV -upper respiratory tract infections in four (44%) of nine and one (14%) of seven allo-HSCT and auto-HSCT recipients, respectively. More worrisome, Englund and coworkers reported real-time PCR evidence of metapneumovirus in 5 of 163 (3%) of BAL samples obtained in HSCT recipients experiencing respiratory disease. Of these five patients, four died of respiratory failure and most carried a diagnosis of “idiopathic pneumonia syndrome” (IPS; discussed further in this chapter). Evashuk and colleagues reported metapneumovirus infection leading to severe respiratory failure in an infant recovering from liver transplantation. More recently, an outbreak of hMPV affecting 15 immunocompromised adults with hematologic malignancies that led to respiratory failure in 33% and death in 26.6% was reported, highlighting the potential virulence of this particular pathogen. It is likely that further investigations will underscore the importance of hMPV as a viral pathogen in immunocompromised adults and children.
Adenovirus
AdV is a DNA virus that is a common cause of community-acquired lower respiratory tract disease. Serotypes 3 and 7 are associated with epidemics of bronchiolitis and pneumonia in the general population. In immunocompromised patients such as HSCT recipients, AdV infections can lead to gastrointestinal, urologic, and pulmonary morbidity with disseminated disease that is associated with a high mortality. AdV typically causes fever, pharyngitis, cough, and conjunctivitis. Pneumonia is usually mild in normal hosts, but rapid progression, with necrotizing bronchitis and bronchiolitis, can occur in immunocompromised patients. The radiographic picture is nonspecific and resembles other causes of diffuse pneumonia. Failure of pneumonia to respond to standard therapy, particularly in the setting of epidemic acute respiratory disease in the community or hospital staff, should raise suspicion of AdV involvement.
The diagnosis is usually made by lung biopsy or brushings demonstrating typical adenoviral inclusions, or by culture, but institution of empiric antibiotic and antifungal therapy leading to delay in invasive procedures may delay the diagnosis. PCR to quantify adenoviral DNA in blood or other fluids may be useful as a screening tool. The antiviral agent Cidofovir has been shown to have activity against AdV, but more study is needed before it can be universally recommended. Because very low T-cell counts in HSCT recipients are a major risk factor for disseminated disease in those patients found to harbor AdV, and because PCR is quite sensitive, algorithms have been proposed for determining which patients might benefit from antiviral treatment with agents such as cidofovir. Meanwhile, supportive therapy for affected patients includes oxygen, treatment of bacterial superinfections, IV immunoglobulin, and assisted ventilation. A novel approach to treatment of refractory AdV (and other viral) infections in immunocompromised patients involves the production of multivirus-specific T-cells using overlapping peptide libraries incorporating antigens from a variety of pathogenic viruses to stimulate peripheral blood mononuclear cells in vitro. While this technology is only recently developed, it may provide a more sustainable treatment in this patient population.
Fungal Pathogens (See Also Chapter 31 )
Pneumocystis jirovecii (Formerly P. carinii )
Pneumocystis has been an organism of uncertain taxonomy and was regarded as a parasite because of its resemblance to cystic spore-forming protozoa. DNA sequencing of 16S-like ribosomal RNA of P. carinii with phylogenetic analysis demonstrates that it is much more closely related to fungi than to protozoal organisms. There are several animal models for infection with Pneumocystis, with no evidence of infection transmission across species. The nomenclature P. carinii is now reserved for the organism infecting rats, while P. jirovecii is the organism isolated in humans. The term PCP, initially an acronym for P. carinii pneumonia, is retained with P. jirovecii pneumonia, now indicating Pneumocystis pneumonia.
The organism exists in two forms in tissues: the more common trophic, or trophozoite, and the cystic form containing sporozoites. The trophozoites measure 2–5 µm and stain best with Giemsa stains or fluorescein-conjugated monoclonal antibody but are not visible with Grocot-Gomori methenamine-silver nitrate or toluidine blue O stains, which stain the 5–8-µm cyst forms. The cysts are spherical or cup-shaped and often appear to contain up to eight 1–2 µm sporozoites within the cyst wall. The organism cannot be cultured from routine clinical specimens and must be identified in tissue, sputum, or alveolar lavage. The trophozoites appear to attach to type I cells through surface glycoproteins related to lectins and there undergo encystation. This interaction directly or through soluble factors leads to cell injury. The alveoli of lungs infected with P. jirovecii are filled with trophozoites and protein-rich debris, and the altered permeability produced by the organism contributes to the development of pulmonary edema and surfactant abnormalities, which lead to decreased pulmonary compliance and altered gas exchange. Latent infection with P. jirovecii was thought to be common, since serologic studies indicated that 40% of children had antibodies to the organism, and more recent studies using sensitive PCR assays have found evidence of P. jirovecii in the nasopharynx and lungs of normal infants. Colonization appears to be common in immunocompromised patients but less common in normal hosts, and there is some evidence of patient-to-patient transmission. Reactivation of latent infection in the immunocompromised host, previously felt to be the most likely explanation for disease, has been called into question by epidemiologic data, suggesting new acquisition of airborne organisms.
In patients with congenital or acquired immunodeficiency, the clinical features of PCP are nonspecific and include dyspnea, tachypnea, fever, and cough. Cyanosis occurs later, but early hypoxemia with a mild respiratory alkalosis is common. The most common chest radiographic finding is diffuse bilateral infiltrates.
P. jirovecii pneumonia can be treated with several medications, although trimethoprim-sulfamethoxazole (TMP-SMZ) is the preferred agent for both prophylaxis and treatment in children as well as adults. The earliest drug available for PCP was the dihydrofolate reductase inhibitor pentamidine, which was associated with a high rate of immediate adverse reactions including hypotension, tachycardia, and nausea as well as hypoglycemia and nephrotoxicity. Pentamidine may be administered by the aerosol route for treatment or prophylaxis in at-risk patients to prevent PCP, but its effectiveness is highly dependent on the delivery system used. Other “second-line” medications that have activity against P. jirovecii include combination therapy of clindamycin and primaquine, dapsone (sometimes combined with trimethoprim), and atovaquone. Limited studies and case reports of the use of the antifungal agent caspofungin have shown some promise, but further study is necessary. A newer form of combination therapy, reported for patients with AIDS, is trimetrexate-folinic acid, but this will also require further evaluation. For proven PCP infection with moderate to severe hypoxemia, high-dose TMP-SMX with adjuvant glucocorticoid therapy remains the treatment of choice.
Patients at known risk for P. jirovecii generally receive prophylaxis. For pediatric oncology and immunocompromised patients, oral TMP-SMZ given 3 days per week is effective. However, if patients or parents are noncompliant, there is a risk of breakthrough pneumonias on this schedule. For most patients, TMP-SMZ remains the drug of choice, but other prophylactic regimens have been used, including IV pentamidine.
Aspergillus Species
Aspergillus is a group of ubiquitous fungal organisms found in soil and other settings, including the hospital environment. Aspergillus fumigatus is the most common species to cause pneumonia in immunocompromised hosts, but other pathogenic species include A. niger and A. flavus. Invasive Aspergillus is most common in pediatric patients with malignancy (especially acute myelocytic leukemia) and HSCT.
In tissues, the organisms are seen as septate hyphae with regular 45-degree dichotomous branching, best seen with methenamine silver staining ( Fig. 64.2 ). Novel PCR-based detection methods for Aspergillus (and other fungal pathogens) in BAL samples are being developed but require further testing. Aspergillus causes both acute invasive pulmonary aspergillosis and a more chronic necrotizing form. The former occurs most commonly in patients undergoing cancer therapy, as well as other immunocompromised patients, such as those with aplastic anemia. Aspergillosis of the lung is often preceded by or accompanied by invasion of the nose and paranasal sinuses in susceptible hosts. Confirmed risk factors for aspergillosis include prolonged neutropenia, concurrent chemotherapy and steroid therapy, and broad-spectrum antibiotic therapy. Cutaneous aspergillosis is often observed in patients with disseminated disease. The killing and/or clearance of Aspergillus by neutrophils and alveolar macrophages are partly dependent on intracellular calcineurin, which itself is a target of widely used antirejection medications. This suggests another reason why transplant recipients are more susceptible to invasive Aspergillus infections.

In the lungs, Aspergillus can cause tracheobronchitis, pneumonia, abscesses, cavity formation, and diffuse interstitial pneumonia. The organisms often extend along blood vessels, and nodular lesions of necrosis surrounded by air often develop within an area of pneumonia, leading to the typical air crescent sign, seen on CT scan of the chest. This finding is more common in adults, rather than pediatric, immunocompromised patients, and while strongly suggestive of Aspergillus infection, is usually seen early in the disease process, and later it is replaced by nodular infiltrates with or without cavitation. CT scanning of the chest is much more sensitive than plain radiographs and can reveal early evidence of cavitation.
The diagnosis of Aspergillus pneumonia is generally made by tissue examination, but this has limitations, as several other fungal species can histologically mimic Aspergillus histologically. Aspergillus can be isolated from BAL in approximately 50% of cases, and needle aspiration biopsy of peripheral lung lesions can also demonstrate typical fungal lesions. Although direct tissue sampling by needle biopsy or transbronchial biopsy is recommended for diagnosis, the angioinvasive nature of Aspergillus increases the risk of bleeding or secondary infection following such procedures. Isolation of Aspergillus from a nasal culture in a patient with typical clinical risk features (prolonged neutropenia, progressive nodular infiltrates, cavitary lesion) may be helpful, but negative cultures do not exclude Aspergillus.
Galactomannan, a polysaccharide consisting of a mannose backbone linked to galactose side groups, is a component of the cell wall of Aspergillus that is released with growth. It can be detected in blood, BAL fluid, and cerebrospinal fluid in patients with invasive aspergillosis. Although most of the studies using galactomannan as a biomarker for invasive fungal disease have been in adult patients, it has gained favor in the pediatric population. Aspergillus galactomannan antigen detection in blood and BAL fluid as well as other noninvasive tests such as fungal-specific PCR-based diagnostics under development offer the promise for accurate diagnosis without more invasive procedures such as needle biopsy or transthoracic lung biopsy. Further studies to demonstrate utility in clinical practice will be necessary.
Although initially amphotericin B was the sole antifungal agent with activity against Aspergillus, newer agents are available and include the orally available itraconazole and voriconazole, which have excellent activity against Aspergillus; of these, voriconazole is the treatment of choice for invasive aspergillosis. Alternative therapies for aspergillosis include lipid formulations of amphotericin B, caspofungin or other echinocandins, or itraconazole. The utility of surgical excision of Aspergillus lesions is somewhat controversial. Invasive pulmonary aspergillosis during treatment of hematologic malignancy is generally considered a contraindication to subsequent BMT, but adult patients treated with amphotericin B and surgery have survived free of disease and without reactivation of Aspergillus following BMT. The outcome of Aspergillus pneumonia depends primarily on host factors, including degree of immunosuppression and recovery of neutrophil counts, as well as early diagnosis and treatment.
Mucor and Rhizopus
Mucormycosis includes fungal disease caused by organisms in the order Mucorales, including a variety of species in the genera Rhizopus, Mucor, and Cunninghamella. In tissues they are differentiated from Aspergillus by their broad, nonseptate hyphae that branch at angles up to 90 degrees and have an appearance of “twisted ribbons.” Rhizopus organisms cause disease only in patients who are immunosuppressed or who have an underlying disease, especially diabetes. In adults, it is associated with chronic or recurrent acidosis, such as uncontrolled diabetes mellitus with ketoacidosis. Most pediatric cases of pneumonia occur in the oncology population, where the organism affects the same risk groups as Aspergillus.
Pneumonia due to Rhizopus is usually an insidious segmental pneumonia that is slowly progressive despite antifungal therapy. Persistent fever, chest pain, hemoptysis, and weight loss are typical. Cavitation may occur, and dissemination to brain and other sites occurs because of the propensity of the organism to invade blood vessels. Death may occur suddenly with massive pulmonary hemorrhage, mediastinitis, or airway obstruction. The specific diagnosis usually depends on demonstration of the organism in specimens obtained by open, transbronchial, or needle-aspiration biopsy. As with Aspergillus, treatment with amphotericin B and possibly surgical resection as early as possible is critical to achieving a cure. Posaconazole has good activity against mucormycosis, which can be aggressive and relatively resistant to voriconazole. Correction of chronic acidosis, if present, also appears to be important in some forms of Rhizopus disease.
Candida Species
Though important as a cause of fungal sepsis and secondary hematogenous pulmonary involvement, primary Candida pneumonia is unusual. Candida albicans and C. tropicalis are the most important causes of fungal sepsis and secondary pulmonary involvement. Neutropenic children colonized with C. tropicalis are at a 10-fold higher risk for dissemination than children colonized with C. albicans. Patients with HIV infection, primary immunodeficiencies, or prolonged neutropenia are at greatest risk, but other predisposing conditions include diabetes, corticosteroid administration, broad-spectrum antibiotic treatment, IV hyperalimentation, and the presence of deep venous access devices (conditions common to the patient with solid organ or HSCT).
In tissue, silver stains show oval budding yeasts 2–6 µm in diameter with pseudohyphae. The prominent histologic features of primary Candida pneumonia include bronchopneumonia, intraalveolar exudates, and hemorrhage. Amphotericin B is usually the treatment of choice for invasive Candida infections, along with flucytosine if synergism is desired. The imidazole antifungal agents, including ketoconazole, fluconazole, and itraconazole, have activity against C. albicans and have been used successfully. Although fluconazole prophylaxis, as well as the use of hematopoietic growth factors, has led to a reduction in the frequency of early Candida infections in patients at risk, many institutions have experienced an increase in azole-resistant nonalbicans Candida infections. In response to this, caspofungin appears to be an excellent alternative, with less toxicity than amphotericin B and improved coverage against systemic Candida infections when compared with fluconazole. A Cochrane review of various antifungal treatments points out the relative benefits of liposomal Amphotericin as well as the need for further controlled clinical trials to determine the optimal treatment for deep fungal infections in the pediatric host. There is increasing interest in the use of combination therapies to improve the treatment of Candida, as well as other fungal pathogens. Such combination therapies take advantage of different therapeutic targets of the various antifungals, possibly reducing the emergence of resistant organisms.
Histoplasmosis and Blastomycosis
Histoplasma capsulatum and Blastomyces dermatitidis are ubiquitous soil fungi endemic to the eastern and southeastern United States. Histoplasmosis is especially associated with exposure to bird or bat fecal material ; as a result, it is a fairly common infection in immunocompetent children and may be asymptomatic or lead to an acute pneumonia with fever, hilar adenopathy, and pulmonary infiltrates. Blastomycosis is a less common but more serious infection. Both can cause chronic granulomatous pulmonary disease and lead to extrapulmonary dissemination.
In the immunocompromised patient, particularly pediatric oncology patients, histoplasmosis may present as an acute illness with fever, cough, and diarrhea, or in a disseminated form with additional features of hepatosplenomegaly, fevers, and adenopathy. Progressive disseminated histoplasmosis is a rare (<0.1% of cases) complication of primary infection, but it is much more common in immunocompromised patients. Interestingly, neutropenia is not always associated with H. capsulatum infections. Chest radiographs or CT scans usually demonstrate hilar adenopathy and nodular parenchymal disease in both forms of the disease. Blastomycosis is much less common in adult or pediatric oncology patients, and may be associated with dermatologic manifestations such as skin ulcers, in addition to diffuse chronic pulmonary infiltrates.
Amphotericin B is indicated for both histoplasmosis and blastomycosis in immunocompromised hosts. Itraconazole is also effective for histoplasmosis and moderate blastomycosis without central nervous system involvement.
Cryptococcus Neoformans
Cryptococcus neoformans is a yeast that causes protean clinical manifestations in immunocompromised patients, often involving the meninges, endocardium, skin, and lymph nodes. The lungs are the portal of entry for C. neoformans, and pulmonary involvement may be minimal if dissemination occurs quickly. Pneumonia typically causes chest pain, fever, and cough. The diagnosis of cryptococcosis relies on demonstration of the organism histologically, in biopsy tissue or pleural fluid, or by culture methods. Although controlled trials are lacking, treatment of cryptococcosis in immunosuppressed patients with IV amphotericin B and oral flucytosine followed by fluconazole is recommended.
Rarer Fungal Pneumonias
Several recent trends have been noted in fungal infections, among them increased identification of rarer fungal pathogens, including saprophytic fungi such as Trichosporon beigelii and Fusarium sp. These fungi cause skin and soft tissue infections and occasionally invade the lungs and sinuses. For example, Scedosporium apiospermum (formerly Pseudallescheria boydii ) causes invasive disease in solid-organ transplant patients, with lung involvement in 50% ; it is difficult to distinguish histologically from Aspergillus. These fungi are thus often difficult to diagnose, and unlike Aspergillus, their response to therapy with amphotericin B and echinocandins (e.g., Caspofungin) may be very poor. Newer azole agents such as posaconazole and voriconazole have activity against Fusarium spp. and Scedosporium spp. and should be considered if these species are isolated from immunosuppressed patients. Other rare fungal pathogens seen in immunocompromised patients include filamentous fungi of which there are several species, including Dactylaria gallopava, Cladophialophora bantiana, and Exophiala. Several of these have a proclivity for infecting the CNS, but soft tissues and lungs may also become involved with cavitary lesions.
Bacterial Pathogens (See Also Chapter 25 )
The bacterial pathogens associated with pneumonia in immunocompromised hosts include those pathogens typically associated with pneumonia in children, such as S. aureus (particularly methicillin-resistant strains), H. influenzae, and S. pneumoniae. Pseudomonas aeruginosa is an additional important cause of pneumonia and sepsis in hospitalized immunocompromised children. Significant risk factors for bacterial infections include neutropenia, the presence of indwelling venous catheters, and perineal skin lesions. More unusual bacterial causes of pneumonia include Listeria monocytogenes, a gram-positive rod that causes primarily septicemia in immunocompromised patients, with subsequent pulmonary involvement. Corynebacteria (commonly called diphtheroids) are gram-positive bacilli or coccobacilli that exist as saprophytes on mucous membranes and skin. Corynebacterium jeikeium is a strain from this group that may lead to sepsis and pneumonia in oncology and HSCT patients. Listeria can be successfully treated with ampicillin plus an aminoglycoside, but newer cephalosporins are not active against Listeria. C. jeikeium is resistant to most antibiotics except vancomycin. Other rare Gram-negative organisms that cause pneumonia in the immunocompromised host include Legionella pneumophila and Capnocytophaga sp. It is important to realize that factors such as geographic location, changes in infection control, prophylactic antimicrobial protocols, and technological advances all have an effect on microbial predominance.
Mycobacteria (See Also Chapters 29 and 30 )
With the onset of the AIDS epidemic, disease due to both Mycobacterium tuberculosis and atypical strains such as M. avium-intracellulare, M. kansasii, and M. abscessus have been increasingly recognized in both AIDS and non-AIDS immunodeficient populations.
The development of disseminated M. tuberculosis following HSCT is a serious and often fatal complication ( Fig. 64.3 ). Based on data from developed countries, M. tuberculosis infections are rare in HSCT recipients. However, the incidence of tuberculosis in the HSCT population directly reflects its incidence in the general population. In Turkey, where tuberculosis is endemic (35/100,000 population vs. 7/100,000 in the United States), tuberculosis was 40 times more common in allo-HSCT patients than in the general population. The presence of multidrug-resistant strains of M. tuberculosis (indirectly related to treatment of HIV disease) is of great concern and has led some programs to maintain a high index of suspicion for tuberculosis and to treat HSCT recipients with this complication for longer periods of time, often with multidrug regimens.

Nontuberculous mycobacterial (NTM) infections can be either catheter-related or respiratory infections. Mere isolation of NTM on BAL may not be of pathogenic significance, unless there is evidence of tissue invasion or concomitant bacteremia is present. Treatment requires 2–4 antimicrobials guided by in vitro susceptibility testing, and removal of indwelling catheters (if contaminated), as well as surgical debridement of subcutaneous tunnel infection sites.
Legionella Pneumophila
Patients undergoing bone marrow and solid organ transplantation are particularly susceptible to Legionella infections due to prolonged neutropenia and abnormalities in cell-mediated immunity. Legionnaires’ disease (LD) can be acquired by inhalation of aerosols containing L. pneumophila, or by microaspiration of contaminated drinking water. LD should always be in the differential diagnosis of pneumonia among HSCT recipients. Appropriate tests to confirm LD include culturing sputum, BAL, and tissue specimens; testing BAL specimens for Legionellae by direct fluorescent antibody (DFA), as well as examining for L. pneumophila serogroup 1 antigen in urine and performing testing for 5S rRNA PCR of BAL, urine, or serum samples.
Parasitic Agents (See Also Chapter 33 )
Toxoplasma Gondii and Cryptosporidium Parvum
Toxoplasma gondii infects cats and other animals and secondarily infects humans, causing congenital toxoplasmosis during intrauterine infection. Primary infection later in life usually causes only lymphadenopathy and mild systemic symptoms. Cryptosporidium parvum infects a variety of hosts, including immunosuppressed individuals. Its usual routes of transmission are water- or food-borne, but person-to-person transmission is possible. Toxoplasma primarily causes central nervous system disease, but disseminated disease with secondary pulmonary involvement can occur, presenting with shortness of breath, cough, fever, and bilateral interstitial infiltrates. C. parvum causes severe diarrhea, but disseminated disease with pulmonary involvement can occur. Treatment of T. gondii is with pyrimethamine-sulfadiazine, while treatment of C. parvum is rapidly evolving, with combination therapy of azithromycin-paramomycin being supplanted by the more effective nitazoxanide in many parts of the developing world.
Pulmonary Coinfections
Late after solid organ transplantation or in the late phase infections following HSCT, it is common to find copathogens—that is, isolation of more than one pathogenic species of bacteria, fungus, or opportunistic virus from BAL or lung biopsy specimens. For example, pulmonary copathogens may be isolated in as many as 53% of patients with parainfluenza pneumonia. HSCT recipients with CMV disease or respiratory viral infections are more susceptible to invasive fungal infections, especially Aspergillus. Alangaden and colleagues described the occurrence of gram-negative bacilli and Aspergillus infections among allogeneic BMT recipients with chronic GVHD requiring long-term steroid use. Chronic colonization of the airways of patients with GVHD may be analogous to colonization of the respiratory tract, with Pseudomonas and Aspergillus species in patients with cystic fibrosis, possibly suggesting an alteration in the homeostasis of the respiratory mucosa, mucociliary clearance, and airway surface liquid. Recovery of bacteria from respiratory secretions in this scenario may represent airway colonization rather than invasive parenchymal disease.
Pulmonary Complications Following Solid-Organ Transplantation
This section will refer mainly to pulmonary complications of nonlung, solid-solid organ transplantation, as lung transplantation and its complications will be discussed in Chapter 67 .
Pulmonary Edema, Pleural Effusions, and Acute Respiratory Distress Syndrome
Pulmonary edema can occur posttransplant because of increased hydrostatic pressure (e.g., caused by fluid and blood product administration or poor left ventricular function) or decreased oncotic pressure (e.g., caused by hypoalbuminemia), or because of increased vascular permeability (e.g., caused by immune-mediated transfusion-related acute lung injury [TRALI]).
Pleural effusions may also occur as a result of increased hydrostatic or decreased oncotic pressure. Lymphatic vessels that drain the lungs can be overwhelmed or disrupted in transplantation. Infection or injury in the thoracic, mediastinal, or abdominal cavity can lead to sympathetic effusions or empyema. Finally, abdominal ascites fluid (i.e., in patients with liver or kidney disease) can translocate through pores in the diaphragm and enter the pleural space.
Pleural effusions can occur in up to 40% of children who undergo liver transplantation, are predominantly right-sided, and are thought to occur as a result of disruption of diaphragmatic lymphatic channels. Effusions exclusively in the left pleural space following liver transplantation should prompt evaluation for other causes. Generally, pleural effusions following liver transplantation will enlarge over the first week following transplantation and then resolve over the ensuing 3–4 weeks. Between 14% and 25% of pediatric patients require pleural drainage because of significant respiratory compromise. Not surprisingly, pleural effusions significantly increase the duration of mechanical ventilation and ICU days. Persistent pleural effusions may be a sign that acute rejection of the liver allograft is occurring.
Acute respiratory distress syndrome (ARDS) is a devastating complication in up to 15% of liver transplant recipients in the immediate postoperative period. This is primarily caused by sepsis, but in the case of liver and small bowel transplantation, other risk factors should be considered as potential causes (e.g., severe malnutrition of the recipient, the extensive and prolonged abdominal surgery, massive intraoperative blood transfusion, and aspiration in the early posttransplant period).
Impairment of Respiratory Mechanics
The extensive surgery in the upper abdomen that occurs during orthotopic liver transplantation can have significant effects upon diaphragmatic excursion, especially on the right side. Diaphragmatic dysfunction can also occur in heart, kidney, and small bowel transplantation, but to a lesser degree than after liver transplantation. Poor excursion of the diaphragm can lead to impaired cough and airway clearance, resulting in atelectasis and pneumonia. Diaphragmatic function can be impaired by swelling in the subdiaphragmatic area, as well as phrenic nerve injury when the suprahepatic vena cava is clamped during liver transplantation. Diaphragmatic dysfunction or paralysis can be found in 8%–11% of pediatric liver transplant recipients, and with more sensitive techniques, phrenic nerve conduction abnormalities are seen in up to 80% of adult liver transplant recipients. For adults, the injury to the right phrenic nerve does not seem to have significant impact on recovery, as determined by the duration of mechanical ventilation or hospital stay. In infants and small children, compromise of one diaphragm can lead to more respiratory distress, atelectasis, and pneumonia, as well as longer duration of mechanical ventilation and ICU stays.
Recruiting atelectatic lung and performing adequate airway clearance in the postliver transplant period can be challenging because of pain and the “fresh” surgical sites. This is especially true for patients in whom the abdomen is not completely closed in the immediate postoperative period. In this situation, the important abdominal muscles that are needed for cough are impaired. Patients may require noninvasive positive pressure ventilation (NIPPV) after extubation. Airway clearance and the use of a mechanical in-exsufflator (cough assist device) can help clear secretions and prevent atelectasis in the immediate postoperative period. Since physiotherapy to the chest wall may be painful after sternotomy, oscillating positive expiratory pressure devices and incentive spirometry (in patients who can cooperate) and intrapulmonary percussive ventilation (in those that cannot cooperate) can be invaluable in the postoperative period. When diaphragmatic dysfunction or paralysis is present, plication of the diaphragm can be considered (utilized in up to 25% of patients with diaphragm paralysis). However, plication should only be considered if phrenic nerve function has not returned after a period of observation with noninvasive management of at least 90 days, or if the patient develops clinical respiratory deterioration.
Medication Toxicity
Early infusion of cyclosporine has been reported to cause ARDS in patients receiving liver transplantation. Fortunately, most medications used for immunosuppression in the postorgan transplantation period do not have direct toxicity to the lung. Two exceptions are the mammalian targets of rapamycin (mTOR) inhibitors, sirolimus (rapamycin) and everolimus. Both are potent immunosuppressants that confer less nephrotoxicity than calcineurin inhibitors. They have been increasingly popular because of their overall favorable side-effect profile. However, sirolimus and everolimus cause interstitial pneumonitis at both therapeutic and supratherapeutic drug levels. Incidence of this complication ranges from 0.4% to 2.9%. Sirolimus-induced interstitial pneumonitis can occur acutely, but the onset is usually insidious. Patients usually present with dyspnea on exertion (66%–90%), dry cough (75%–100%), and fever (60%–87%). Lung function testing may reveal a restrictive defect. Plain films and CT scans of the chest might reveal an interstitial pattern with interstitial infiltrates, consolidation, or ground-glass opacification with lower lobe predominance. BAL and biopsy specimens can show patterns of lymphocytic alveolitis, pulmonary hemorrhage, or organizing pneumonia. Treatment requires discontinuation of the causative medication, and resolution is generally prompt, although radiographic abnormalities may persist. Corticosteroid use should be considered and may be effective, although supportive data are limited.
Posttransplant Lymphoproliferative Disease
The immunosuppression required for solid-organ transplant recipients places them at increased risk for developing posttransplant lymphoproliferative disease (PTLD). PTLD typically stems from an immunosuppressive regimen that causes T lymphocyte depletion, which then leads to uncontrolled EBV-driven B cell proliferation. This unregulated growth of B cells can range from benign polyclonal B lymphocyte expansion to aggressive immunoblastic B cell lymphomas. Less frequently, PTLD also can result from T cell or natural killer (NK) cell proliferation. PTLD occurs in approximately 3%–9% of heart transplant recipients and up to 20% of intestinal transplant recipients. PTLD can occur following kidney and liver transplantation as well, but less frequently. PTLD arising in the lung is much more common following heart, lung, and heart-lung transplantation than following transplantation of abdominal organs. The median onset of PTLD is typically within 24 months following solid-organ transplantation.
A significant risk factor for PTLD is seroconversion of an EBV-naïve recipient, either by primary infection in a previously uninfected recipient or by transplantation of a graft from an EBV-seropositive donor. Other risk factors include Rh factor negativity, Rh mismatch, and recipient CMV seronegativity, and a high degree of immunosuppression, although induction immunosuppression does not appear to appreciably increase the risk of PTLD. Shortness of breath, cough, and upper airway obstruction were the most common symptoms in those with pulmonary PTLD. Because many will not have symptoms at presentation, screening of the at-risk patient population with EBV viral load may be the earliest means of detecting the presence of EBV as a marker for PTLD. A retrospective study in liver transplant recipients showed a marked reduction in PTLD and prevention of rejection in patients in the era in which EBV PCR monitoring was available (PTLD incidence decreased from 14.9% to 1.9%). Increasing EBV load may signify a need to adjust the patient’s immunosuppressive regimen. Because CMV may be a coinfecting agent in these patients, prophylactic antiviral therapy and CMV PCR monitoring are also recommended. In patients who present with pulmonary disease, nodular abnormalities are the most common finding ( Fig. 64.4A and B ), although lymphadenopathy, consolidations, or effusions may be seen on chest imaging. Even when PTLD is strongly suggested by the combination of increasing EBV load and typical radiographic findings, biopsy material should be obtained to confirm the diagnosis histologically (see Fig. 64.4C ) and assess for the presence or absence of EBV-early RNA (EBER)–staining cells (see Fig. 64.4D ). In addition, it is useful to characterize cell surface markers such as CD20, which may guide therapy.

Initial therapy for PTLD is the reduction of immunosuppression to reduce the degree of T lymphocyte depletion. This reduction can be associated with further complications (e.g., graft rejection) and still may be insufficient for recovery. Thus some have employed novel assays of immune function to monitor the degree of immunosuppression, while at the same time monitoring EBV viral load. Antiviral agents and IV immunoglobulin, including anti–B cell immunotherapy with the monoclonal anti-CD-20 antibody (rituximab), have been attempted with varying degrees of success. The main complication of rituximab therapy is prolonged B cell depletion and hypoimmunoglobulinemia. Anti-CMV therapy should be continued in patients at risk for this coinfection, but it may not decrease the cellular proliferation of PTLD, because there is very little active EBV replication in these lesions.
PTLD following solid-organ transplantation confers an increase in mortality, reflecting the severity of the disease itself or its treatment, specifically the treatment effects upon the transplanted organ.
Other Noninfectious Pulmonary Complications of Solid-Organ Transplantation
Many pulmonary complications that arise pretransplantation may not resolve completely or immediately posttransplantation. Metastatic pulmonary calcifications can occur in patients with kidney or hepatic failure, and while usually benign, can lead to restrictive lung disease. Furthermore, their nodular appearance on chest radiographs can mimic nodules from infection or malignancy (see the previous section).
Pulmonary conditions such as alveolar simplification and primary ciliary dyskinesia (PCD) can be associated with congenital heart disease, including those associated with heterotaxy, and they may not have been recognized before heart transplantation. These conditions should be considered if unexpected pulmonary complications including prolonged hypoxemia and recurrent atelectasis develop in the posttransplant period.
Hepatopulmonary syndrome (HPS) and porto-pulmonary hypertension (PPHTN) are two entities that occur in end-stage liver disease and can significantly improve with liver transplantation. However, improvement is not universal or even expedient. In the case of HPS, significant hypoxemia can occur because of dilation of pulmonary capillaries, and this may persist in the postoperative period, leading to prolonged mechanical ventilation and the use of supplemental oxygen. Typically, resolution of hypoxemia from HPS occurs over the initial 8 months following transplant.
Severe cases of PPHTN may preclude liver transplantation since the operative mortality increases as the severity of pulmonary hypertension increases. Additionally, PPHTN can recur or occur de novo after liver transplantation. Posttransplant pulmonary hypertension (PHTN) can occur in those with pretransplant HPS, those with recurrent liver disease, and those with no apparent liver disease and isolated PHTN. Severe PHTN in either the pretransplant or the posttransplant period increases the risk of mortality. Continuous infusion of prostaglandins can serve to lower pulmonary pressures, acting as a bridge to transplant and attenuating surgical risk.
Venous thromboemboli (VTE) can occur in the posttransplant period, thus leading to respiratory distress and hypoxemia, but they are uncommon in pediatric patients. Renal transplant patients may be at higher risk because of manipulation of pelvic veins, although this has mainly been reported in adult patients.
Pulmonary Complications of Childhood Tumors and Their Treatment
Pulmonary complications of solid organ and hematopoietic stem cell transplant may be preexisting conditions either from the underlying malignancy or from the initial treatment for malignancy.
Childhood cancers are treated with a combination of surgery (primarily for solid tumors), chemotherapy, and radiotherapy. See Chapter 59 for a further discussion of pulmonary toxicity from chemotherapy. Radiation therapy is an integral component of curative treatment for a variety of oncologic malignancies, both for the treatment of primary tumors and for those patients with distant metastases. A common site for metastases is the lung, particularly in diseases such as Wilms tumor, sarcomas, and hepatoblastoma. Whole lung irradiation (WLI) is often used as adjuvant therapy for patients with distant lung metastases. Unfortunately, lung tissue is particularly sensitive to the effects of radiation, and the untoward effects of WLI are a concern, both during and well beyond the end of therapy. In addition, total body irradiation (TBI) is commonly used as part of pretransplant conditioning regimens.
Therapeutic doses of radiation can cause acute (radiation pneumonitis) and chronic (radiation fibrosis) lung injury. The incidence of acute radiation pneumonitis is up to 15% of adults treated for breast and lung cancers and Hodgkin disease, but the incidence in pediatric patients is less well described. The incidence may be dependent on the radiation dose, fractionation, and volume of lung exposed, as well as concurrent chemotherapy. At thoracic radiation doses approximating 1000 cGy or more, total lung capacity and diffusing capacity may be significantly impaired. Some chemotherapeutic agents (bleomycin, dactinomycin) have additive toxicity with radiation, and others (actinomycin D and Adriamycin) can result in a “radiation recall” effect, with delayed presentation of toxicity, up to 6 weeks later.
Acutely, radiation injury results in the release of a number of proinflammatory cytokines that can cause subendothelial and perivascular damage. Over time, this causes type II pneumocyte hypertrophy and infiltration of inflammatory cells. Radiation pneumonitis typically presents 30–90 days after radiation therapy, with symptoms of cough, dyspnea, hypoxemia, and pleuritic pain. Examination may reveal crackles or a pleural friction rub, and chest radiography may show diffuse haziness or ground-glass densities. Early injury may be detected by decreases in diffusing capacity for carbon monoxide. The differential diagnosis for this presentation includes infections and other etiologies that must be excluded. Treatment of radiation pneumonitis usually involves systemic corticosteroids, based on a murine model of radiation toxicity.
Radiation fibrosis may appear 6–24 months after radiation therapy. It is usually but not always preceded by symptoms of radiation pneumonitis, and itself most commonly presents with progressive dyspnea. Radiographically, there may be streaky densities, decreased lung volume or atelectasis, or pleural thickening. Pulmonary function findings include hypoxemia, a restrictive defect, and decreased diffusing capacity. Histologic findings include pleural and subpleural fibroses, interstitial fibrosis, interstitial pneumonitis, interlobular septal fibrosis, and obliteration of pulmonary vessels ( Fig. 64.5 ). Unfortunately, there is no useful treatment for radiation fibrosis. Some patients with severe lung disease because of radiation fibrosis have undergone lung transplantation. As such, efforts should be focused on prevention (with considerations of shielding, dose, and effects of other agents) and close monitoring for patients at high risk.

Analysis of self-report data from the Childhood Cancer Survivor Study cohort showed that in survivors who were more than 5 years from diagnosis and received radiation to the chest or TBI, there was a statistically significant increase in the relative risk of developing late pulmonary complications. These included lung fibrosis, emphysema, recurrent pneumonia, chronic cough, persistent shortness of breath, and abnormal chest wall development. As more children survive cancer and live well into adulthood, it is important to consider the late effects associated with WLI. Beyond the lung parenchyma itself, radiation can also impair the growth of the muscle, cartilage, and bone of the thorax. Studies in adults receiving WLI suggest that mild restrictive disease usually resolves over a period of 2–4 years. However, the ongoing development of the chest wall and the lung in children makes the impact of such toxic therapy quite different. Continued alveolar multiplication occurs until 2–4 years of age, and alveolar enlargement continues for some time after that. The growing chest wall may also be impacted by radiation treatment. Weiner and colleagues reported a retrospective review of pulmonary function in 30 children who had received WLI for treatment of malignancy. At a median of almost 3 years post radiation, 20% of subjects had moderate or severe reduction in FEV 1 , 43% had moderate or severe reduction in TLC, and 43% had moderate or severe reduction in diffusing capacity. At least two patients demonstrated a clear progressive decline over time. These authors suggested that recognition of early abnormalities might allow for earlier intervention for radiation pneumonitis (e.g., corticosteroid therapy) and that it cannot be presumed (as in adults) that loss of lung function after radiation is transient.
Benoist and coworkers followed 48 children treated with WLI for Wilms tumor for 2–17 years following treatment. Two subjects had clinical evidence of radiation pneumonitis shortly after treatment, which resolved within 3–8 weeks. In this study, the percentage of patients with abnormal TLC or lung compliance increased over time, with reduced TLC observed in 50% at 6 months and greater than 90% at 6 years. These authors posit that early lung function abnormalities are caused by parenchymal lung injury, while later effects result from impaired chest wall growth. These findings argue for continued pulmonary follow-up of children treated with thoracic irradiation.
Pulmonary Complications of Hematopoietic Stem Cell Transplantation
Significant advances in transplantation immunology as well as innovations in chemotherapy and irradiation have allowed HSCT to become a more viable therapy for the treatment of hematologic diseases. The science of bone marrow transplantation, which began as the allogeneic transplantation of whole bone marrow, has progressed to include allogeneic altered marrow (e.g., T cell depleted), autologous marrow, and HSCT. Despite many advances, even the most experienced transplant centers encounter significant posttransplantation morbidity and mortality. Infectious and noninfectious pulmonary complications remain common following marrow transplantation in both adults and children. While most studies of HSCT recipients deal with adults rather than children, many important principles apply across age barriers. Although different sources of stem cells for transplantation (e.g., allogeneic or autologous) portend different risks of particular complications, we will describe potential complications in a more general fashion and will refer to relative incidences when appropriate. In addition, we will refer to these procedures collectively as HSCT.
Pretransplant Factors
Some preexisting diseases that themselves are treated with HSCT may have significant pretransplant pulmonary complications. For example, sickle cell disease (SCD) can be complicated by previous acute chest syndrome and pulmonary infarction, and patients with underlying malignancies may have been treated with irradiation targeting the lung or with pulmonary toxic chemotherapy including bleomycin, busulfan, or cyclophosphamide. These all may affect lung function prior to HSCT.
It is critical that patients previously exposed to treatments that are potentially “pneumotoxic” have pre-HSCT pulmonary screening ( Table 64.2 ), including the determination of lung volumes, flows, diffusing capacity, and respiratory muscle strength. The monitoring of PFTs remains useful during the posttransplantation period and can be especially important as adjuncts to radiographic evaluation of intercurrent illness affecting the lung. Recent reports suggest that pre-HSCT pulmonary function can predict post-HSCT pulmonary complications as well as survival. Musculoskeletal weakness is both a common preexisting condition and a known complication of HSCT ; several relatively simple tests (6-minute walk test and respiratory muscle strength test) are useful in identifying patients at risk, allowing for musculoskeletal rehabilitation and nutritional support in both the pre- and post-HSCT period.
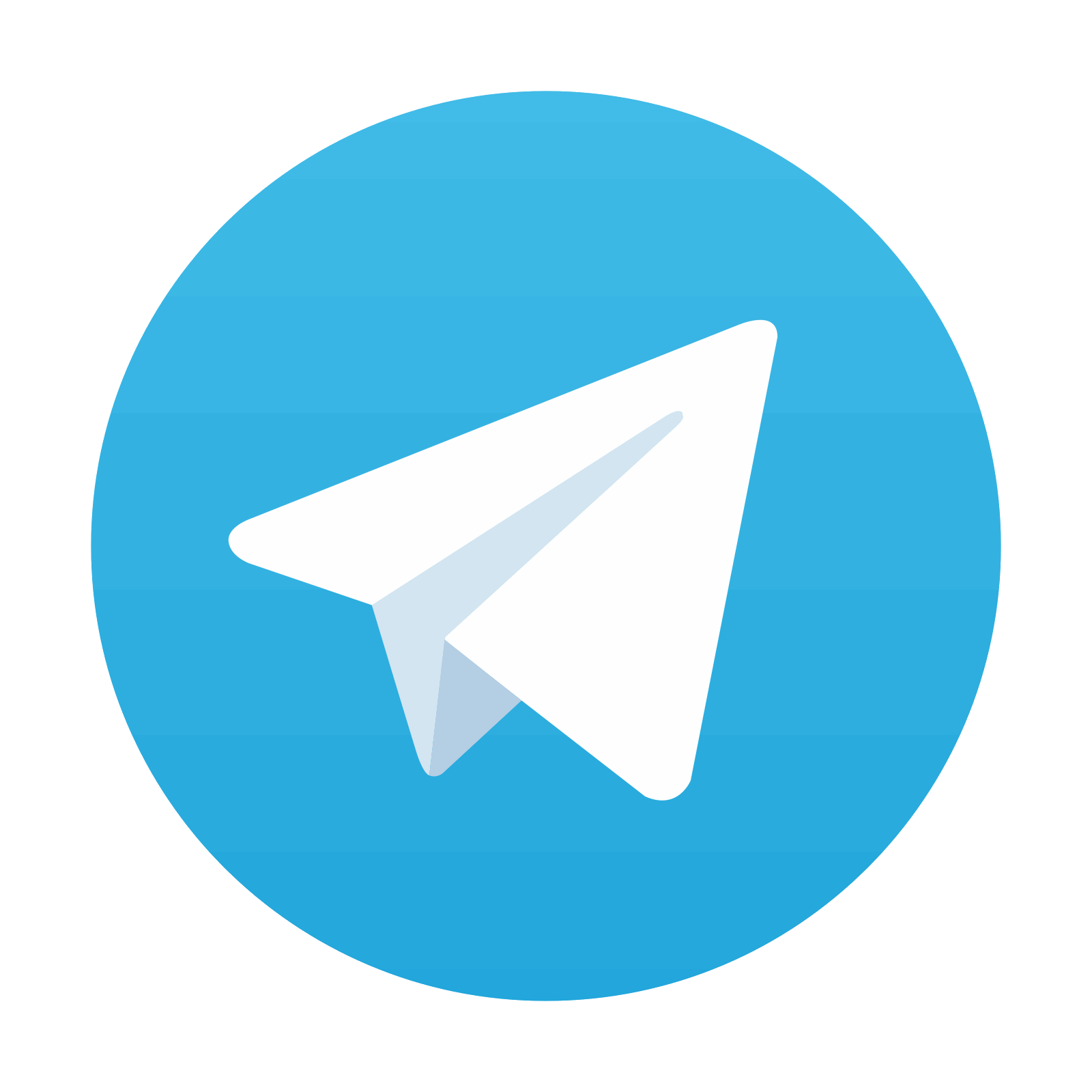
Stay updated, free articles. Join our Telegram channel

Full access? Get Clinical Tree
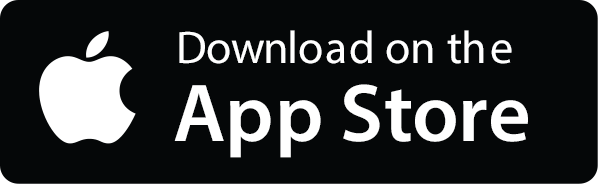
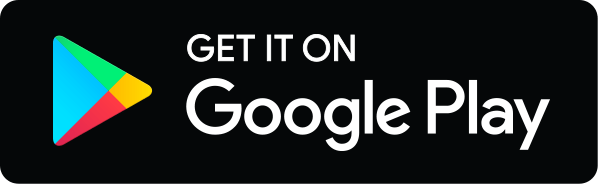
