Abstract
Congenital heart disease affects nearly 1% of births. Unrepaired cardiac disease, the postoperative state after congenital heart surgery, and long term of sequelae of certain congenital repairs can significantly adversely impact pulmonary vascular function. Upper airway obstruction can be caused by unrepaired vascular rings, and lower airway obstruction can be caused by plastic bronchitis after single ventricle palliation. Lung parenchymal disease from cardiac bypass is a well-recognized entity caused by ischemic/reperfusion injury and transfusion. Pulmonary vascular conditions such as postoperative pulmonary hypertension have seen significant improvements, whereas treatment of pulmonary vein stenosis continues to be extremely challenging. Developing new therapies will be critical in improving clinical outcomes in many of these conditions.
Keywords
congenital heart disease, vascular rings, pulmonary hypertension, pulmonary vein stenosis, pulmonary reperfusion injury, tetralogy of Fallot, single ventricle physiology
Introduction
Both acute and chronic pulmonary disease may be present in the setting of various forms of congenital heart disease. Postoperative atelectasis, pulmonary edema, or pleural effusions are commonly seen after cardiac surgery, particularly when cardiopulmonary bypass is required. Atelectasis with resultant ventilation-perfusion mismatch, pulmonary edema, and pulmonary hypertension (not specifically in association with congenital heart disease) are discussed elsewhere within the text, and this chapter will focus on more specific pulmonary conditions that may be encountered in association with congenital heart disease.
Airway Compression Due to Vascular Rings and Slings
Background
Pulmonary vascular rings are congenital vascular conditions in which the trachea and esophagus are encircled by vascular structures, as first reported and surgically repaired by Dr. Robert Gross in 1945. Vascular rings are considered rare and thought to represent only approximately 1% of all congenital heart conditions.
Vascular rings develop secondary to abnormalities of embryological vascular development as described below. During normal fetal development, the aortic sac gives rise to six paired vascular branchial arches that connect to the right and left dorsal aortae ( Fig. 39.1 ). The dorsal aortae give rise to intersegmental arteries at each segmental level that connect to the vertebral arteries. Part of the aortic sac becomes the ascending aorta, and the distal portions of the right and left dorsal aorta fuse to become the descending aorta. The proximal left-sided dorsal aorta remains patent and becomes the proximal descending aorta, and the proximal right-sided dorsal aorta regresses. The third, fourth, and sixth branchial arches contribute primarily to the development of the aortic arch and proximal head and neck vessels. The third branchial arches become the common carotid arteries. The right fourth branchial arch becomes the proximal right subclavian artery, and the left fourth branchial arch becomes the transverse aortic arch. The seventh right intersegmental artery becomes the distal right subclavian artery, and the seventh left intersegmental artery becomes the left subclavian artery. The proximal right sixth branchial arch becomes the right pulmonary artery, and the distal right sixth branchial arch regresses. The proximal left sixth branchial arch becomes the left pulmonary artery, and the distal left sixth branchial arch becomes the patent ductus arteriosus that eventually becomes the ligamentum arteriosum upon closure.

The most common type of vascular ring is the double aortic arch ( Figs. 39.2 and 39.3 ). The ascending aorta gives rise to left-sided and right-sided aortic arches with symmetrical takeoff of carotid and subclavian arteries before connecting with the proximal descending aorta. This occurs if the right-sided dorsal aorta and right fourth branchial arch, which normally regress, persist. The normal left-sided dorsal aorta and left fourth branchial arch also persist. In this situation, the trachea and esophagus are completely encircled by left and right aortic arches.


The second most common type of vascular ring is when there is a right aortic arch in association with an aberrant left subclavian artery ( Figs. 39.4 and 39.5 ). The head and neck vessel branching order is as follows: left carotid artery, right carotid artery, right subclavian artery, and aberrant left subclavian artery that takes a retroesophageal course. This occurs when the right-sided dorsal aorta and right fourth branchial arch persist, the left-sided dorsal aorta and left fourth branchial arch regress, but the left dorsal aorta remains patent. The trachea and esophagus are completely encircled by the right aortic arch and left ligamentum arteriosum which spans the proximal left pulmonary artery and the distal transverse aortic arch near the insertion of the left subclavian artery. Dilation of the proximal left subclavian artery or Kommerell diverticulum is often seen in the setting of a right aortic arch with an aberrant left subclavian artery. This can also cause tracheal and esophageal compression. In addition to double aortic arch and right aortic arch with aberrant left subclavian artery, there are additional complete vascular and incomplete vascular rings such as circumflex aorta, persistent fifth aortic arch, and cervical aortic arch. However, these are less common.


Although not a true vascular ring, a pulmonary artery sling is a condition when the left pulmonary artery connects directly to the right pulmonary artery instead of connecting to the pulmonary trunk ( Figs. 39.6 and 39.7 ). The left pulmonary artery then courses posterior to the trachea and anterior to the esophagus causing tracheal compression. Pulmonary slings can be associated with primary tracheal stenosis with extended segments of complete tracheal rings.


Tetralogy of Fallot with absent pulmonary valve is a particular variation of this condition that can be associated with significant respiratory compromise ( Fig. 39.8 ). In addition to presence of a ventricular septal defect, overriding aorta, pulmonary stenosis, and right ventricular hypertrophy, a thick ring of tissue at the pulmonary valve annulus in place of a pulmonary valve results in significant pulmonary stenosis and regurgitation. Massive dilation of the main and branch pulmonary arteries can result in significant compression of the trachea and bronchi.

Clinical Presentation
Pulmonary vascular rings typically present with respiratory or gastrointestinal symptoms. Respiratory signs and symptoms may include noisy breathing, barking cough, wheezing, frequent upper respiratory infections, apneic episodes, or intermittent cyanosis. Gastrointestinal symptoms typically do not present until patients begin eating solid foods. The symptoms may include a sensation of food being stuck in the throat, slow eating, or hyperextension during feedings. Typically, patients with double aortic arches tend to present earlier in life with more profound symptoms than patients with right aortic arch and an aberrant left subclavian artery. Backer et al. reported a series of patients with double aortic arch typically presenting before one month of age and those with right aortic arch and aberrant left subclavian artery generally between 1 and 6 months of age. Patients having a pulmonary artery sling may also have associated “ring-sling” complex in which the trachea is not only compressed by the posterior left pulmonary artery, but the presence of complete tracheal rings that also create further narrowing of the airway. The ring-sling complex is associated with significant respiratory symptoms with high morbidity and mortality. Patients with tetralogy of Fallot with absent pulmonary valve syndrome present with significant respiratory distress during the neonatal period in slightly less than half of all cases. They are often cyanotic initially, although this seems to improve with time.
Diagnosis
Chest x-ray can often suggest the diagnosis of a vascular ring from the position of the aortic knob, apparent tracheal narrowing, and/or unilateral hyperinflation of the lung. Barium swallow has also been used to diagnose vascular rings based on patterns of esophageal compression. Echocardiography may be used to diagnose a vascular ring by delineating the course of the aorta and proximal and neck vessels. A secondary imaging modality is usually required since the airway cannot be evaluated by ultrasound. Echocardiography is nonetheless recommended in patients diagnosed with vascular rings as many have associated congenital heart abnormalities.
In the current era, pulmonary vascular rings are now typically evaluated by tomographic imaging, either computed tomography (CT) with contrast or magnetic resonance imaging (MRI) with or without contrast. Computed tomography allows the airway and vascular anatomy to be evaluated rapidly, oftentimes without the need for sedation. MRI provides excellent evaluation of the blood vessels with the advantage of avoiding ionizing radiation. The airway can be evaluated using spin echo techniques. However, longer acquisition times often require sedation in younger patients.
Bronchoscopy is an important technique in evaluating some patients with vascular rings. Direct observation of the airway can be very useful in determining the both the degree and mechanism of airway obstruction.
Treatment
Patients with a vascular ring who are symptomatic are treated surgically. In the case of double aortic arch, the vascular ring is typically relieved by resection of the smaller nondominant arch, often through a thoracotomy ( Fig. 39.9 ). Patients with a right aortic arch and aberrant left subclavian artery are treated by division of the associated ligamentum arteriosum. Patients with a right aortic arch and aberrant left subclavian artery may have dilation of the proximal left subclavian artery, which is called a Kommerell’s diverticulum. The Kommerell’s diverticulum can cause direct compression of the airway or the esophagus. Resection of the Kommerell’s diverticulum and reimplantation of the left subclavian artery may be required ( Fig. 39.10 ). Pulmonary vascular slings often require translocating the anomalous left pulmonary artery coming from the right pulmonary artery onto the main pulmonary artery. If complete tracheal rings are also seen in the presence of the pulmonary vascular sling, a slide tracheoplasty may be required ( Fig. 39.11 ). Patients with tetralogy of Fallot with absent pulmonary valve syndrome who develop respiratory distress during the neonatal period may benefit from prone positioning and often require mechanical ventilation. Airway compression can be addressed surgically. Standard strategies include reducing the size of the pulmonary arteries and repositioning the pulmonary arteries anteriorly (LeCompte maneuver). There is not currently a consensus as to the best approach.



Pulmonary Hypertension and Postoperative Pulmonary Hypertensive Crisis
Background
Pulmonary hypertension occurring during the postoperative period for congenital heart surgery is a serious condition resulting in significant morbidity and mortality. Mortality related to postoperative pulmonary hypertension was reported to be as high as 20% during prior eras. With a better understanding of the risk factors related to pulmonary hypertensive crises, surgical repair of congenital heart defects at younger ages, and the development of more effective treatments, the incidence of this condition has decreased significantly, with the current incidence estimated to be 2%–7%.
Risk Factors
Patients undergoing surgical repair of certain types of congenital heart disease are at higher risk of developing postoperative pulmonary hypertension. These include total anomalous pulmonary venous return, truncus arteriosus, transposition of the great arteries, ventricular septal defect (particularly with late repair), complete atrioventricular canal (with moderate to large ventricular septal defect component), and hypoplastic left heart syndrome. Preexisting pulmonary hypertension as well as the presence of certain chromosomal abnormalities (trisomy 21, trisomy 18, trisomy 13) may also increase the risk of developing postoperative pulmonary hypertensive crises. Cardiopulmonary bypass itself increases the risk of pulmonary hypertension because of endothelial injury, impaired production of nitric oxide, release of endothelin and thromboxane, and release of microemboli. Pulmonary hypoxia and ischemia during cardiopulmonary bypass and subsequent pulmonary reperfusion injury may also contribute to a state that may favor development of pulmonary hypertensive crises.
Pathophysiology
Pulmonary hypertensive crises are particularly dangerous, since they lead to both pulmonary as well as cardiac dysfunction. Increased pulmonary arterial pressure and resistance result in right ventricular dilation and right ventricular dysfunction. Right ventricular dilation leads to right-to-left shift of the interventricular septum and resultant compression of the left ventricle. Left ventricular compression decreases left ventricular compliance and therefore causes a decrease in pulmonary venous return and cardiac output. Distention of the pulmonary arteries and resultant pulmonary edema leads to decreased lung compliance and hypoxia, hypercapnia, and respiratory acidosis.
Evaluation and Monitoring
Pulmonary hypertensive crises may begin with nonspecific findings such as tachycardia and systemic hypotension. Subsequent progression to bradycardia and poor perfusion may signal impending cardiac arrest. Unless there is an intracardiac shunt present, oxygen saturations may often be normal or near normal until profound clinical deterioration occurs. Several modalities are useful in detecting clinically significant pulmonary hypertension. Presence of an increased gradient between the arterial pCO 2 from a blood gas, and end-tidal CO 2 from capnography may indicate decreased pulmonary blood flow. Direct hemodynamic monitoring using intravascular catheters are also useful in the critical care setting. A right atrial line can assess right ventricular filling pressures and measure mixed venous oxygen saturations to estimate oxygen delivery. Pulmonary arterial lines can directly measure pulmonary arterial pressures.
Echocardiography is another important noninvasive tool to evaluate pulmonary arterial pressure. The systolic pulmonary arterial pressure can be estimated by measuring the peak systolic velocity of the tricuspid regurgitation jet from spectral Doppler. Similarly, mean pulmonary arterial pressures can be estimated measuring the end-diastolic velocity of the pulmonary insufficiency jet by spectral Doppler. Right ventricular size, right ventricular systolic function, and interventricular septal contour and position can all be assessed qualitatively using two-dimensional echocardiography.
Treatment
Pulmonary hypertensive crises can be minimized through careful monitoring and treatment by several means. Appropriate sedation and pain control is extremely important to minimize pulmonary vascular resistance. Fentanyl may be particularly useful in this setting, and paralysis is also used. Appropriate oxygenation and ventilation is important in maintaining low pulmonary vascular resistance, as both hypoxia and acidosis may cause pulmonary vasoconstriction. Although alkalosis promotes pulmonary vasodilation, high tidal volumes over time can damage alveoli and result in pulmonary edema. Conversely, inadequate tidal volumes can lead to alveolar collapse and resultant hypoxia.
Current pharmacologic treatment of pulmonary hypertension involves the alteration of three mechanisms of pulmonary vasodilation: the nitric oxide/cyclic guanosine monophosphate (cGMP) pathway, the prostacyclin (PGI2)/cyclic adenosine monophosphate (cAMP) pathway, and the endothelin-1 pathway (see Chapter 35 ). Inhaled nitric oxide is a selective pulmonary vasodilator, which has rapid onset of action. Inhaled nitric oxide has been used to treat postoperative pulmonary hypertension in patients who have undergone repair of obstructed total anomalous pulmonary venous return, mitral stenosis, and nonrestrictive ventricular septal defects. It has also been shown to be beneficial in single ventricle patients with Glenn and Fontan circulations by improving pulmonary blood flow, oxygen saturations, and central venous pressures. Sildenafil has also been used as an adjunct to therapy when transitioning from inhaled nitric oxide to prevent rebound pulmonary hypertension. Inhaled prostacyclin has also been shown to be effective in treating pulmonary hypertension in the postoperative setting. Although bosentan therapy may be initiated in the intensive care setting, its effect on pulmonary hypertension is not rapid, so it is not effective in treating a pulmonary hypertensive crisis.
In addition to the pulmonary vasodilators mentioned previously, treatment of heart failure is critical in the management of patients with postoperative pulmonary hypertension. Milrinone infusion, in addition to decreasing pulmonary and systemic vascular resistance, improves left ventricular contractility and diastolic function. Systemic vasodilation may require initiation of a secondary agent. Norepinephrine, phenylephrine, and vasopressin may also be helpful in supporting cardiac function in the setting of pulmonary hypertensive crisis.
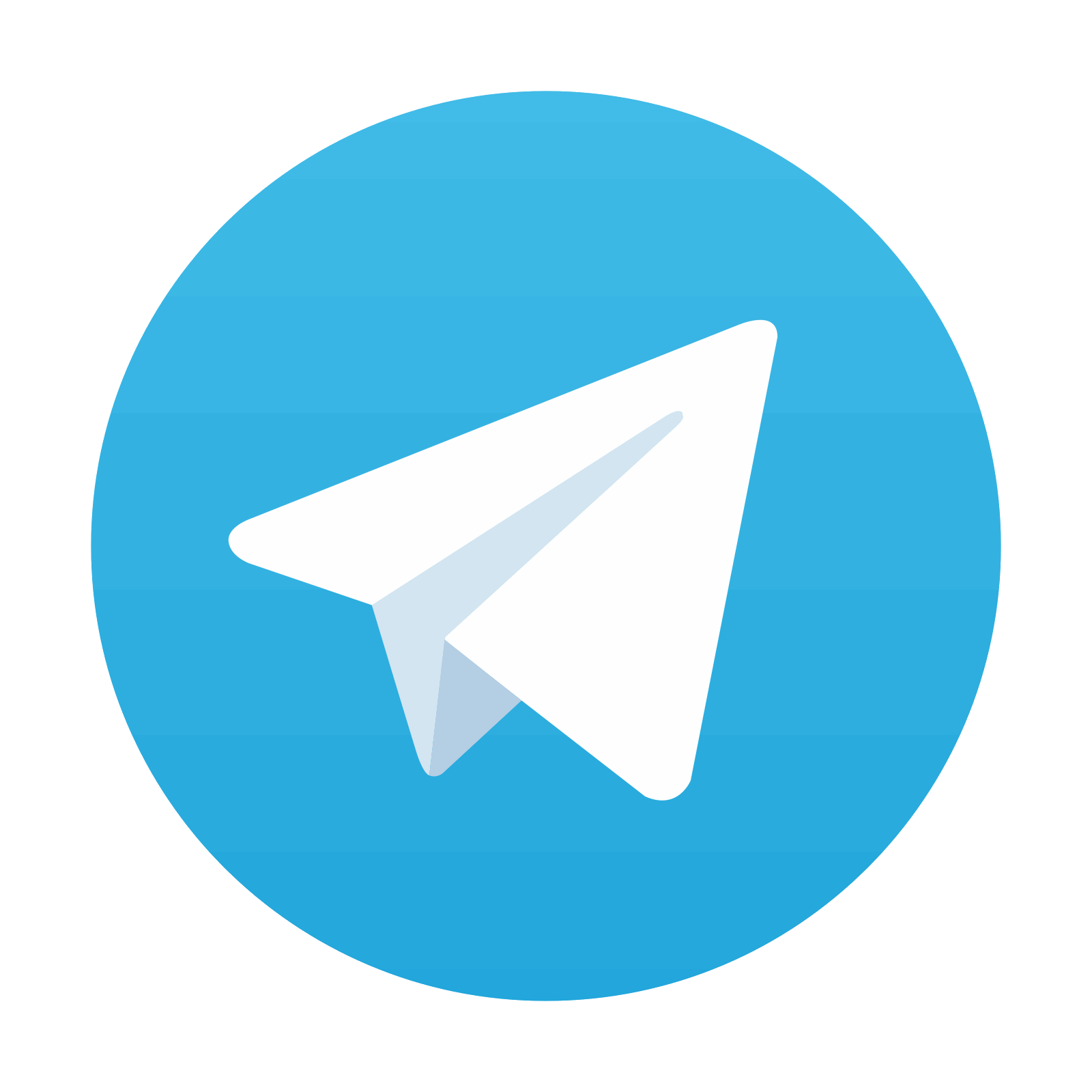
Stay updated, free articles. Join our Telegram channel

Full access? Get Clinical Tree
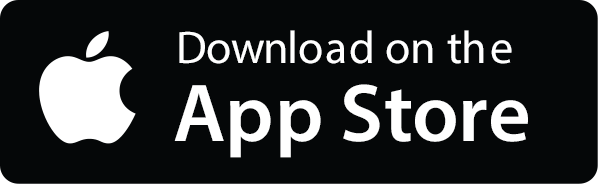
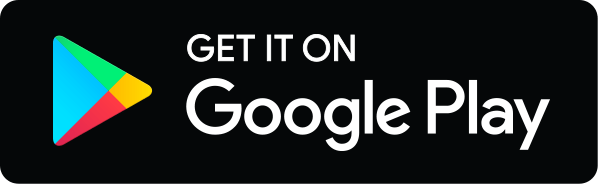