Chapter 93
Prosthetic Grafts
Zheng Qu, Elliot L. Chaikof
Synthetic conduits were introduced to modern arterial reconstructive surgery by Voorhees et al1 in 1952 with the development of a vascular graft woven from fibers of Vinyon-N, a copolymer of vinyl chloride and acrylonitrile. Despite successful early clinical outcomes, first-generation vascular prostheses lacked long-term durability. Within 5 years, graft dilatation and aneurysmal degeneration were frequent, with an 80% loss in tensile strength.2,3 A variety of candidate materials for synthetic arterial conduits were examined throughout the 1950s, including solid rigid metals, glass, and silk. Their failure led to the supposition that porosity and resistance to thrombosis were critical features for an arterial substitute. Innovations in polymer science and engineering in the period surrounding World War II led to the synthesis of polytetrafluoroethylene (PTFE; e.g., Teflon) in 1938 and polyethylene terephthalate (PET; e.g., Dacron) in 1941. Both polymers, when engineered as fibers or as nonwoven fabric, proved to be more durable and biocompatible and have remained commercial standards. In the aortic position, the performance of prosthetic grafts is excellent, with susceptibility to graft infection the remaining major limitation. In the pediatric population, the inability of commercially available prostheses to match the growth of the child is an additional limitation of current synthetic large-caliber conduits.
Prospective randomized clinical studies have confirmed that PET and PTFE differ little in their long-term performance characteristics when used in the infrainguinal position.4 However, both display significantly lower patency than vein grafts because of greater risk of thrombosis and anastomotic neointimal hyperplasia. The inherent thrombogenicity of available polymeric materials and the presence of a chronic injury site at the anastomosis caused, in part, by a compliance mismatch between the prosthesis and host artery contribute to late graft occlusion. These failure modes exist whether a synthetic conduit is used for lower extremity revascularization or for arteriovenous hemodialysis access, even when the prosthesis has an inner diameter of 6 mm or larger. Currently, coronary and infrageniculate lower extremity revascularization requires the use of conduits smaller than 6 mm in diameter. Despite significant effort by industrial and academic research groups over the past half-century, a durable, synthetic, small-diameter vascular graft does not exist.
Attempts to address these failure mechanisms and the complete absence of a small-diameter conduit have led to collaborative interactions among vascular surgeons, material scientists, biologists, and engineers. Novel materials with improved mechanical responses continue to be synthesized, and new surface engineering schemes along with systemic antithrombotics have been devised to reduce the risk of thrombus formation. As the distinction between “biologic” and “synthetic” continues to be blurred, the design of “living,” biologically functional structures may ultimately supplant autologous vein grafts as the ideal small-diameter conduit. Such a development would revolutionize the field.
Materials
Dacron Prosthesis
Dacron is the DuPont trademark for PET, a highly durable polyester thermoplastic polymer that can be processed into synthetic fibers. DeBakey introduced the first knitted Dacron prosthesis in 1957; it was used extensively for arterial reconstruction of the thoracic and abdominal aorta, as well as proximal peripheral vessels.5 This pioneering work, combined with developmental and clinical studies by Szilagyi, Wesolowski, Sauvage, and Cooley, contributed to the popularization of Dacron grafts.6
Historically, weaving and knitting have been the two common techniques for fabrication of Dacron fibers into a tubular conduit. The knit structure involves looping fibers in an interlocking chain, which yields a soft and stretchable fabric. In contrast, a woven structure assembles the yarn in an over-and-under pattern in the lengthwise and circumferential directions (Fig. 93-1).7–9 Woven grafts are stronger and less porous than knitted grafts but are less compliant and may fray when cut. Typically, highly porous knitted Dacron grafts required “preclotting,” a process that involved exposing the graft to an aliquot of the patient’s blood before heparinization. Currently, most commercial knitted grafts are impregnated with gelatin,10 collagen,11 or albumin.12 Despite some evidence that coated grafts may induce a greater inflammatory response than preclotted grafts,13,14 the two display similar patency rates.15,16
Figure 93-1 Scanning electron microscopy of typical yarn configurations in Dacron grafts. A, DeBakey standard-knit Dacron vascular prosthesis (original magnification ×37). B, Vascutek Gelsoft Köper knitted prosthesis sealed with gelatin (×40). C, Woven Dacron (×50). (A from Snyder R, et al: Woven, knitted, and externally supported Dacron vascular prostheses. In: Stanley JC, editor: Biologic and synthetic vascular prostheses, New York, 1982, Grune & Stratton; B from Hake U, et al: Evaluation of the healing of precoated vascular Dacron prostheses. Langenbecks Arch Chir 376:323, 1991; C from Salzmann DL, et al: Inflammation and neovascularization associated with clinically used vascular prosthetic materials. Cardiovasc Pathol 8:63, 1999.)
Characteristically, knitted grafts incorporate a velour finish that orients the loops of yarn upward, perpendicular to the fabric surface, thereby increasing the available surface area and enhancing the anchorage of fibrin and cells to promote tissue integration. The preference for a velour finish has been motivated primarily by improved handling characteristics with little data demonstrating that internal, external, or double velour grafts exhibit greater patency rates.17 Dacron grafts are often crimped longitudinally to increase flexibility, elasticity, and kink resistance. However, these properties are lost soon after implantation as a consequence of tissue ingrowth.
Several modifications of Dacron grafts have been approved for clinical use. To reduce surface thrombogenicity, grafts coated with bioactive heparin or passivated with fluoropolymers have been developed.18 A silver-coated Dacron graft has also been introduced to decrease the occurrence of graft infection. Long-term follow-up studies will be required to determine whether these modifications improve outcomes.
Early versions of knitted grafts were prone to dilatation over time, with a 10% to 20% increase in graft size immediately after implantation,19 followed by slow expansion.20 Nonetheless, correlation of graft dilatation and structural failure has not been established.21 Most Dacron grafts have been extremely durable. In 1997, the U.S. Food and Drug Administration (FDA) disclosed a total of 68 cases of structural failure occurring at an average of 7.4 years after implantation.22 Given that approximately 60,000 aortic reconstructions are performed annually, the overall rate of graft failure is small.22 A single-center review noted a 0.2% structural failure rate at a mean follow-up of 12 years.23
ePTFE Prosthesis
PTFE is a fluoropolymer that was trademarked as Teflon by DuPont in the late 1930s. It is chemically inert, hydrophobic, and mechanically durable. PTFE was initially used as a fiber in textile-based grafts, but today it is predominantly processed into expanded PTFE (ePTFE) tubes by extruding the polymer resin through a die. Expanded PTFE grafts were first implanted in animals in 1972,24 and Campbell et al25 reported their initial clinical use in 1976.
Macroscopically, the surface of ePTFE is smoother than Dacron’s, and the grafts are soft and pliable. On a microscopic scale, the extrusion process results in a porous morphology consisting of solid islands or nodes linked by fibrils (Fig. 93-2). This node-fibril structure occupies about 20% of the total volume of the expanded polymer and results in a higher ratio of pores to material than in Dacron grafts. However, the void space in ePTFE grafts is considerably smaller than the voids in woven or knitted material. Furthermore, the inherent hydrophobic nature of the fluoropolymer establishes a natural barrier to water that prevents permeation of blood. Despite the chemical inertness of ePTFE, plasma proteins and platelets adhere to the surface, and the host response is similar to that to Dacron.26
Figure 93-2 Scanning electron microscopy of node-fibril structure in expanded polytetrafluoroethylene grafts (original magnification ×500). (From Lumsden AB, et al: Nonporous expanded polytetrafluoroethylene grafts reduces graft neointimal hyperplasia in dog and baboon models. J Vasc Surg 24:825, 1996.)
In standard ePTFE grafts, fibril length measures about 30 µm, although there have been experimental variants with a larger fibril length of 60 µm, which in animal models facilitated luminal endothelization.27 However, these observations have not been replicated in clinical studies. Thin-walled ePTFE has a wall thickness of 200 to 300 µm, compared with a standard wall thickness of 400 to 600 µm. This increases compliance of the graft and improves handling, but with some loss of suture retention strength and an inability of the prosthesis to be used for repeated dialysis access. Clinical performances of thin- and standard-walled ePTFE grafts are otherwise similar.28 Stretch ePTFE is a modification of standard ePTFE in which a microcrimping process is applied to compress the fibrils. This property allows the graft to extend longitudinally as the fibrils are stretched to their full length, providing some improvement in handling characteristics. Kink resistance of ePTFE grafts has been augmented by the application of external plastic rings. A recognized limitation of ePTFE grafts is their relative stiffness, which leads to a compliance mismatch between the prosthesis and host artery that may contribute to anastomotic intimal hyperplasia. In addition, because ePTFE does not display self-sealing characteristics, early access for hemodialysis is precluded by bleeding at the puncture site.
Despite some evidence suggesting that platelet deposition29–31 and complement activation32 are lower on ePTFE than on Dacron prostheses, the patency rates of Dacron and ePTFE grafts are similar.4 Heparin- and carbon-coated ePTFE prostheses have become commercially available, but data confirming increased patency rates over unmodified grafts are limited. It has been suggested that intimal hyperplasia may be related to adverse local hemodynamic effects. This has led to the use of a vein cuff at the distal anastomosis, with reports of improved 3-year patency rates for below-knee femoral-popliteal bypass (45% versus 19%).33 A clinical study has demonstrated that grafts in which a distal PTFE cuff is incorporated have patency rates similar to those with a vein cuff.34
Hybrid Stent-Grafts
A hybrid stent-graft consists of a bare metal framework that is located inside, outside, or within prosthetic graft material such as Dacron or ePTFE. Depending on the device, this framework may span the entire length of the graft or be restricted to its ends. Metal hooks and barbs may be incorporated into the design so as to reduce migration. Stent-grafts have been used in the endovascular repair of aortic aneurysms as an alternative to open repair and as such have been associated with less morbidity, mortality, and faster recovery. Stent-grafts have also been used in peripheral applications to treat vascular trauma and occlusive and aneurysmal disease of arteries and dialysis arteriovenous fistulae. These applications have been detailed in Chapters 95 and 96.
First clinical uses of stent-grafts occurred, independently, in Argentina35 and the Ukraine35a more than 20 years ago. In addition to exclusion of arterial aneurysms and vessel defects, stent-grafts are designed to reduce tissue infiltration and intimal hyperplasia that may occur with bare metal stents.36 Two broad classes of stent-grafts are currently in use. Balloon-expandable stent-grafts are manufactured using stainless steel in a crimped state and are positioned on a balloon. On deployment they are expanded to vessel diameter by balloon inflation. Self-expandable configurations are fabricated using nitinol in an expanded state and then crimped and constrained by a covering sheath. These stent-grafts expand to a predefined dimension upon sheath retraction at the delivery site.37 Because of their potential for precise positioning and minimal foreshortening and recoil, balloon-expandable stent-grafts are preferentially deployed adjacent to vessel ostia, bifurcations, or side branches.38 Self-expandable stent-grafts are preferred in peripheral applications because they exhibit greater flexibility and are less prone to collapse and deformation by external forces that may exist in superficial locations, such as the neck and thigh. Self-expandable stent-grafts should be used when relatively long vessel coverage is needed or in the presence of an associated target vessel dissection.39,40
In the treatment of an aortic aneurysm, a stent-graft creates a proximal and distal pressure seal that excludes the aneurysm from circulation. The aneurysm sac may thrombose within hours of the procedure and stabilize or even shrink over time. In approximately 20% to 25% of cases, postoperative “endoleak” may occur, as incomplete seal or patent branch vessels facilitate blood flow into the aneurysm sac. This may lead to sac pressurization and increased risk of rupture, and as a result, endovascular aneurysm repair requires lifelong surveillance to detect endoleak and aneurysm expansion.41 Although open aortic aneurysm repair is associated with durable results, the minimally invasive nature of the endovascular stent-grafting approach is more appealing for older patients and in patients with significant concomitant comorbid diseases.42
When a stent-graft is used in a peripheral application, intimal hyperplasia may develop at the interface between the device and uncovered vessel where the injured intima is not covered by graft material.43 Thrombotic occlusion continues to be a significant complication with a reported 1-year incidence of 17%.44 Finally, the impact of the unavoidable occlusion of perforating side branches adjoining the treated area is uncertain.45 Despite lack of long-term evidence supporting the use of stent-grafts as a primary approach in peripheral applications, their use in treating long-segment superficial femoral artery disease may have advantages and in the setting of vessel rupture is warranted.46,47 The effect of heparin modification of self-expandable stent-grafts has yet to be determined in controlled clinical trials.48
Polyurethane Prosthesis
The development of a polyurethane vascular prosthesis was largely motivated by a desire to reduce compliance mismatch. Polyurethane is more elastomeric than Dacron or ePTFE and has been used as a polymeric coating for pacemaker leads, breast implants,49 and barrier films for dermal wounds.50 Polyurethane is a copolymer that consists of three different monomer types—a diisocyanate hard domain, a chain extender, and a diol soft domain. At physiologic temperatures, the soft domains provide flexibility, whereas the hard domains impart strength. The most common medical-grade polyurethanes are based on soft domains made from polyester, polyether, or polycarbonate. Varying the composition of the monomer repeat units enables the mechanical properties of polyurethane prostheses to be tuned.
Polyester-based soft domains were used in the earliest generation of polyurethane vascular grafts. Good biocompatibility was observed in vivo, but the hydrolytic susceptibility of the ester linkage led to chemical deterioration.51,52 In a small clinical study of below-knee bypass grafts, more than half occluded within 1 year.53 Biodegradation is also a concern because some degradation products may be carcinogenic. The FDA terminated the use of polyurethane foam in breast implants in 1991 as a result of the release of 2,4-toluene diamine, which caused liver cancer in animals.54 However, most polyurethanes studied today incorporate a different monomeric diamine subunit.
A more hydrolytically stable polyether-based polyurethane graft was developed later, but the ether linkage remains susceptible to oxidative degradation in vivo.52 Vectra (Bard Peripheral Vascular, Inc., Tempe, Ariz) is the only commercially available graft based on polyether urethane and is used solely for hemodialysis access.55 It may be punctured within 24 hours of implantation because of its self-sealing characteristics. A second polyurethane graft known as the Aria graft (Thoratec Corporation, Pleasanton, Calif) had been subjected to clinical study but is no longer being produced.56
The latest generation of polycarbonate-based polyurethanes is inert to both hydrolysis and oxidation in both in vitro and animal studies.57–59 The Corvita graft (Corvita Corporation, Miama, Fla), a composite polyurethane and Dacron prosthesis, did not dilate in dogs after a 1-year implantation period but is no longer in development.60 The Expedial graft (LeMaitre Vascular, Inc., Burlington, Mass) was a polycarbonate-based polyurethane graft impregnated with heparin. This prosthesis underwent evaluation for hemodialysis application but is no longer under development.61
Choice of Prosthetic Graft Based on Indications and Anatomic Location
Although several new vascular grafts have become commercially available in the past decade, little has changed in graft selection. Additional data are available on long-term patency and durability (Table 93-1)8,18,33,34,62–68; however, graft selection remains dictated largely by handling characteristics, convenience, surgeon preference, and cost. The following sections evaluate the available arterial substitutes (Table 93-2) and provide general guidelines for their most suitable anatomic application.
Aortic Reconstruction
Synthetic arterial prostheses have performed best as large-diameter replacements for the thoracic or abdominal aorta. Dacron grafts display patency rates higher than 95% at 5 years.69 Similar results have been observed for ePTFE grafts, which may be somewhat more resistant to infection.70,71 Overall, late clinical outcomes appear to be independent of graft type whether the grafts are used in open surgery or as components of endovascular grafts for the repair of abdominal or thoracic aneurysms.
Infrainguinal Reconstruction
Controversy exists over the preference of a synthetic graft for above-knee revascularization. An operative procedure with a synthetic graft is simpler, and some have proposed that the saphenous vein be reserved for future operations72 because patency rates for secondary revascularization are higher when it is performed with vein than with prosthetic grafts.73–75 However, ePTFE grafts have a higher occlusion rate than vein grafts,76,77 and prosthetic grafts lead to a greater number of secondary operations.78 Late patency rates are higher with vein than with prosthetic grafts for femoropopliteal bypass (61%-76% vs. 38%-68%).78–80 Thus, we agree with the proposition that autologous bypass conduits should be used whenever possible because of their significantly higher long-term durability.81 Nonetheless, a prosthetic graft may be an appropriate choice in selected patients with disabling claudication and good runoff.
When autologous vein is not available, synthetic grafts are acceptable substitutes. Although ePTFE is currently the most widely used graft material for above-knee femoropopliteal bypass, the long-term patency rates of Dacron and ePTFE prostheses in this position are generally similar.4,65,82–84 Heparin-bonded Dacron displayed significantly better patency than did unmodified ePTFE in the above-knee femoropopliteal position at 3 years in one study, but this difference was not apparent at 5 years.63 During prolonged follow-up in another study, Dacron femoropopliteal bypass grafts exhibited better patency than ePTFE after 5 years, but not at 2 or 10 years.85
Saphenous vein is preferred for below-knee infrapopliteal bypass because of a patency rate of approximately 70% at 5 years.79,86,87 Reported patency rates for synthetic grafts in this position range from 18% to 41% at 3 years.88–91 An adjunctive venous cuff, patch, or boot, as well as an arteriovenous fistula, has been used at the distal anastomosis to improve graft patency (see Chapter 110). A prospective, randomized multicenter trial found that a vein cuff significantly improved the 2-year patency rate of ePTFE grafts (52% vs. 29%).92 Corroborating these results, another study found that use of a Miller vein cuff more than doubled the 3-year patency rate of noncuffed grafts (45% vs. 19%).33 Interposition of a Linton patch93 or Taylor patch94 has also been found to improve patency. Adopting the geometry of such adjuncts, one study of an ePTFE graft (Fig. 93-3) with an expanded distal cuff (Distaflo, Bard Peripheral Vascular, Inc.) demonstrated 1-year patency comparable to that of grafts that had been modified with a vein cuff.34
Figure 93-3 Externally supported expanded polytetrafluoroethylene graft with a modified distal cuff. (Adapted from Panneton JM, et al: Multicenter randomized prospective trial comparing a pre-cuffed polytetrafluoroethylene graft to a vein cuffed polytetrafluoroethylene graft for infragenicular arterial bypass. Ann Vasc Surg 18:199, 2004.)
Extra-Anatomic Reconstruction
Axillofemoral, femorofemoral, and axilloaxillary bypasses require a much longer, subcutaneously placed prosthetic graft. This requirement often precludes the use of autologous vessels because of insufficient length. These grafts are often externally supported with a removable continuous spiral coil to reduce kinking and compression. Expanded PTFE and Dacron grafts have similar patency rates in these positions.95–98 For carotid-subclavian bypass, some reports have indicated better patency rates with either ePTFE or Dacron than with vein grafts.99–101
Other Locations
Prosthetic grafts display excellent long-term patency for visceral and renal arterial reconstruction as a result of short graft length, high blood flow, and absence of extrinsic mechanical compression. Large-caliber venous reconstruction of the inferior or superior vena cava102–105 and the iliofemoral,102 jugular,106 and portal veins,107 including portosystemic shunts,108,109 is typically performed with externally supported ePTFE grafts. Adjunctive anticoagulation is generally required, except in patients with portal hypertension.
Hemodialysis Access
Optimal selection of the currently available vascular access options, which include autologous fistulae, prosthetic grafts, and double-lumen cuffed catheters, remains a challenge in the care of patients undergoing long-term hemodialysis. In the United States, the Fistula First Initiative has encouraged nephrologists, vascular access surgeons, and dialysis units to increase fistula use from 24% to 52% between 2000 and 2008. In that time frame, graft use decreased from 58% to 22%, and catheter use increased from 17% to 26%.110 A comparison of the current hemodialysis options is detailed in Section 13.
Prosthetic grafts for arteriovenous (AV) access are indicated in patients with failed AV fistulae, exhausted superficial veins, or unsuitable vessels. Insertion sites for AV grafts in the upper limb are preferred because of the lower risk of associated sepsis. Upper extremity grafts can be placed in the forearm or upper arm and may have straight or looped configurations. Arterial inflow may include the brachial, axillary, and less commonly, wrist arteries, whereas outflow varies among the axillary, brachial, cephalic, basilic, and median cubital veins. When arm sites are exhausted, the thigh is the next favored site, where the graft is usually looped between the common femoral artery and the proximal great saphenous vein.111 The advantages of AV grafts include ease of placement, a short time from operation to utilization (2-3 weeks), and a potentially large surface area for cannulation. Grafts largely fail because of restenosis from intimal hyperplasia at the venous anastomosis as well as thrombosis and infection, which occur at higher rates than in fistulae.111,112 The commonly used grafts are constructed from ePTFE, though a newer polyurethane graft (Vectra) has the advantage of almost immediate use with less needle site bleeding complications.113 Heparin bonded grafts do not appear to perform better than conventional ePTFE grafts.114
Failure Modes for Prosthetic Vascular Grafts
In general, failure of prosthetic vascular conduits can be classified as early, midterm, or late. Technical problems during surgery and the presence of underlying thrombophilia are common causes of early graft failure within the first month after surgery. Graft occlusion occurring between 6 months and 3 years after surgery is usually due to anastomotic intimal hyperplasia. Late graft thrombosis is frequently secondary to progression of distal atherosclerotic disease. Graft infection is relatively uncommon but most often requires removal of the prosthesis. The interaction among blood, host artery, and graft initiates a complex set of biochemical and cellular responses that ultimately limit implant function.
The Blood-Material Interface
The concept of a blood-compatible material has been driven by strategies to minimize activation of prothrombotic responses. Coagulation is a normal defense mechanism that responds almost instantaneously to injury and is a precursor of the inflammatory responses that constitute the healing process. The full cast of this response includes a combination of lipids, proteins, and cells that act in concert to provide localized amplification (Fig. 93-4). A series of negative feedback mechanisms control the propagation and termination of these responses. Because many of these antithrombotic mechanisms are localized to the endothelium, the inability of current prostheses to replicate these complex systems plays a large role in eventual graft failure.
Figure 93-4 Simplified view of biomechanical phenomena at the distal end-to-side anastomosis (graft-artery interface). On a macroscopic scale, fluid dynamics and wall shear stress near the anastomosis encourage intimal hyperplasia. Microscopically, a host of inflammatory and thrombotic responses mediated by cellular and protein components could lead to anastomotic intimal hyperplasia. Incomplete transanastomotic ingrowth results in poor endothelial coverage in the midgraft area, thereby potentiating graft thrombosis. C5, C5a, Components of complement; ECM, extracellular matrix; EGF, epidermal growth factor; FGF, fibroblast growth factor; GFs, growth factors; IL, interleukin; PDGF, platelet-derived growth factor; TGF, transforming growth factor; TNF, tissue necrosis factor.
Protein Interactions
One of the earliest events that occurs when blood encounters an artificial surface is the adsorption of proteins. The composition of the adsorbed protein layer plays a direct role in the thrombotic response by activating the coagulation and complement cascades. The adsorption process is largely determined by the size, charge, and structure of the protein and related surface physiochemical properties, including topography and chemical composition. Inhibiting the adsorption of thrombogenic proteins in vivo remains a significant challenge.
Protein Adsorption.
Transport of proteins to a surface occurs by both convection and diffusion. Convective transport is determined by blood velocity and flow profile. However, very close to the surface, within a region referred to as a boundary layer, protein transport occurs primarily by diffusion.115,116 Other properties, such as charge, the tendency of a protein to unfold, and hydrophobicity, affect the affinity of bound proteins to the surface. Overall, this dynamic change in surface protein composition over time is referred to as the “Vroman effect.” That is, surface composition is initially governed by different rates of diffusion for each molecular species, but at later times specific binding affinities and exchange reactions with other proteins dictate the equilibrium surface composition of the adsorbed species.117 Bound protein typically unfolds or denatures to expose domains that trigger events ultimately resulting in thrombosis.
Complement Cascade.
Activation of the alternative pathway of the complement system may contribute to surface-induced thrombosis. Binding of C3b or C3 to a foreign surface and subsequent binding of factor B results in the formation of a complement complex that stimulates an inflammatory response. This serves as a prelude to platelet activation as well as monocyte and neutrophil infiltration, all of which have been implicated in the development of neointimal hyperplasia and may also inhibit endothelialization of the lumen of the graft.118
Coagulation Cascade.
Although the coagulation system is dealt with in greater detail elsewhere in this text, we must emphasize here that molecular events at both blood- and tissue-material interfaces initiate the coagulation cascade. Binding of plasma factor XII to a foreign surface results in a conformational change that yields an activated form, XIIa, which catalyzes the activation of factor XI and leads to the production of Xa. Factor Xa converts prothrombin to thrombin, the main effector of this cascade.119,120 In addition, tissue factor, invariably expressed at sites of injury that occur with the creation of an anastomosis, triggers the formation of factor VIIa, which generates factors IXa and Xa. Both physical and biochemical events serve to enhance or limit propagation of the coagulation cascade. Blood flow modulates this process by controlling the rate at which coagulation proteins and activated factors are delivered to or removed from the graft surface. For example, locally disturbed flow or eddies at an anastomosis may create recirculation regions that localize coagulation factors. Although the negative feedback control of clot propagation is complex, it is important to note that antithrombotic systems include circulating factors that may limit the generation of thrombin as well as inactivate thrombin after its formation.
Cellular Interactions
Platelets.
The platelet, the major cellular component of the thrombotic response,121 is involved in both complement-mediated and T-cell–mediated immune responses.122 Adsorption of fibrinogen, fibronectin, or von Willebrand factor onto a graft surface facilitates the deposition and aggregation of platelets. Platelets provide a reactive phospholipid surface for the coagulation cascade to propagate. In turn, thrombin stimulates the release of platelet granule contents, such as serotonin, adenosine diphosphate, and calcium, that support further platelet activation and aggregation. Chemoattractants such as platelet factor 4 and β-thromboglobulin are also released and promote an inflammatory response.
Increased levels of platelet-generated thromboxane and decreased platelet counts continue to be observed 1 year after graft implantation in animals,123 and clinical studies have revealed persistent platelet deposition on Dacron grafts for at least several months.124–126 Early studies have shown that antiplatelet agents can reduce platelet deposition onto prosthetic grafts.127,128 More detailed discussion of adjunctive antiplatelet therapies can be found in the later section on pharmacologic adjuncts for improving long-term graft function.
Neutrophils and Macrophages.
Recruitment of circulating neutrophils to the graft surface is mediated by chemoattractants such as C5a and leukotriene B4.129 Neutrophils interact with surface-bound proteins such as fibrinogen, immunoglobulin (Ig) G, C3bi, and factor X through cell surface integrins,130 as well as with glycoproteins on adherent platelets.131,132 At sites of vascular injury, endothelial cells upregulate adhesion molecules such as intercellular adhesion molecule-1 and vascular cell adhesion molecule-1, which also increase neutrophil and monocyte recruitment.133,134 Despite their role in the phagocytosis of foreign material and bacteria, neutrophils recruited to a biomaterial surface do not protect against infection.135
Although infiltration of neutrophils occurs initially after graft implantation, neutrophils are short lived and are replaced by monocytes, which differentiate into macrophages. Macrophages produce proteases, chemotactic factors, reactive oxygen radicals, complement components, and coagulation factors, and these substances contribute to a chronic inflammatory state that inhibits re-endothelialization. Macrophages form foreign body giant cells in a layer covering the surface of ePTFE grafts.136,137
The production of cytokines appears to depend, in part, on the material activating the leukocytes. Monocytes and macrophages produce interleukin-1β, interleukin-6, and tumor necrosis factor-α138 in greater amounts when in contact with Dacron than when ePTFE is used.139 In addition, activated macrophages exposed to ePTFE or Dacron express tumor necrosis factor-α as well as several growth factors that may stimulate smooth muscle cell (SMC) proliferation and contribute to intimal hyperplasia.140,141
Endothelial and Smooth Muscle Cells.
Migration of endothelial cells from the perianastomotic area in humans is highly inefficient and results in the formation of a neointima extending no more than a few centimeters from the anastomoses with little coverage of the midportion of the graft. However, scattered islands of endothelial cells have been observed far from the anastomotic regions, which may be due to transmural ingrowth of microvessels from perigraft tissue27,142–144 or adherence of blood-borne endothelial progenitor cells (EPCs).145–147 Indeed, Dacron grafts implanted in dogs appear to be rapidly covered with bone marrow–derived hematopoietic stems cells.148
Despite efforts to increase endothelial cell coverage, endothelial cells growing onto prosthetic graft surfaces that display a procoagulant phenotype can, in principle, promote rather than retard thrombosis. Furthermore, activated endothelial cells may increase growth factor production and secretion and thereby encourage SMC proliferation. Indeed, subintimal SMC proliferation occurs predominantly in areas that have an overlying endothelium.149 As an additional example, ePTFE grafts coated with anti-CD34 antibodies and implanted in pigs were found in one study to capture EPCs and increase endothelial cell coverage; however, at 4 weeks, intimal hyperplasia at the distal anastomosis was significantly greater.150
The turnover of SMCs at the anastomotic interface peaks 2 weeks after graft implantation and persists at a level higher than in the native artery. SMCs in the resulting neointima also display increased production of growth factors151 and secrete higher amounts of collagen.152,153 Inflammatory cells likewise produce a variety of growth factors that increase proliferation of SMCs and production of extracellular matrix (ECM).154,155
Infection
The incidence of graft infection ranges between 1% and 6%,156,157 and infection is associated with a 50% amputation rate and a 25% to 75% mortality rate.158 Graft infection is often a result of the assembly of a bacterial biofilm that protects bacteria from antibody and cell-mediated attack159,160 and reduces antibiotic effectiveness.161 Attempts to decrease bacterial infection have included binding rifampicin, silver, and other antibacterial compounds onto the luminal surface of grafts. However, the effectiveness of rifampicin-coated162–164 or silver-coated grafts62,165 has been difficult to ascertain.
The Tissue-Material Interface
Porosity
Measurement of porosity depends on the graft material. In textile grafts such as Dacron, porosity is defined by water permeability, whereas the porous nature of ePTFE grafts is defined by the average internodal distance. Optimization of graft porosity was driven by a desire to improve vascular wall healing under the presumption that a transmural angiogenic response would lead to endothelialization of the lumen of the graft.166 For example, ePTFE grafts with internodal distances of 60 or 90 µm demonstrated greater transmural tissue ingrowth and luminal coverage of endothelial cells than did grafts with 30-µm pores in a primate model.27,167,168 Nonetheless, a clinical trial using 90-µm ePTFE grafts did not show a benefit over standard 30-µm grafts.169
Compliance
Compliance is defined as the percentage change in diameter of a cylindrical conduit between diastolic pressure and systolic pressure. Compliance mismatch between a relatively extensible host vessel and stiff graft leads to a region of excessive mechanical stress that may contribute to anastomotic intimal hyperplasia as well as pseudoaneurysm formation.170 In addition, native vessels have a dynamic compliance that is inversely proportional to blood pressure. At low physiologic pressure, native vessels may be better able than noncompliant prosthetic grafts to preserve pulsatile energy and optimize flow.171 Compared with a native artery (compliance of 0.059%/mm Hg) and vein (0.044%/mm Hg), Dacron (0.019%/mm Hg) and ePTFE (0.016%/mm Hg) grafts are relatively noncompliant,172 whereas polyurethane grafts have a compliance similar to that of the host artery.172 It also bears noting that the compliance of synthetic grafts may decrease after implantation because of the formation of a surrounding fibrous capsule, which stiffens the graft wall. As a result, the impact of initial compliance on long-term patency may be difficult to assess.173
Hemodynamics
Anastomotic intimal hyperplasia (IH) is a common cause of prosthetic graft failure, in part because of local flow disturbances (e.g., flow separation, recirculation, reattachment, stasis, and reflux) and alterations in wall shear stress. Decreased neointimal thickness has been observed with the placement of a distal venous cuff,174 patch,175 or arteriovenous fistula176,177 in animal models, which initially was attributed to increased compliance at the distal anastomosis.178 However, no difference was noted between grafts with a vein cuff and those in which the vein cuff was wrapped with ePTFE, thus suggesting that modulation of local fluid dynamics plays the dominant role in reducing IH.170,179 Indeed, local flow conditions may modulate endothelial cell function.180 In an end-to-side anastomosis, disturbed flow may drive endothelial cells to an activated, prothrombotic phenotype.181 Activated endothelium, in turn, promotes proliferation and migration of SMCs and production of matrix proteins.182 Thrombus formation may also be potentiated by regions of stasis created by low blood velocity as well as by high shear rates, which activate platelets locally.183
Examination of failed and failing prosthetic bypasses with and without vein cuffs revealed that the extent of IH is not lower in cuff-supplemented anastomoses than in direct anastomoses, but the greatest IH is redistributed from the cuff-artery interface to the expanded upstream region around the graft-cuff interface, which appears to delay restenosis.174,184,185 As illustrated by experimental flow visualization and numerical methods, the cuff geometry may generate a vortical flow structure that enhances mixing in the cuff cavity to reduce stagnation regions and flow separation near sites prone to IH (e.g., toe, heel, and floor of host artery as shown in Fig. 93-4).186,187
The use of vein cuffs does not cause any apparent harm and facilitates a technically challenging distal anastomosis, but their use for femorodistal bypass cannot be recommended on the basis of clinical studies to date, which have yielded conflicting results. Only one randomized clinical trial from the Joint Vascular Research Group showed significant benefit in favor of a Miller vein cuff at the distal anastomosis for below-knee popliteal ePTFE arterial bypass.33,188 The later randomized Scandinavian Miller Collar Study (SCAMICOS) showed no benefit from using a vein cuff in ePTFE grafts.189 A 2012 meta analysis that included both randomized trials and three cohort studies showed that the use of a vein cuff slightly improved primary patency as well as limb survival at 2 years, but not at 1 or 3 year intervals.190 The distally widened ePTFE graft that mimics a vein cuff has been available for clinical use (Distaflo) since 2005, and is an adequate alternative to the vein cuff.34,191 Advances in numerical simulations have yielded valuable data on velocity distributions near the anastomosis, which has led to the rational design of grafts that modulate local blood flow.183 Indeed, helical grafts have been developed with better cross-plane mixing, a characteristic that has been reported to improve graft patency in an animal model192 as well as in a pilot clinical study.193
Biofunctional Vascular Prostheses
Thromboresistant Surface Engineering
The generation of nonfouling surfaces that resist protein adsorption and subsequent platelet deposition and activation has been pursued within a framework in which it is postulated that thermodynamic factors at the blood-material interface drive protein adsorption. Specifically, protein adsorption is favored by electrostatic and hydrophobic interactions between the adsorbed protein and synthetic surface and by the local increase in entropy that occurs upon the displacement of water molecules and counter-ions from the first few nanometers of the material surface. Both synthetic and natural materials, including polyethylene oxide (PEO), pyrolytic carbon, albumin, phosphorylcholine, and more recently, elastin-inspired protein polymers, have been investigated for the development of strategies to passivate the prosthetic surface by reducing or eliminating the enthalpic or entropic effects that drive protein and cell adsorption on a molecular level.
Inhibition of Protein and Cell Adsorption
Polyethylene Oxide.
In the early 1970s it was observed that PEO passively adsorbed onto glass surfaces prevented the adsorption of viruses, platelets, and thrombin, and subsequent studies have demonstrated that PEO exhibits among the lowest levels of protein or cellular adsorption of any known polymer. This property was attributed to the presence of a hydrophilic ether oxygen in its structural repeat unit, [CH2-CH2-O]n, which leads to a water-solvated structure that is capable of forming a “liquid-like” surface with highly mobile molecular chains that exhibit no systematic molecular order. Unlike in other hydrophilic polymers, such as polyhydroxyethylmethacrylate and polyacrylamide, the absence of surface charges and the presence of only small hydrophobic methylene groups in the structural repeat unit in PEO were thought to provide few other sites for protein or cellular binding.194 Surface modification with PEO has been attempted by several methods, including bulk modification, covalent grafting, and physical adsorption. In most cases, investigators have been able to demonstrate resistance to protein binding in vitro; however, in vivo results have been inconsistent, and clinical studies of a PEO-modified stent or graft have yet to be performed. For the interested reader, a comprehensive review of the blood compatibility of PEO can be found in a monograph by Lee et al.195
Albumin Coating.
Albumin as an inert, thromboresistant coating has been pursued since early studies of platelet interactions with various adsorbed proteins on artificial surfaces established that albumin induced significantly less platelet adhesion than did other plasma proteins, including fibrinogen and gamma globulin.196–199 Some groups have covalently grafted albumin to surfaces,200–202 whereas others have modified surfaces with long aliphatic chains (C8-C18) or PEO-tethered warfarin to increase selective affinity for endogenous albumin.203–208 Guidoin et al209–210 developed a glutaraldehyde–cross-linked albumin coating later manufactured by Bard Bard Peripheral Vascular, Inc. The Bard albumin-coated Dacron prosthesis was evaluated by Kottke-Marchant et al211 and found to display reduced platelet and leukocyte adhesion and aggregation in vitro as well as decreased fibrin production. In a thoracoabdominal bypass model in dogs, however, only small differences were observed between coated and uncoated grafts.212 In clinical studies, Al Khaffaf and Charlesworth213 and Kudo et al214 evaluated this prosthesis in the aortic position and demonstrated performance characteristics similar to those of other noncoated vascular prostheses.
Carbon Coating.
Pyrolytic, or graphitic, carbon coating of implantable materials has been investigated for a variety of blood-contacting devices, including vascular grafts, heart valves, and stents.215–217 In brief, pyrolytic carbon films are produced by chemical vapor deposition, a process in which a hydrocarbon, such as methane, is heated to its decomposition temperature and the graphitic layer allowed to crystallize as a highly ordered layer of carbon atoms. Early animal studies of carbon-coated vascular prostheses demonstrated improved patency rates in comparison with uncoated controls.218,219 However, surface irregularities on carbon-lined ePTFE grafts have contributed to poor performance in other investigations.220,221 More recently, 15-month implant studies in sheep documented reduced platelet adhesion and spreading on carbon-coated polyester grafts, but these observations did not affect overall histologic outcomes or patency rates.222 Furthermore, in a prospective, randomized multicenter study conducted in Germany, 283 patients received either 6-mm carbon-coated (Carboflo, Bard Peripheral Vascular, Inc.) or uncoated ePTFE grafts (Bard Peripheral Vascular, Inc.) for femoral–anterior tibial artery bypass. At 3-year follow-up, no significant differences were observed between the two groups with respect to patency or limb salvage.68
Phosphorylcholine Coating.
Planar-supported bilayers of phosphatidylcholine, the predominant glycerophospholipid in animal cell membranes, have been shown to reduce protein and cell adhesion in vitro.223–227 It has been proposed that this phenomenon is due to the zwitterionic nature of the phosphorylcholine head group, which, although carrying both positive and negative charges, is electrically neutral at physiologic pH. Application of supported lipid films as coatings for implantable devices has been limited by the inherent instability of a coating that is formed by individual molecules that “self-assemble” as a monolayer or bilayer film through relatively weak hydrophobic van der Waal interactions.228 Consequently, methods have been devised to create stable “membrane-mimetic” films through protein anchors,229,230 heat stabilization,231 and in situ polymerization of synthetically modified, polymerizable phospholipids.232–237 In all studies, the protein- and cell-resistant properties of the exposed phosphatidylcholine layer were retained.
Since 1984, a variety of polymethacrylate- and polyurethane-based polymers have been synthesized that incorporate the phosphorylcholine head group within the polymer backbone.238,239 For example, Yoneyama et al240–242 demonstrated excellent in vivo blood compatibility of a segmented polyether urethane/2-methacryloyloxyethyl phosphorylcholine (MPC) polymer blend processed as a coating for Dacron prostheses. Chen et al243,244 evaluated 4-mm-diameter ePTFE grafts coated with a copolymer of MPC and laurylmethacrylate in a canine femoral arteriovenous shunt; significantly reduced platelet deposition was demonstrated as well as limited neointimal hyperplasia at anastomotic sites. Similarly, ultraviolet-polymerizable, acrylate-modified phospholipids assembled on the lumen of a 4-mm-diameter ePTFE graft reduced platelet adhesion in an ex vivo baboon femoral arteriovenous shunt model.245 Direct chemical grafting of phospholipids on Dacron and ePTFE has been reported to reduce fibrinogen and platelet adhesion in vitro,246 and polyurethane-based grafts (d = 2 mm) modified with phosphorylcholine prevented thrombus formation after 8 weeks in a rabbit model.242
Elastin-Inspired Surfaces.
Elastin is a constituent structural protein in the vascular wall that elicits minimal platelet adhesion and aggregation.247,248 Because Dacron grafts coated with elastin inhibit SMC migration, it was suggested that elastin may also inhibit neointimal hyperplasia.249 However, the intrinsic insolubility of elastin makes its purification and processing from tissue difficult. This problem has largely been overcome by the identification of consensus sequences involved in the molecular assembly of elastin, which are used to construct a variety of elastin-mimetic protein polymers.250–255
Covalent immobilization of poly(VPGVG) on silicone was found to reduce fibrinogen and immunoglobulin adsorption and inhibit the secretion of proinflammatory cytokines from monocytes in vitro.256 In a second study, passive adsorption of recombinant elastin peptides onto polyurethane catheters decreased fibrin deposition and increased patency in a rabbit model.257 In a third study, ePTFE grafts (d = 4 mm) coated with a thin film of a recombinant amphiphilic elastin-mimetic protein polymer inhibited platelet deposition in an acute primate ex vivo shunt model.258
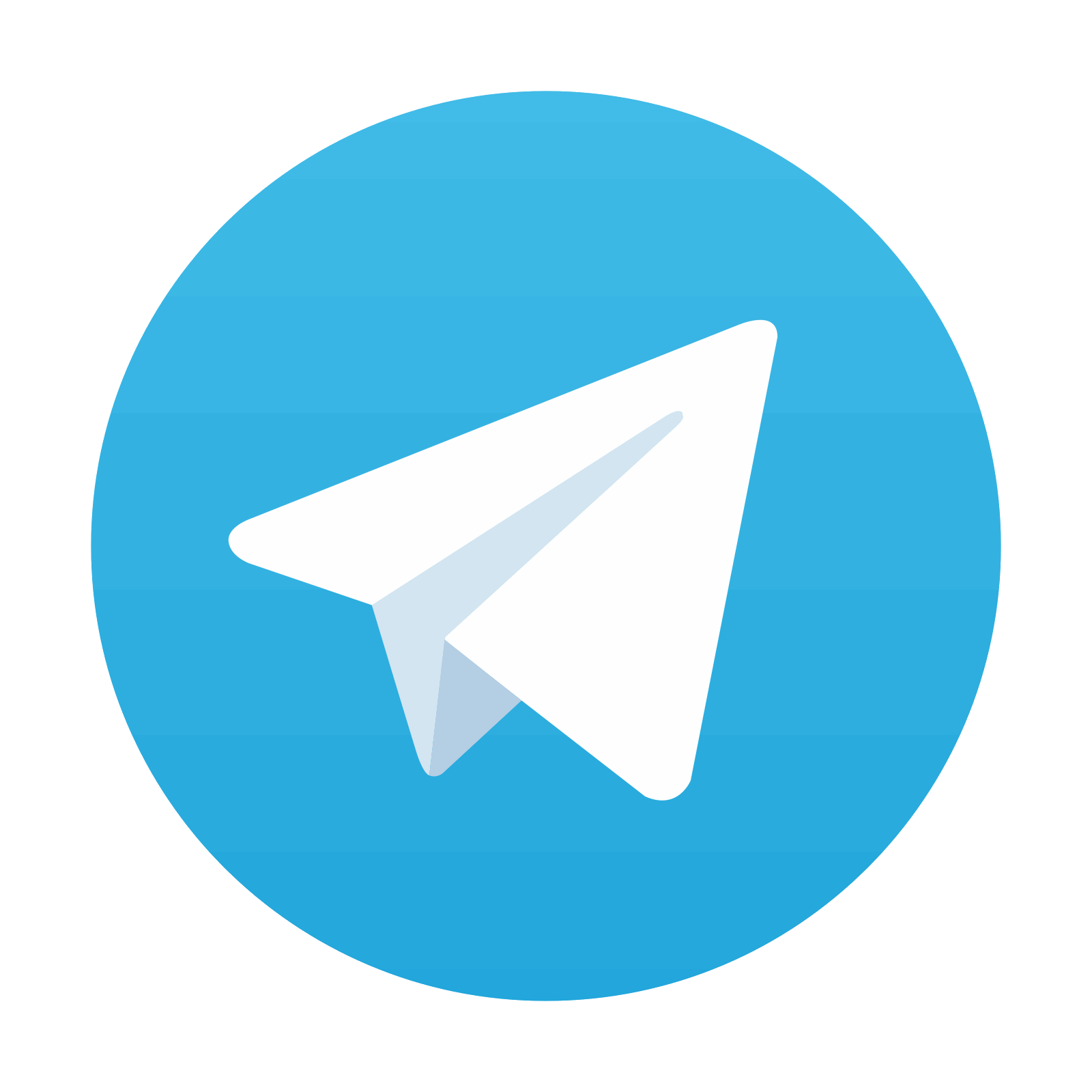
Stay updated, free articles. Join our Telegram channel

Full access? Get Clinical Tree
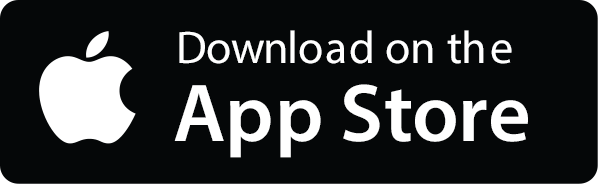
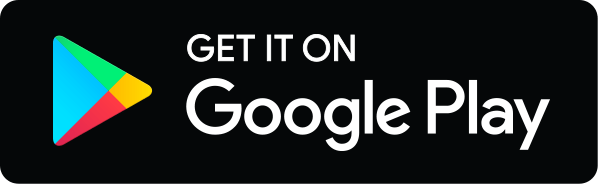