Background
Quantification of myocardial blood flow reserve in patients with coronary artery disease using real-time myocardial perfusion echocardiography (RTMPE) has been demonstrated to further improve accuracy over the analysis of wall motion and qualitative analysis of myocardial perfusion. The aim of this study was to determine the prognostic value of qualitative and quantitative analyses obtained by RTMPE in patients with known or suspected coronary artery disease.
Methods
From March 2003 to December 2008, 227 consecutive patients with normal left ventricular function who underwent RTMPE were prospectively studied. Replenishment velocity reserve (β) and myocardial blood flow reserve were derived from RTMPE. Primary outcomes were cardiac death, myocardial infarction and unstable angina with need for urgent coronary revascularization, and secondary outcomes were coronary bypass graft surgery or angioplasty.
Results
During a median follow-up period of 32 months (range, 5 days to 6.9 years), 19 major events (two deaths, six myocardial infarctions, and 11 episodes of unstable angina) and 46 total events occurred. Wall motion (hazard ratio [HR], 2.8; 95% confidence interval [CI], 1.4–5.6; P = .003) and qualitative myocardial perfusion analysis (HR, 4.3; 95% CI, 2.1–8.5; P < .001) were predictors of total events but not primary events. Abnormal myocardial blood flow reserve and abnormal β reserve were predictors of total events (HR, 8.1; 95% CI, 3–21; P < .001; and HR, 16.5; 95% CI, 5.5–49; P < .001) and primary events (HR, 3.8; 95% CI, 1–15; P = .048; and HR, 8.7; 95% CI, 1.8–40; P = .005). On multivariate analysis, only abnormal β reserve was an independent predictor of total (HR, 10.6; 95% CI, 2.5–43; P = .001) and primary (HR, 10.5; 95% CI, 1.5–6; P = .015) events. Abnormal β reserve added incremental value in predicting primary events (χ 2 = 2.0–13.2; P = .014).
Conclusions
Quantitative adenosine stress RTMPE added independent and additional prognostic information over wall motion and qualitative myocardial perfusion analysis in patients with known or suspected coronary artery disease and normal left ventricular function.
Coronary artery disease (CAD) remains the foremost important cause of death worldwide. Preventing early deaths and the late consequences of myocardial infarction using a cost-effective, nonionizing technique seems to be a reasonable part of the solution of this complex issue. To noninvasively detect CAD in patients with normal left ventricular function, avoiding unnecessary coronary angiography, stress echocardiography has been used successfully for many years. However, the spatial extent and severity of induced ischemia are important factors with regard to prognosis, and unfortunately, wall motion (WM) analysis by stress echocardiography does not accurately represent these factors.
Real-time myocardial perfusion (MP) echocardiography (RTMPE) has been demonstrated a useful technique for the quantification of MP and the determination of myocardial blood flow reserve (MBFR). The prognostic value of qualitative analysis of MP by RTMPE has been assessed using both dobutamine and high-dose dipyridamole stress echocardiography. Although the accuracy of MBFR with a cutoff value of 2.0 has been demonstrated to be accurate in the literature for detecting CAD, the predictive importance of MBFR obtained by RTMPE is still not clear. Also, there are no data comparing the prognostic value of WM with qualitative and quantitative assessments of MP in patients undergoing coronary angiography.
In this study, we sought to determine whether MBFR may add incremental prognostic value over conventional analysis of WM and MP in patients with known or suspected CAD and normal left ventricular function.
Methods
From March 2003 to December 2008, we prospectively studied 227 patients with known or suspected CAD who were referred for coronary angiography, met the inclusion and exclusion criteria, and underwent adenosine stress RTMPE. Inclusion criteria were age ≥18 years and normal left ventricular systolic function (ejection fraction ≥50%). Exclusion criteria were previous coronary artery bypass graft surgery, unstable angina, second-degree or third degree atrioventricular block, chronic obstructive pulmonary disease, severe valvular disease, severe ventricular arrhythmias, history of allergy to echocardiographic contrast media, presence of any intracardiac shunt, pregnancy, and breast feeding. The time interval between RTMPE and coronary angiography ranged from 1 to 57 days (median, 18 days).
The study protocol was approved by the ethics committee of the Heart Institute at the University of São Paulo Medical School, and all patients gave written informed consent to participate.
RTMPE
Complete resting two-dimensional echocardiography was performed, with special attention paid to the assessment of global and segmental left ventricular systolic function. Baseline left ventricular ejection fraction was calculated using the modified Simpson’s rule, and the left ventricle was divided into 17 segments for both WM and MP analysis. Commercially available ultrasound systems (Sonos 7500 and iE33; Philips Medical Systems, Bothell, WA) equipped with S3 broadband transducers and contrast echocardiographic software with low–mechanical index imaging was used for RTMPE. Imaging was performed in the apical two-chamber, three-chamber, and four-chamber views using power modulation mode with a mechanical index of 0.2, a frame rate of 25 to 30 Hz, and continuous intravenous infusion of either lipid-encapsulated microbubbles (Definity; Lantheus Medical Imaging, North Billerica, MA) or perfluorocarbon-exposed sonicated dextrose albumin (PESDA). The formulation of PESDA has been described elsewhere. Microbubble destruction was achieved using a packet of five high-intensity (mechanical index, 1.5) pulses (flash). Definity was diluted in 60 mL saline solution and infused continuously into the right antecubital vein after an initial bolus dose of 3 mL. After that, an infusion rate of 4.8 mL/min was begun, adjusting the rate according to the shadowing effect observed in the mid left atrial cavity in the four-chamber view projection, never exceeding a dose of 10 mL/min. PESDA was prepared by diluting a suspension of 0.1 mL/kg in 80 mL of 5% dextrose and was administered intravenously in a continuous fashion at a rate of 2 to 5 mL/min. The contrast infusion rate was adjusted until complete left ventricular cavity opacification and shadowing in the mid left atrial cavity were obtained. Once stable myocardial enhancement was reached, the contrast infusion rate was kept constant, and sequences of low-power perfusion images containing ≥15 cardiac cycles were acquired. After completion of resting perfusion sequences, contrast infusion was stopped, and hyperemia was induced by adenosine infusion through a parallel port, resulting in an infusion rate of 140 μg/kg/min for 6 min. After 3 min of adenosine infusion, contrast infusion was restarted at the same resting rate. After 4 min of adenosine infusion, perfusion sequences were acquired for hyperemic measurements.
End points of adenosine stress were achievement of the end of the infusion protocol, development of extensive WM abnormalities, or intolerable side effects. Blood pressure and cardiac rhythm were continuously monitored before, during, and 20 min after adenosine infusion. Twelve-lead electrocardiography was performed at baseline, at 2-min intervals during adenosine infusion, and at any time at the operating physician’s discretion.
Qualitative Analysis of WM and MP
Qualitative analysis of segmental myocardial contraction was based on visual assessment of myocardial thickening during optimal cavity contrast enhancement and was graded according to a scoring system as follows: 1 = normal, 2 = hypokinesis, 3 = akinesis, and 4 = dyskinesis. To maintain linearity with the MP analysis, the 17th segment was also scored for WM when an abnormality was observed at the very edge of the left ventricular apex. A test was considered positive for WM analysis if new segmental WM abnormalities were present in one or more segments. In the setting of resting segmental dysfunction, a segment was considered ischemic if it worsened by at least one score grade during adenosine stress. Qualitative analysis of MP was based on a visual assessment of subendocardial and transmural myocardial contrast enhancement after the flash impulse. The myocardial opacification during this phase was graded visually as follows: grade 1 = intense, grade 2 = reduced, and grade 3 = lack of opacification in the first four to five cardiac cycles after the flash impulse. Reduced myocardial opacification (grade 2) could represent either reduced intensity at all times or delayed appearance of contrast either compared with other segments or requiring >2 sec during stress. It was defined as positive if one or more segments exhibited a new grade 2 or 3 defect in one or more myocardial segments at peak stress. Both WM and MP analyses were performed separately, >1 month apart, by one experienced observer (W.M.), who was also blinded to prognostic findings.
Quantitative Analysis of RTMPE
Offline image analysis for quantification was performed with commercially available software (QLAB version 6.0; Phillips Medical Systems). Regions of interest were traced manually within the 17 segments of myocardium and in the adjacent left ventricular cavity. Myocardial plateau and adjacent left ventricular plateau signal intensities (in decibels) and the signal intensity exchange rate (β; per second), which is proportional to myocardial blood flow velocity, were calculated automatically by the software. Normalized myocardial acoustic intensity (An) was defined as the ratio of myocardial plateau signal intensity to adjacent left ventricular plateau signal intensity, representing the relative myocardial blood volume. To originate an index of blood flow, the product of An and β (per second) was calculated. Quantitative RTMPE was defined as feasible in the left anterior descending, right, and left circumflex coronary artery territories when at least five of the eight, two of the four, and two of the five segments were analyzable in each territory, respectively. For each study subject, MBFR (An × β), and myocardial blood flow velocity reserve (β) were calculated in each coronary artery territory as the arithmetic mean of values obtained individually in the segments assigned to each coronary artery territory.
Cutoff values of β reserve and MBFR that represent the points of best performance for accurately diagnosing angiographic coronary obstruction have been previously reported in our laboratory and were used in this study to differentiate normal and abnormal β reserve and MBFR. The cutoff values of β reserve and MBFR were 2.0 and 2.2, respectively. These values were used for comparative analysis according to the classification of patients into those with normal coronary arteries, those with significant lesions in one arterial territory, and those with lesions in two or more arterial territories. Intraobserver variability of β reserve and MBFR was assessed in 10 randomly assigned patients, by the same observer, with the two independent measurements made ≥1 year apart.
Follow-Up
Follow-up was obtained by review of patients’ hospital charts and electronic records and telephone interviews with patients. Events were divided into primary and total events. Primary events were defined as cardiac death, nonfatal myocardial infarction, and refractory or unstable angina requiring coronary revascularization during the same hospitalization. Cardiac death was defined as death associated with known or suspected myocardial infarction, life-threatening arrhythmia, or pulmonary edema. Sudden unexpected death occurring without another explanation was included as cardiac death. Nonfatal myocardial infarction was defined by means of a serial increase in cardiac specific enzymes and/or the development of new electrocardiographic changes. Total events were the sum of primary events and secondary events. Secondary events were defined as patients who underwent elective coronary revascularization ≥3 months after RTMPE. Patients who underwent coronary revascularization <3 months after RTMPE were censored at the time of the procedure because the stress echocardiographic results could have influenced the decision to perform revascularization; therefore, these patients were not counted in determining the total number of secondary events. All other patients revascularized after 3 months were censored at the time of revascularization and counted as experiencing “minor events” that were included in the analysis of total events. The date of an event was used to establish the time of follow-up; in the absence of an event, the date of the last interview or of review of medical records was used to determine the time of follow-up.
Statistical Analysis
Data are expressed as mean ± SD for continuous variables and as absolute or relative frequencies for discrete variables. Data normality was tested using the D’Agostino-Pearson omnibus test. Discrete variables were analyzed using χ 2 or Fischer’s exact tests, if necessary. Continuous variables among groups were analyzed using Student’s t tests or analysis of variance (two or three groups, respectively, for variables with normal distribution) and Mann-Whitney or Kruskal-Wallis (two or three groups, respectively, for variables with distributions not considered normal) tests. Kaplan-Meier curves were used to estimate the distribution of time to the events. A Cox model was used to estimate the hazard ratio (HR) of events for each variable. The differences between the event curves were compared using the log-rank test. Clinical variables inserted in the model were age, male sex, ≥ 2 risk factors for CAD (including hypertension, diabetes, cigarette smoking, and family history), stable angina, previous myocardial infarction, and previous percutaneous coronary intervention. Parameters derived from adenosine stress RTMPE included WM (normal or abnormal), qualitative MP (normal or abnormal), β reserve (normal when ≥2.0, abnormal when <2.0), and MBFR (normal when ≥2.2, abnormal when <2.2). Multivariate predictors of events were determined using a Cox proportional-hazards model. The incremental value of real-time MP echocardiographic information was assessed in three modeling steps. The first step consisted of fitting a multivariate model of qualitative analysis of WM only (normal vs abnormal). Qualitative analysis of MP by RTMPE was then added to this model (normal vs abnormal), followed by quantitative analysis of MP using β reserve (<2.0 or ≥2.0) or MBFR (<2.2 or ≥2.2). The significance of adding additional variables to previous modeling steps was based on the change in model-based likelihood statistics, with degrees of freedom equal to the number of additional variables. Intraobserver variability was assessed using coefficients of variation and intraclass correlation coefficients. Two-tailed P values < .05 were considered statistically significant. Statistical tests were performed using SPSS version 13.0 for Windows (SPSS, Inc., Chicago, IL).
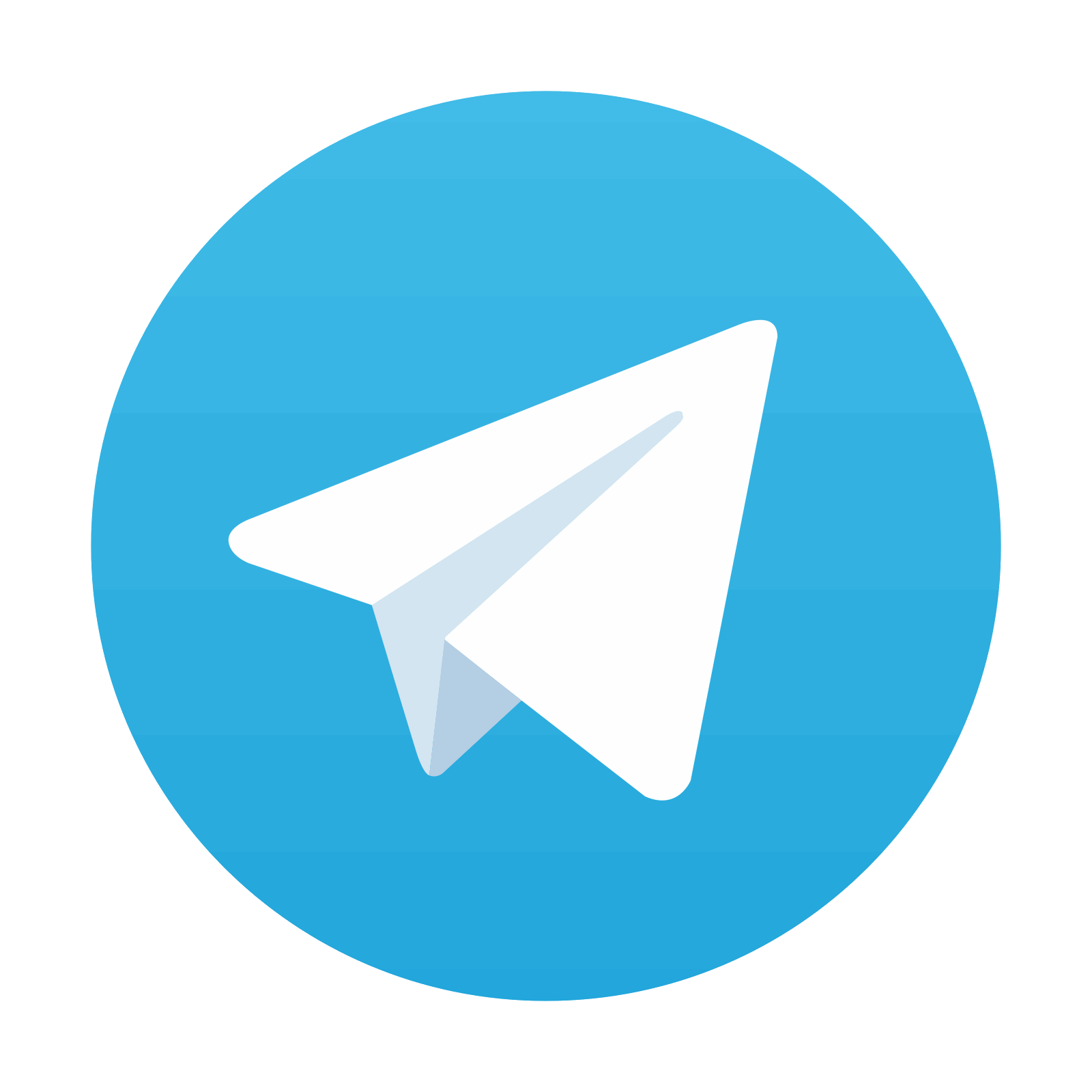
Stay updated, free articles. Join our Telegram channel

Full access? Get Clinical Tree
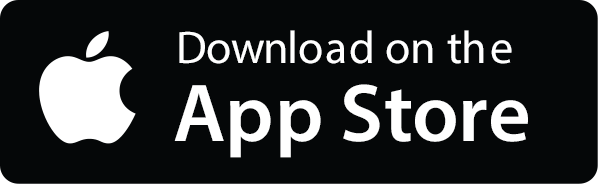
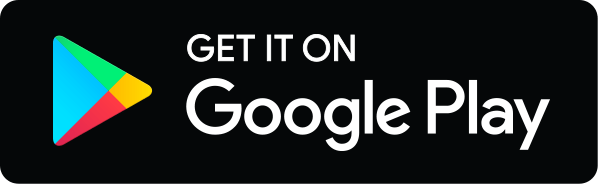