© Springer International Publishing AG 2017
Anna Maria Hibbs and Marianne S. Muhlebach (eds.)Respiratory Outcomes in Preterm InfantsRespiratory Medicine10.1007/978-3-319-48835-6_1The Problem of the Preterm Lung: Definitions, History, and Epidemiology
(1)
Eudowood Division of Pediatric Respiratory Sciences, Johns Hopkins Medical Institutions, David M. Rubenstein Building, 200 North Wolfe Street, Baltimore, MD 21287, USA
Keywords
Bronchopulmonary dysplasiaChronic lung diseasePretermPrematureLung Parenchymal Disease
The archetypal lung disease in preterm infants and one of the more common complications of preterm birth is bronchopulmonary dysplasia (BPD), which may include both parenchymal and small airway components. Common clinical manifestations of BPD include hypoxia, hypercarbia, tachypnea, and asthma-like symptoms [7]. “Classic” or “old” BPD was first described in 1967 by Northway et al. [8], and was characterized by inflammation with airway injury and alveolar fibrosis. Over time, BPD has evolved (“new” BPD), particularly with use of newer ventilation strategies and exogenous surfactant, to a phenotype characterized by fewer and larger simplified alveoli with dysnaptic growth of the pulmonary vascular bed [9, 10]. Although several definitions of BPD or chronic lung disease of prematurity have been used since 1967 [11], currently the most widely agreed upon definition of BPD was developed at a NICHD workshop published in 2001 [12]. At this workshop, the diagnosis and severity of BPD in premature infants were based on gestational age (<32 weeks or ≥32 weeks) and need for oxygen and/or respiratory support at specified time points. This definition was subsequently validated in 2005 [11]. It should be recognized that this oxygen-based definition could overestimate or underestimate the incidence of BPD as goal oxygen saturations remain controversial [13, 14] and can vary among clinical centers [15]. Additionally, this oxygen-based definition may overestimate the incidence of BPD in clinical sites at higher altitudes where the partial pressure of oxygen is decreased [16, 17]. Furthermore, many preterm infants are placed on supplemental oxygen for apnea of prematurity who may or may not have moderate or severe BPD. In addition, these criteria do not define the BPD severity with reference to emerging respiratory support technologies, such as high-flow nasal cannulas.
In comparison to many of the other pulmonary manifestations of prematurity, more data exist on the epidemiology of BPD. Approximately 25 % of infants born with a birth weight of less than 1500 g are diagnosed with BPD [18], although the frequency of BPD reported may vary by center [5, 18]. Assuming that 1.4 % births in the United States annually are born with a birth weight less than 1500 g [4], this extrapolates to 14,000 infants who develop BPD in the United States annually. Although rates for preterm births have declined slightly in recent years [4], rates of BPD may not be mirroring this decline [19].
Infants with BPD frequently experience hypoxemia and many require supplemental oxygen at home, usually delivered via nasal cannula. A validation study of the NICHD BPD criteria in 4866 infants born at less than 32 weeks gestation and weighing less than 1000 g at birth observed that 2.8 % of infants with no or mild BPD were discharged on oxygen, while 64.4 % of those with moderate or severe BPD were discharged to home on supplemental oxygen [11]. These data would suggest that 31.6 % of these extremely low birth weight preterm infants are discharged to home on oxygen. Also, in this validation study, infants with no BPD or mild/moderate BPD were discontinued from home-supplemental oxygen between 7–8 months of age on average, whereas infants with severe BPD were discontinued from oxygen at 9.7 months of age [11].
Infants and children with the most severe BPD resulting in chronic respiratory failure may require tracheostomy placement for long-term ventilation with varying published estimates on the incidence. A 2010 study from Riley Children’s Hospital estimated the incidence to be 4.77 per 100,000 live births with a median duration of home ventilator support of 24 months of age [20], which extrapolates to 400 patients with BPD on home ventilation in the United States at any given time. However, a 2011 study from the Ventilator Assisted Children’s Home Program in Pennsylvania estimated that 8000 children in the United States are receiving home-invasive ventilation, and in this program, 36 % of ventilator-dependent children have chronic lung disease, with 77 % of these diagnosed with BPD [21], which extrapolates approximately 2200 patients with BPD on home ventilation in the United States at any given time. One single site study of 102 infants with BPD requiring home ventilation found an 81.4 % survival rate with 83.1 % of survivors being weaned from ventilation at a median age of 24 months, and 87.0 % of those weaned from ventilation being decannulated at a median age of 37.5 months [20].
Contributing to the difficulty of formulating algorithms for the management of BPD is its multifactorial development. Identified risk factors certainly include gestational age at birth, with infants born at earlier gestational ages experiencing a higher incidence of BPD [19]. Fetal growth also plays a role as infants with lower birth weights corrected for gestational age (fetal growth restriction) have higher rates of BPD [22, 23], and a large Swedish cohort found a 2.7 times higher risk of developing BPD with being born small for gestational age (2 standard deviations below the mean) [24]. Some prenatal risk factors for the development of BPD could include preeclampsia [25, 26], chorioamnionitis [26, 27], and the premature rupture of membranes [24, 28]. However, there remains controversy regarding the role of preeclampsia, as other studies have found preeclampsia to be protective [29] or not a risk factor at all [23, 30]. Likewise, studies of chorioamnionitis as a risk factor have also produced conflicting results [31, 32]. Postnatal risk factors may include patent ductus arteriosus [24, 33, 34] (and again, this is not definitively clear) [35], late-onset infections [24, 34, 36], pneumothorax [24], and mechanical ventilation [24, 34, 37]. In terms of postnatal therapies that may alter the incidence of BPD, retrospective data suggest that prophylactic use of caffeine within the first few days of life may reduce the likelihood of developing BPD [38–40]. However, while antenatal corticosteroids administered to women at risk of preterm birth can accelerate fetal lung maturation [41], neither the use of postnatal systemic or inhaled corticosteroids has been shown to reduce the incidence of BPD [42–45].
In addition, genetic factors may predispose individual infants to develop BPD based on heritability studies of twins [46, 47], but genomewide association studies have not confirmed positive findings from candidate gene studies [48]. Three genomewide association studies have been performed among infants with BPD, and one performed in European and African populations identified SPOCK2, which may have a potential role in alveolar development, to be associated with BPD [49], but two North American studies did not identify any SNPs meeting genomewide significance, including SPOCK2 [50, 51]. Pathway analyses in one of the GWAS studies found an association with BPD and known pathways of lung development and repair (CD44, phosphorus oxygen lyase activity) as well as novel pathways (adenosine deaminase, targets of miR-219) [51]. In addition to genetic factors, an emerging risk factor for the development of BPD may be epigenetic changes (nonnucleotide changes to the genome) that alter gene expression [52]. One study of umbilical cord blood demonstrated that preterm infants that developed BPD had distinct patterns of chromatin remodeling and histone acetylation pathways compared to those who did not (n = 54) [53].
Small Airways Disease
Another frequently seen pulmonary sequelae of preterm infants is small airways disease, which is often secondary to BPD, and can manifest as wheezing and coughing among patients of all ages, and obstructive findings on pulmonary function testing in older children. Computed tomography of the chest in preterm children with a prior history of BPD may reveal emphysema, hyperexpansion, and fibrous/interstitial changes [54].
Objective data regarding the incidence of small airways disease in infants are difficult to capture, as infant pulmonary function testing is only available in a small number of centers and carries the risks attendant to sedating an infant with known respiratory disease. In older children, spirometry can routinely be carried out as a marker of obstructive lung function secondary to small airways disease. A meta-analysis of lung function in former preterm and full-term infants published in 2013 demonstrated that the percent predicted forced expiratory volume in 1 s (FEV1), a commonly used marker of obstructive lung disease, in former preterm infants has been steadily improving in later birth cohorts, although still is approximately 90 % that of former full-term infants [55].
Although the small airways disease associated with prematurity is not necessarily equivalent to asthma, the prototypical obstructive lung disorder in children, the diagnosis of asthma can serve as a marker of small airways disease among preterm infants. Although the risk of asthma is highest in preterm infants born at ≤32 weeks gestation (adjusted OR: 3.9) compared to full-term infants born at 39–40 weeks gestation, even late preterm infants born at 33–36 weeks gestation and early term infants born at 37–38 weeks gestation may be at higher risk as well (adjusted ORs: 1.7 and 1.2, respectively) [56]. It should be noted that the association between asthma and late preterm births has not been consistently observed [57], and that the strength of this association may decrease with age [58]. Nevertheless, given that the prevalence of asthma is 9.5 % among children aged 0–17 years within the general population in the United States [59] and there is an increased odds ratio of asthma of ~3.9 among early preterm infants [56, 58], the prevalence of asthma among these infants may approach 40 %.
Large Airways Disease
Large airways disease in preterm infants is most likely to manifest as tracheomalacia and/or bronchomalacia. Typically, infants with large airway malacia present with respiratory distress (often with abrupt cyanosis), or persistent atelectasis or hyperinflation on imaging [60, 61]. A common clinical scenario of infants with severe large airway malacia may be the onset of sudden respiratory distress and profound desaturations in the setting of agitation or vagal stimulation, and may require use of a bag valve mask for resuscitation.
In terms of the epidemiology of large airway disease in preterm infants, the prevalence is less well characterized and may be underdiagnosed, perhaps due to the difficulties in diagnosis. Classically, direct visualization with bronchoscopy is utilized for diagnosis, which may not be readily available at all NICUs, and may include the attendant risks of sedation and intubation. Newer modalities of imaging, such as CT scanning, may be helpful in the diagnosis of evaluating tracheomalacia [62], but may be only available at select tertiary care institutions and may not be reliable in all patients [61].
One prospective study of 117 preterm infants with a history of intubation for >7 days found that 16.2 % had tracheomalacia, which was associated with severe BPD, hypercarbia, and apnea [63]. Of these patients with moderate to severe tracheomalacia, 21 % required tracheostomies for management.
Vascular Disease
Over the past decade, there has been emerging recognition of pulmonary vascular disease in preterm infants in the form of pulmonary hypertension, which is defined as a resting mean pulmonary artery pressure >25 mm Hg after 2–3 months of age [64]. Pulmonary hypertension in preterm infants is almost always associated with severe lung disease and secondary to a reactive or diminished pulmonary vascular bed, a fact reflected in recent international classifications of pulmonary hypertension [65–67]. However, pulmonary hypertension can also be secondary to cardiovascular lesions; for example, pulmonary vein stenosis has also been reported in preterm infants with pulmonary hypertension and can be associated with poorer outcomes [68, 69]. Although the gold standard for diagnosis of pulmonary hypertension is cardiac catheterization [64], many cases are screened or diagnosed via echocardiograms. Among young children with chronic lung disease, including BPD, echocardiography has been shown to diagnose pulmonary hypertension, but may not be reliable in determining severity [70].
Pulmonary hypertension in preterm infants can manifest as persistent BPD symptoms poorly responsive to therapies or evidence of right heart insufficiency or failure [71]. Also, patients with baseline pulmonary hypertension can experience acute hypertensive crises, which present with an acute increase in work of breathing, hypoxemia, and/or, in the most severe instances, cardiorespiratory failure or arrest [71]. These sometimes life-threatening episodes can be triggered by anesthesia induction [72] or respiratory tract infections [73], possibly through changes in gas exchange leading to hypoxemia or hypercarbia [74–76].
Prospective and retrospective studies have demonstrated a range in prevalence of pulmonary hypertension ranging from 14 to 43 % of preterm infants with BPD [77–82]. Unfortunately, the mortality with patients with pulmonary hypertension remains high, ranging from 14 to 38 % [77–82]. In addition, these patients also experience additional morbidity in the form of longer initial hospital stays, with infants with pulmonary hypertension having a 2.2 month longer initial NICU admission compared to infants with BPD without pulmonary hypertension, which may result in at least an additional $198,000 in health care expenditures [83].
Pulmonary hypertension likely arises from a series of complex interactions between multiple factors, including genetic and prenatal/postnatal environmental factors [84]. These factors may include alterations in angiogenic pathways [85–88], possible genetic modifiers [46, 89], intrauterine growth [78, 81, 90–92], oligohydramnios [79, 92], patent ductus arteriosus [77], and placental vascularity [93, 94]. Although most cases of pulmonary hypertension arise as a function of reactive or diminished pulmonary vascular bed, pulmonary hypertension can occur as a function of pulmonary vein stenosis [68, 69], for which umbilical catheters may be a risk factor [95].
Control of Breathing
Infants born prematurely frequently demonstrate issues with immature control of breathing manifesting as central apneas. These apneas, if prolonged, can be accompanied by bradycardia and oxygen desaturations. The oxygen desaturations may be more prominent in infants with poor pulmonary reserve (i.e., parenchymal disease) and can occur with brief central apneas that last only a few seconds [96]. In addition, preterm infants with BPD may have blunted chemoreflex responses to both hypoxia [97] and/or hyperoxia [98]. Limited data suggest that infants with a history of posthemorrhagic hydrocephalus are more likely to have apneic events [99, 100], and certainly untreated or inadequately treated elevated intracranial pressures from hydrocephalus may cause bradypnea or apnea.
Although central apneas may be isolated events, they also may occur in rapid successive sequence with periodic breathing. Periodic breathing is commonly observed in infants, including preterm infants, but may frequently lead to oxygen desaturations in preterm infants [101]. The incidence of disordered control of breathing is likely underestimated, as the use of supplemental oxygen or augmented airflow may also mask control of breathing abnormalities [102]. Nevertheless, the frequency of such events and time to resolution are associated with gestational age at birth with infants born earlier at a higher risk of demonstrating apnea and a later age of resolution [103, 104].
Data from apnea monitor downloads suggest that preterm infants may be at a risk of more extreme events until 43 weeks postconceptional age [105]. Overnight polysomnography data obtained on infants and toddlers born prematurely also demonstrate a decrease in central apneas after 12 months of age [96]. Although there are no published data regarding sleep-disordered breathing in adults born prematurely, alterations in ventilatory control, including both hypoventilation and hyperventilation, in exercising children and adults born prematurely have been observed compared to term controls [106] as well as in adults born prematurely exposed to hypoxic and hyperoxic conditions [107].
Upper Airway Obstruction
Upper airway obstruction leading to obstructive apneas or hypopneas can occur in several anatomic locations in preterm infants. During infancy, obstruction in the palatal or retroglossal regions may be more common, in addition to laryngeal lesions such as vocal cord paresis and subglottic stenosis, whereas in toddlers, anatomic obstruction is more likely to be secondary to adenotonsillar hypertrophy [108]. In addition, a relatively larger tongue size for preterm infants has been postulated to contribute to narrowing of the upper airway based on respiration-timed radiographs [109]. Although the gold standard for diagnosis of upper airway obstruction is overnight polysomnography with specialist evaluation [110], specific diagnoses are often made by direct visualization by an otolaryngologist.
The risk factors and prevalence for upper airway obstruction in preterm infants are dependent on the site of the obstruction. Acquired subglottic stenosis is often associated with intubation, and pertinent risk factors may include duration of intubation, endotracheal tube size, and the occurrence of traumatic intubation [111]. Acquired subglottic cysts may also be more likely to occur with traumatic or prolonged intubation [112, 113]. In at least one prospective study, subglottic cysts were identified in 7.2 % of preterm infants after prolonged intubation (n = 153) [113]. Although it can be postulated that gastroesophageal reflux (which is not uncommon in preterm infants) could be associated with obstructive apnea through upper airway inflammation or laryngeal chemoreflexes [114], the association between reflux and apnea is not well established in infants, regardless of prematurity [115, 116]. Vocal cord paresis may occur as a complication of cardiac surgeries, including PDA ligation. In one meta-analysis, the weighted pooled proportion of unilateral vocal fold paralysis in studies that assessed the vocal folds postoperatively was 29.8 % for any congenital cardiac surgery (11 studies; n = 584) and 39 % for patent ductus arteriosus ligation (6 studies; n = 274) [117].
Although there are limited data regarding the prevalence of sleep-disordered breathing among former preterm infants, a US-based population cohort study of 850 children aged 8–11 years, of whom 46 % were born preterm, found that sleep-disordered breathing was 3–5 times more common among those born prematurely compared to those born at term [118]. Other retrospective chart reviews have also found an association between prematurity and obstructive sleep apnea [119].
Conclusions
Preterm infants can manifest a variety of respiratory complications, which providers who care for preterm infants should be aware of. The diverse elements of the respiratory tract that may be involved and the varying severity of presentations make it difficult to apply common algorithms for prevention and management. Further research is necessary to more clearly delineate the scope of disease as well as best practices for diagnosis and treatment.
References
1.
Stoll BJ, Hansen NI, Bell EF, Walsh MC, Carlo WA, Shankaran S, et al. Trends in care practices, morbidity, and mortality of extremely preterm neonates, 1993-2012. JAMA. 2015;314(10):1039–51. PubMed PMID: 26348753.PubMedPubMedCentral
2.
Liu L, Oza S, Hogan D, Perin J, Rudan I, Lawn JE, et al. Global, regional, and national causes of child mortality in 2000-13, with projections to inform post-2015 priorities: an updated systematic analysis. Lancet. 2015;385(9966):430–40. PubMed PMID: 25280870.PubMed
3.
Martin JA, Hamilton BE, Osterman MJ. Births in the United States, 2014. NCHS Data Brief. 2015;216:1–8. PubMed PMID: 26460599.
4.
Hamilton BE, Martin JA, Osterman MJ, Curtin SC, Matthews TJ. Births: final data for 2014. National vital statistics reports: from the Centers for Disease Control and Prevention, National Center for Health Statistics. Natl Vital Stat Syst. 2015;64(12):1–64. PubMed PMID: 26727629.
5.
Guaman MC, Gien J, Baker CD, Zhang H, Austin ED, Collaco JM. Point prevalence, clinical characteristics, and treatment variation for infants with severe bronchopulmonary dysplasia. Am J Perinatol. 2015;32(10):960–7. PubMed PMID: 25738785. Pubmed Central PMCID: 4617756.PubMedPubMedCentral
6.
Lapcharoensap W, Gage SC, Kan P, Profit J, Shaw GM, Gould JB, et al. Hospital variation and risk factors for bronchopulmonary dysplasia in a population-based cohort. JAMA Pediatr. 2015;169(2):e143676. PubMed PMID: 25642906.PubMed
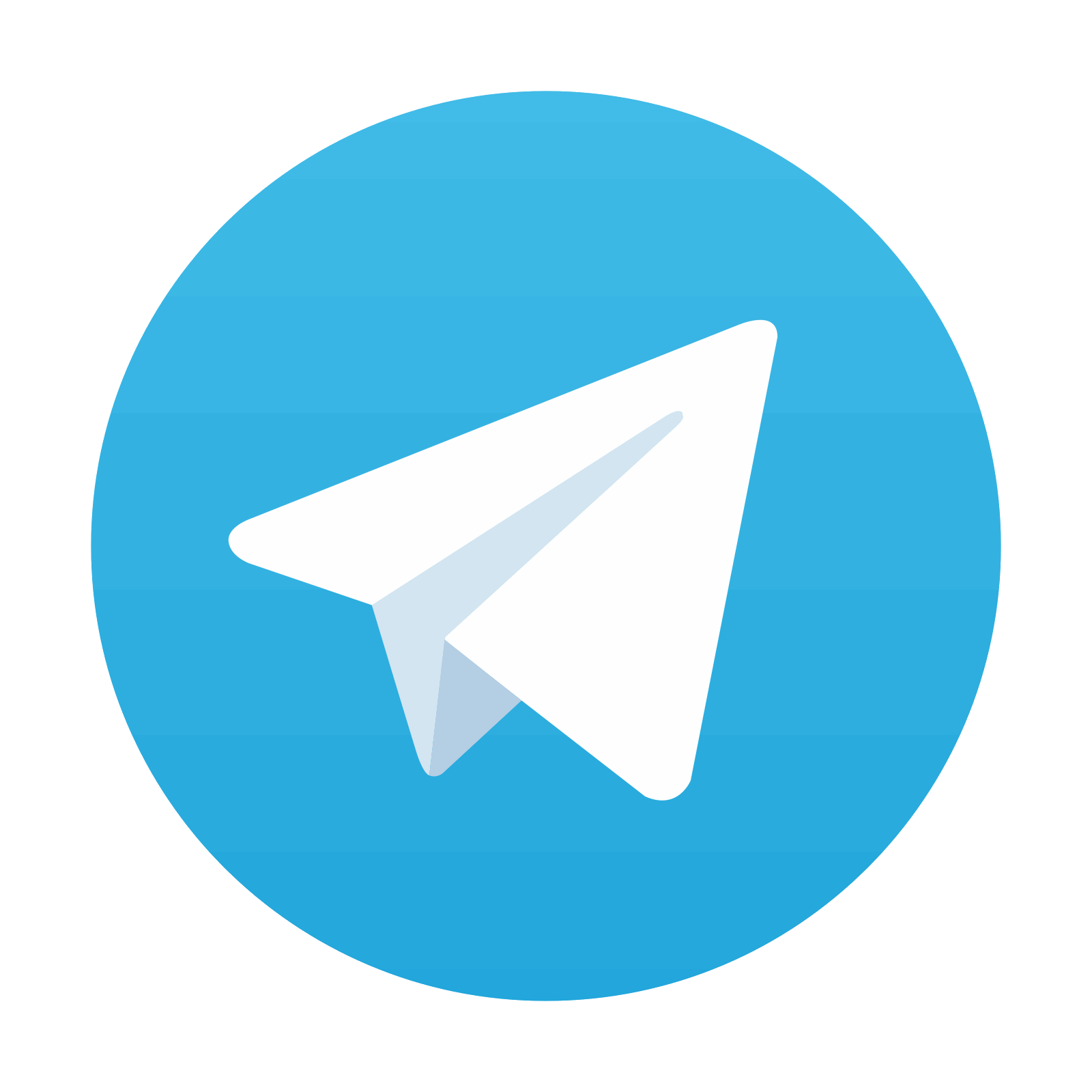
Stay updated, free articles. Join our Telegram channel

Full access? Get Clinical Tree
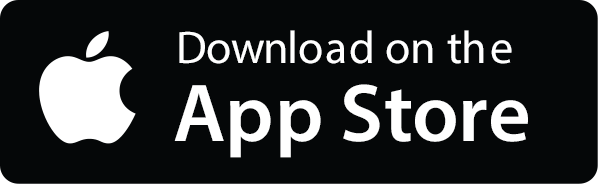
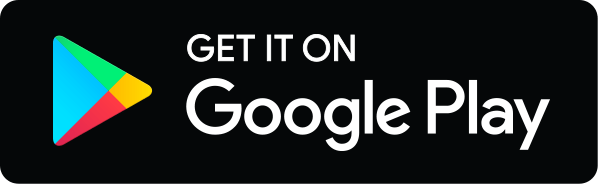
