Barbara J. Deal1, Amanda L. Hauck1, Sabrina Tsao2, Angira Patel1, Simon Lee3, and Doff B. McElhinney4 1Ann & Robert H. Lurie Children’s Hospital of Chicago, Chicago, IL, USA 2Queen Mary Hospital, University of Hong Kong, Hong Kong 3Nationwide Children’s, Columbus, OH, USA 4Lucille Packard Children’s Hospital, Stanford University, Palo Alto, CA, USA Preoperative evaluation of a patient with congenital heart disease (CHD) requires an understanding of the anatomy and physiology of the lesion, associated cardiac and systemic anomalies, prior surgeries, and rhythm concerns. The goals of the preoperative evaluation are to optimize survival of the patient, which encompasses minimizing unexpected anatomic findings in the operating room, preventing perioperative complications as much as is feasible, and ultimately improving subsequent quality of life for the patient. The quality of life depends on the quality of anatomic repair as well as preservation of heart, brain, and organ system function, and efforts to minimize long‐term arrhythmias and heart failure, which are the major late causes of morbidity and mortality following surgery. Although survival following repairs of CHD in infancy has improved dramatically in the last two decades, late neurodevelopmental disabilities are increasingly recognized [1–3]. Increased efforts to improve perioperative morbidity and neurodevelopmental outcomes are imperative and encompass many aspects of care [4, 5]. Accuracy of preoperative anatomic diagnosis and optimization of preoperative hemodynamic status are essential to improve surgical outcomes. In addition to a thorough assessment of anatomic variables, optimization of outcomes requires evaluation of extracardiac and genetic concerns, analysis of arrhythmias, conduction disorders, and chronotropic competence, and evaluation of ventricular function. Patient‐specific variables in addition to complexity of heart disease that negatively impact survival include prematurity, low birth weight, extracardiac and/or genetic abnormalities, race, sex, and presence of preoperative renal dysfunction, shock, and mechanical ventilation [6–9]. Center‐specific variables that impact survival after primary cardiac repairs include center volume of congenital heart surgeries, complexity of case mix, and volume of neonatal cardiac repairs [10, 11]. The quality of congenital heart surgery impacts the length of stay, with increased length of stay independently correlated with four variables: revision of primary repair, diaphragm paralysis, postoperative complete heart block requiring a permanent pacemaker, and unplanned postoperative cardiac catheterization [12]. The surgeon‐specific variable of technical performance skill is paramount in improving postoperative morbidity and neurodevelopmental outcomes [4, 13–17]. Cardiac surgeries in young patients generally fall into a series of distinct age groups: the neonate with cyanotic or obstructive lesions, the older infant and younger child with predominantly left‐to‐right shunting defects, and the increasing number of reoperations for conduit or valve changes or residual lesions, including in adult patients with CHD (Tables 4.1–4.3). Advances in imaging have improved anatomic visualization of all lesions, and have limited the need for invasive studies in most situations, but create choices for selecting the optimal imaging modality for each patient. Physical examination findings, characteristic electrocardiographic findings, catheterization data relevant to specific cardiac lesions, and timing and indications for surgery have been detailed in textbooks of pediatric cardiology and review articles [18]. This chapter will focus on specific concerns for evaluating patients in each of the age groups, taking into account the alternatives of catheter‐based interventions and opportunities for rhythm optimization. Table 4.1 Most common cardiac surgeries in first year of life from the STS Congenital Heart Surgery Database 2012–2015. AV, atrioventricular; TOF, tetralogy of Fallot; VSD, ventricular septal defect. Source: [370] / with permission of Elsevier. Table 4.2 Common cardiac surgeries in the older infant or child: Anatomic characteristics. ASD, atrial septal defect; CT, computed tomography; L, looping; MRI, magnetic resonance imaging; PAPVC, partial anomalous pulmonary venous connection; PFO, patent foramen ovale; RV, right ventricle; TGA, transposition of the great arteries; TOF, tetralogy of Fallot; TV, tricuspid valve; VSD, ventricular septal defect. Table 4.3 Cardiac reoperations in children and adults. CMR, cardiac magnetic resonance; CT, computed tomography; MR, magnetic resonance. The recognition and evaluation of extracardiac anomalies in a neonate with CHD are critically important for assessment of cardiac surgical risk and prognosis, and appropriate counseling of families. The presence of syndromic, genetic, and noncardiac abnormalities can significantly negatively impact outcomes in infants with CHD, with increased hospital stay, in‐hospital mortality, unplanned reoperations, and diminished late survival (Table 4.4) [19–26]. Earlier studies estimated the overall prevalence of extracardiac abnormalities in CHD at 25–30%, while more recent meta‐analyses and population‐based studies reported a prevalence of associated anomalies in the range of 17–28%, which may reflect the more recent increase in terminations of pregnancy [26–31]. A contemporary, multicenter analysis of only neonates showed a prevalence of 18.8% for noncardiac, syndromic, or genetic abnormalities [32]. Understanding which specific abnormalities coexist with specific types of CHD has been challenging and is an important area of emerging research. Changes in screening practices and advances in diagnostic precision will enable identification of genetic abnormalities in a larger fraction of neonates with CHD [33]. A multifactorial hypothesis for the etiology of CHD including genetic and environmental factors has long been recognized. Significant progress has been made in understanding the genetics of CHD, including identification of specific gene abnormalities associated with certain types of malformations [33–35]. The recent advances in elucidating the genetics of cardiac morphogenesis improve our understanding of etiology and pathogenesis of CHD, providing information for families and estimation of recurrence risks [36, 37]. The impact of a genetic mutation on protein function may alter lifelong cardiac regulation as well as the function of other organ systems contributing to morbidity, which was previously poorly understood. Examples include gene mutations altering ciliary function, chromatin, or transcription factor regulation, which may contribute to pulmonary dysfunction in heterotaxy syndrome; impaired neurodevelopmental outcomes affecting more than 10% of CHD patients; late ventricular dysfunction associated with sarcomere mutations; and progressive conduction disease associated with familial septal defects, such as the homeobox transcription gene, NKX2.5 [38]. The 2007 American Heart Association (AHA) Scientific Statement on the Genetic Basis for Congenital Heart Defects outlined several important reasons to determine genetic causes of CHD: to identify other organ systems that might be involved and require surveillance, determine and better define prognosis, inform families about recurrence risk, and identify at‐risk family members and provide necessary family screening, including genetic testing [39]. This statement recommended cytogenetic testing in patients and families with the following scenarios related to CHD: (i) major cardiac visceral organ malformations detected by prenatal ultrasound and/or fetal echocardiography; (ii) dysmorphic features, growth retardation, or multiple congenital anomalies; (iii) recognizable chromosomal syndrome; (iv) offspring of parents with multiple prior miscarriages and/or siblings with birth defects; and (v) children with developmental delay/intellectual disability. In addition, genetic evaluation should be performed in patients with CHD lesions associated with a high prevalence of 22q11 deletion syndrome: tetralogy of Fallot (TOF), interrupted aortic arch, common arterial trunk, ventricular septal defect (VSD) with aortic arch abnormality, isolated aortic arch anomaly, or discontinuous pulmonary arteries [39]. Other societies have suggested additional indications for genetic testing, including for family history of one or more CHD in first‐degree relatives, patients with outflow tract obstructive lesions, heterotaxy syndrome, and for older patients of reproductive age who might not have undergone testing earlier in life and/or are interested in genetic evaluation [40, 41]. Table 4.4 Common cardiac surgeries in infancy: Anatomic characteristics. ASD, atrial septal defect; AV, aortic valve; CT, computed tomography; d‐TGA, d‐transposition of the great arteries; LV, left ventricle; MRI, magnetic resonance imaging; PDA, patent arterial duct; PV, pulmonary valve; RV, right ventricle; TV, tricuspid valve; VSD, ventricular septal defect. The increased availability of genetic testing and assessment of extracardiac abnormalities provides an opportunity to assess their impact on surgical outcomes using large databases such as those from the European Association of Cardiothoracic Surgery and the Society for Thoracic Surgery Congenital Heart Surgery [22, 42]. Incorporation of these data enables more accurate preoperative counseling, and more realistic expectations of morbidity as well as mortality, and forms the basis for improving informed consent to surgery [43, 44]. Infants with Down syndrome undergoing cardiac surgery have similar operative mortality to patients without Down syndrome, but experience prolonged length of stay, increased respiratory and infectious complications, and are more likely to require pacemaker implantation [42]. For infants with conotruncal defects, both the presence of 22q11 deletion and syndromic increase surgical mortality (26% versus 11% and 18% versus 10%, respectively) [20, 21, 45]. The presence of 22q11.2 deletion in pulmonary atresia and intact ventricular septum is a significant risk factor for in‐hospital and late mortality [19, 23]. In hypoplastic left heart syndrome (HLHS), the presence of any syndromic, genetic, or noncardiac abnormality increases stage 1 mortality by 30% (26% versus 20%), and the presence of a chromosomal defect more than doubles the early risk of death [22]. For neonates undergoing the Norwood operation, independent risk factors for 30‐day hospital mortality, postoperative renal failure, sepsis, length of ventilation, and hospital stay are increased with the presence of a genetic abnormality [24, 46]. Specifically related to HLHS and concomitant diagnosis of Turner syndrome, the mortality is especially high (neonatal morality 36% versus 27%), with long‐term mortality approaching 91% [47]. Some have advocated for more rigorous and perhaps universal screening in all patients with CHD [48]. However, given that genetic screening can produce nonclinically significant results and may impose financial or psychological burdens, further work is likely needed prior to advocating for universal screening. In the meantime, heightened focus should remain on the above‐listed types of CHD and associated genetic anomalies when counseling families regarding surgical risks and prognosis. Appropriate assessment of rhythm and conduction abnormalities includes review of electrocardiograms (ECG) and clinical arrhythmias, which may include the need for 24‐hour rhythm monitors, month‐long event monitors, and potentially intracardiac electrophysiology study and preoperative ablation of arrhythmia substrate when indicated. Open surgical exposure of the heart presents a unique opportunity to modify long‐term chronotropic, rhythm, conduction, and contractility parameters; some of these may be prophylactic in nature. After review of all rhythm and conduction considerations, appropriate recommendations for potential surgical intervention for arrhythmias and rhythm optimization can be made. In some circumstances, this may include recommendations for atrial pacing to optimize atrioventricular synchrony, placement of epicardial leads due to an anticipated high risk of conduction abnormalities, atrial arrhythmia surgery, ventricular resynchronization to improve ventricular function, and/or possible defibrillator implantation at the time of surgery. The preoperative ECG in the neonate may raise suspicion of heart disease as a screening tool, and may be supportive in lesion diagnosis, but is not a major diagnostic resource. The value of the preoperative ECG is to identify potential rhythm or conduction abnormalities including pre‐excitation, assess hypertrophy, and serve as a baseline for recognizing acute and chronic postoperative changes. Progression of ventricular hypertrophy acutely postoperatively may indicate inadequate anatomic repair, or raise suspicion of associated cardiomyopathy. Acute postsurgical ischemic changes may occur following complex surgeries such as the Norwood procedure for HLHS, arterial switch operation, or anomalous coronary artery surgery, which may cause ischemic changes postoperatively. The postoperative ECG may signal acute ST elevations in comparison to preoperative ECG, prompting early intervention prior to severe hemodynamic compromise. The recognition of a change in rhythm such as the development of atrial tachycardia with variable conduction may be subtle and becomes apparent when a 12‐lead ECG is compared to the preoperative study (Figure 4.1). In infants, the presence of heterotaxy syndrome may be suspected by the presence of two different sinus P waves in the setting of right atrial isomerism, or the absence of sinus rhythm with left atrial isomerism. In addition, patients with heterotaxy syndrome may have twin atrioventricular (AV) nodes noted as alternating QRS axes on ECG or monitoring. AV conduction abnormalities are unusual in the neonate with structural heart disease, but may be present in patients with heterotaxy syndrome or congenitally corrected transposition of the great arteries. Pre‐excitation is more common in patients with Ebstein anomaly and congenitally corrected transposition of the great arteries, and is a substrate for the development of supraventricular tachycardia. Outside of the neonatal period, the presence of pre‐excitation preoperatively is generally a recommendation for electrophysiology study and preoperative ablation, as indicated in patients with Ebstein anomaly [49, 50]. Figure 4.1 Electrocardioigram (ECG) from a neonate with Ebstein anomaly. (A) Sinus rhythm with marked right atrial enlargement and incomplete right bundle branch block. (B) Postoperatively, note irregular rhythm with flutter waves visible in leads II, aVF indicating variable atrioventricular conduction. Sinus node dysfunction may be present in patients who have undergone extensive atrial surgery (Mustard–Senning or Fontan operation) or postsinus venosus atrial septal defect (ASD) repair. In older patients undergoing reoperations, 24‐hour ambulatory electrocardiographic monitoring is useful to assess sinus bradycardia, intermittent conduction abnormalities, and chronotropic competence with exertion. Patients with prior surgical closure of ASD or VSD have abnormal heart rate responses to exercise, and require surveillance for this problem [51]. Atrial reentrant tachycardia develops in 18–60% or more of patients who have undergone the Fontan operation, Mustard–Senning operation, or TOF repair [49, 52–54]. As many as one‐third of children undergoing cardiac surgery have preoperative arrhythmias, particularly in infants who are less than one month of age, and arrhythmias are increased in those receiving milrinone postoperatively [55]. Recent consensus statements and guidelines recommend that patients with preoperative atrial fibrillation or atrial re‐entry tachycardia undergo concurrent arrhythmia surgery at the time of operative interventions [49, 50, 56–58]. AV nodal conduction abnormalities may develop in patients with congenitally corrected transposition of the great arteries, mitral or tricuspid valve replacements, aortic valve replacement, VSD closure, or Ross–Konno surgery [59–62]. Postoperative ECG may reveal new conduction abnormalities, such as left or right axis deviation, and first or second‐degree AV block. Right bundle branch block (RBBB) has been reported in 70–90% of patients undergoing TOF repair historically, and in 60–80% of patients undergoing surgery for VSD closure [60, 61]. Bifascicular block (RBBB plus left anterior hemiblock) was reported among 8–23% of patients with TOF, and these patients are at risk for developing late complete AV block [63]. Despite improvements in postoperative survival in the last several decades, the incidence of postsurgical complete AV block remains relatively constant at 4–7%, and is associated with longer postoperative stays, higher hospital costs, and increased late mortality when implantation of a permanent pacemaker is needed [59, 61, 62, 64]. The risk of complete heart block is highest in infants operated on under one month of age, as well as with longer cardiopulmonary bypass runs/aortic cross‐clamp times, and occurs most commonly following double‐switch repairs and complete repairs of TOF, complete AV septal defect, and VSD [59–61, 65]. In most cases of CHD, a complete anatomic diagnosis can be fully discerned using transthoracic two‐dimensional echocardiography as the sole imaging modality [66–70]. Complete preoperative echocardiographic assessment of CHD should be performed at laboratories with sonographers and physicians experienced in the anatomy of complex heart disease to improve diagnostic accuracy and ascertain details important to surgical planning [71, 72]. Specific details of arch anatomy, coronary arteries, pulmonary venous return, and branch pulmonary arteries may require additional imaging modalities to optimize surgical planning [73–78]. Most pediatric centers routinely employ transesophageal echocardiography (TEE) in the operating room, to assess subtleties of the preoperative anatomy and adequacy of the repair prior to the conclusion of surgery. Adolescents and adults with CHD often did not have optimal transthoracic imaging, may have complex prior repairs including conduits, and may benefit from advanced multimodality imaging [73, 79–82]. Three‐dimensional (3D) echocardiography may add important anatomic detail of valve anatomy and orientation of great arteries for the surgeon as an adjunct to 2D echocardiography [83–86]. For atrioventricular septal defects (AVSDs) and mitral valve disease, 3D echocardiography can provide details of valve morphology, leaflet attachments, and the mechanism of regurgitation [87, 88]. Use of 3D transthoracic echocardiography during transcatheter device closure of ASDs or VSDs may provide accurate defect sizing and en face visualization of the defect [89–93]. Single‐beat 3D echocardiography provides more complete quantification of right ventricular function and estimation of pulmonary hypertension when compared with conventional echocardiography [94]. Cardiac magnetic resonance imaging (MRI) may provide anatomic and hemodynamic information on complex cardiac anomalies not available by echocardiography or catheterization and useful for operative planning; excellent recent guidelines and summaries of indications have been published [95–100]. In infants, when avoidance of cardiac catheterization is desirable, MRI performed in the first week of life provided additional significant findings in more than 65% of patients, which altered surgical management in 50% of infants [101]. Cardiac MRI provides detailed cardiac valve anatomy, and may quantify ventricular size, mass and function, hemodynamic flow, myocardial perfusion, and tissue characterization, as well as imaging extracardiac anatomy. The MRI data can be obtained as a 3D “isotropic” dataset, allowing multiplanar reformatting, 3D reconstruction, and model printing, which may be beneficial for operative planning and education of surgical trainees, as well as an illustrative tool for families [102]. In cases of complex extracardiac anatomy with multiple sources of pulmonary blood flow and complex aortic arch anatomy, both cardiac computed tomography (CT) and MRI can add to diagnostic accuracy of structures that are difficult to see by transthoracic echocardiography (arch vessels, coronary arteries, peripheral pulmonary arteries, anomalous pulmonary venous return) [103, 104]. Cardiac MRI is particularly useful for preoperative assessment of patients with single‐ventricle anatomy to assess pulmonary arterial size and systemic‐to‐pulmonary collateral flow, and in post‐Fontan procedure to assess flow within the Fontan pathway [105–109]. Assessment of ventricular size, mass, and scar with cardiac MRI provides important detail in patients who have undergone repair of TOF, and the degree of ventricular myocardial fibrosis may help prognosticate subsequent arrhythmic risk [110–113]. MRI of peripheral pulmonary artery size and relative flows may be incorporated into catheterization procedures, thus reducing the need for additional angiography and radiation [104, 114, 115]. Brain MRI scanning of neonates with cyanotic heart disease is increasingly used to understand the contributions to neurodevelopmental problems later in life. In infants with transposition of the great arteries and single‐ventricle anatomy, preoperative brain MRI has demonstrated disturbances in cerebral perfusion and reduction in brain volumes compared with healthy controls [116–121]. White‐matter injury to the brain has been demonstrated in 20% of neonates with CHD preoperatively, with new white‐matter injury postoperatively in 44%, with or without the use of cardiopulmonary bypass [122]. Adolescents with transposition of the great arteries showed white‐matter fractional anisotropy on brain MR scanning, which may relate to neurodevelopmental deficits [123]. Neuroimaging, cardiovascular physiology, and functional outcomes are a major source of ongoing neonatal research in efforts to optimize neurodevelopmental outcomes [120, 124–127]. In patients with univentricular physiology, abnormal liver architecture is demonstrated by ultrasound and MRI pre‐ and postoperatively, with elevated liver stiffness detected by 1–5 years post Fontan surgery [128–132]. Ideally, patients with Fontan procedures should undergo liver evaluation prior to reoperations beyond early childhood, and annually as adults [133, 134]. Liver function abnormalities identified in Fontan patients are usually mild elevation of bilirubin and gamma glutamyl transferase, and thrombocytopenia, with otherwise normal liver function tests [135]. MRI can identify hepatic abnormalities in Fontan patients compatible with fibrosis and congestion, which may be undetected by laboratory testing [132, 136]. Due to the increased risk of hepatocellular carcinoma in Fontan patients, liver screening is important to allow successful cryoablation therapy in early stages of cancer with small isolated lesions [137–140]. Although cardiac MRI provides excellent anatomic and functional data without exposure to ionizing radiation, important considerations include the need for sedation or general anesthesia in younger patients. Artifacts from metallic objects (e.g., vascular coils, stents, implanted pacing devices) can limit safety and optimal imaging, and the safety of gadolinium or contrast exposure [141]. The presence of pacemakers or intracardiac defibrillators is a restriction but not absolute contraindication to MRI, due to lead heating, inappropriate defibrillator shock or pacing, and device reprogramming or loss of pacing; careful risk/benefit assessment and collaboration with electrophysiologists for device reprogramming is recommended [142, 143]. Some newer devices and transvenous leads are compatible with MRI; recent data suggest that scanning may be safer than previously reported with appropriate planning and device reprogramming [143–147]. Although there is no exposure to ionizing radiation during MRI, questions regarding the long‐term effects of general anesthesia and gadolinium remain unanswered. Advances in CT scanner technology allow rapid visualization of large anatomic volumes during a single heartbeat, with submillimeter spatial resolution providing diagnostic images of small cardiovascular structures without sedation or anesthesia, a significant advantage for complex CHD [74, 76, 148, 149]. Although the need for anesthesia is much less frequent than in cardiac MRI, imaging over multiple heartbeats or an inability to remain motionless can necessitate a brief period of sedation. Image quality is not as affected by the presence of stents or coils, and imaging can be performed in the presence of a pacemaker or intracardiac defibrillator. CT carries the risk of exposure to ionizing radiation, which is particularly relevant to small and young patients with CHD and repeated imaging requirements [150]. CT is optimal for imaging small vascular structures including coronary artery anomalies or aneurysms, as well as thoracic vasculature abnormalities, including vascular rings and anomalous pulmonary venous drainage [149, 151]. Although MRI is the optimal advanced imaging modality for patients with Fontan repairs or repaired TOF with pulmonary artery dilatation and conduits, patients with implanted devices or bronchial compression may be referred for CT [74]. CT provides excellent imaging for patients with pulmonary atresia to assess sources of pulmonary flow, and with right ventricle (RV)‐dependent coronary circulation (Figure 4.2) [152]. Atrial baffle obstruction in patients with transposition of the great arteries and prior Mustard or Senning procedures can be well visualized with CT, which may also be useful to guide transvenous lead placement or catheter ablation in these patients [153]. Proximity of coronary arteries, conduits, or an enlarged right atrium to the sternum is a concern for reoperations, and either cardiac MR or CT scan images show this relationship clearly. CT provides excellent visualization of noncardiac structures, such as the lung parenchyma, airway, liver, and skeletal anatomy, and is useful in the evaluation of liver parenchyma in older Fontan patients [148, 154]. Limitations of CT include the exposure to ionizing radiation (which may be multiple), the need for iodinated contrast in every study, and its limited ability for functional and hemodynamic evaluation [148]. Although strides have been made in radiation dose reduction, CT is associated with a “nonnegligible” risk of malignancy [150]. With increased life expectancy, patients with CHD are exposed to multiple diagnostic noninvasive imaging procedures, cardiac catheterization, angiography and interventional procedures, and device implantations, resulting in a significant lifetime increase in exposure to low‐dose ionizing radiation [155, 156]. Exposure to ionizing radiation is occurring at progressively younger ages in patients with CHD born since 1995, and with significantly greater frequency, with highest exposures among patients with complex disease [157]. Overall, the lifetime risk of developing cancer is increased in patients with CHD, particularly breast, colon, prostate, and liver cancers, which is likely multifactorial, including cumulative exposures to radiation and anesthesia, cardiac cirrhosis, and genetic programming [137, 158–160]. Ethical questions of informed consent in CHD are many and complex, but patient autonomy is an increasingly relevant concern; to these questions must be added an analysis of the risk of radiation from diagnostic imaging [43, 161, 162]. Figure 4.2 Pulmonary atresia with ventricular septal defect, 3D volume‐rendered reconstruction of computed tomography (CT). Note the diminutive confluent mediastinal branch pulmonary arteries (solid arrow) and major aorto‐pulmonary collateral arteries (dashed arrow) arising from the descending thoracic aorta (Ao). 3D volume‐rendered reconstructions such as this can be helpful in delineating the collateral vessels prior to surgical planning. Predischarge transthoracic echocardiography (TTE) following congenital heart surgery is performed in virtually all patients to assess for residual lesions, ventricular dysfunction, and pericardial effusion, with multiple studies obtained in 23% of patients in a recent study [163, 164]. The need for multiple postoperative studies has been correlated with increased complexity of heart disease, with new abnormal finds detected in approximately 3% of patients requiring escalation of care [164]. A recent retrospective study found that residual obstruction, greater than mild valve regurgitation or ventricular dysfunction, was associated with increased need for hospitalization or reintervention at one year, controlling for complexity of procedure [163]. Additional investigation into the indications for predischarge echocardiograms and their cost‐effectiveness, especially after low‐complexity procedures, is ongoing [164]. With the advancement of noninvasive imaging technologies, the role of cardiac catheterization in the preoperative evaluation of patients with CHD continues to evolve (Table 4.5). Anatomic evaluation in the catheterization lab consists of standard single‐plane or biplane angiography, and more recently 3D angiographic techniques that are similar to CT. The foundations of physiologic evaluation in the catheterization lab are direct measurement of intracardiac and vascular pressures, and measurement of oxygen saturations to facilitate estimation of blood flows and shunts. There are a number of pharmacologic and mechanical manipulations that can be employed to aid in surgical planning or decision‐making (Table 4.1). In surgical candidates, indications for preoperative catheterization can be considered conceptually as (i) assessment to determine whether surgical intervention is indicated; (ii) assessment to understand physiology and/or anatomy in anticipation of surgical therapy; or (iii) assessment to aid in surgical planning. Examples of the types of catheterization‐derived data that may serve these aims are summarized in Table 4.2. Table 4.5 Preoperative cardiac catheterization goals. AV, aortic valve; HLHS, hypoplastic left heart syndrome; RV, right ventricle. For most forms of CHD, preoperative catheterization is not performed routinely, but for some lesions and operations invasive assessment and/or angiography remains standard. Catheterization provides options for anatomic evaluation, assessment of physiology, and selective angiography of cardiovascular structures, which are complementary to noninvasive imaging. The utility and importance of these parameters in preoperative evaluation vary among congenital heart defects, and may also vary among clinicians. There are few circumstances in which angiography is uniquely able to delineate anatomy, compared with other noninvasive imaging modalities, but in some cases familiarity with and accessibility of angiographic images may be of sufficient value to recommend this approach over, or in addition to, other methods. There are certain cardiac anomalies that may currently require diagnostic catheterization in the neonatal period. Coronary anomalies can be identified by echocardiography, but may not be fully characterized [165, 166]. Even in neonates who are clinically stable, characterization of the coronary and pulmonary anatomy in the neonatal period is important to plan and sometimes initiate surgical management [167–169]. In both pulmonary atresia with intact ventricular septum and HLHS with mitral stenosis/aortic atresia, connections between the coronary artery system and the right or left ventricle, respectively, sometimes occur and can have important implications for therapy (Figure 4.3) [166, 170–175]. Therefore, many patients with these anomalies should undergo catheterization before surgery in order to characterize the extent of ventricle–coronary connections [173]. Figure 4.3 These right ventricular angiograms from the frontal (left) and lateral (right) projections in a newborn with pulmonary atresia and intact ventricular septum demonstrate extensive connections with both the right and left coronary artery systems. In patients with TOF, pulmonary atresia, and major aortopulmonary collaterals, pulmonary blood supply is highly variable [176]. Although CT can document the anatomy of pulmonary arteries and collaterals adequately in many cases, catheterization with direct angiography, including selective imaging of the individual sources of pulmonary blood supply, provides the most reliable characterization (Figure 4.4) [177]. Figure 4.4 (A–F) These selective angiograms demonstrate pulmonary vascular anatomy in a neonate with tetralogy of Fallot, pulmonary atresia, and major systemic‐to‐pulmonary collateral arteries. There are multiple collaterals arising from the descending aorta and both subclavian arteries. One of the important uses of diagnostic catheterization is in the postoperative patient whose recovery is outside of the norm. There is minimal to no additional risk to early postoperative catheterization, and prompt identification of treatable abnormalities may help prevent some of the complications that occur with ongoing intensive medical support and facilitate identification of treatable anatomic problems [178, 179]. With the advancement of interventional catheterization technologies and techniques, many congenital and postoperative abnormalities that were formerly treated with surgery only can be managed in the catheterization laboratory. For some anomalies, such as patent arterial duct and simple ASD, transcatheter interventions have become the first‐line treatment at most centers. In other situations, such as congenital aortic stenosis or aortic coarctation, there are differences in opinion and practice around competing surgical and transcatheter options, which are influenced by the age of the patient and the specific nature of the lesion. Table 4.6 summarizes some major considerations in the decision‐making process for catheter or surgical interventions. In still other circumstances, surgical and catheter‐based management should be considered complementary tools in the management of chronic conditions. For example, patients with congenital heart defects who require reconstruction of the right ventricular outflow tract with a conduit, bioprosthetic valve, or plastic procedure inevitably develop pulmonary outflow obstruction or regurgitation with chronic pressure and/or volume loading of the RV [180–182]. Historically, surgical pulmonary valve replacement was the only option for unloading the RV in such situations, but transcatheter pulmonary valve replacement is now an alternative in many cases, including the treatment of dysfunctional bioprosthetic valves (Figure 4.5) [183–187]. Because most such patients will require multiple interventions over a lifetime, collaboration to determine the right sequence of surgical and transcatheter interventions, and selection of the most advantageous valves to implant in anticipation of the need for subsequent interventions, may be the approach that best serves the patient over time. Similarly, many surgeons have found it useful to incorporate catheter‐based tools into open or hybrid surgical procedures for CHD [188]. d‐Transposition of the great arteries (TGA) in which the aorta arises from the RV and the pulmonary artery arises from the left ventricle (LV), occurs in 2–5/10,000 live births, and accounts for 3–5% of congenital heart defects [189–192]. Associated noncardiac or genetic syndromes are relatively uncommon, identified in 4% of infants [32]. Due to parallel circulation of oxygenated and deoxygenated blood, preoperative survival depends on mixing of these circulations at the ductal and especially the atrial level. Associated VSDs are generally not sufficient for adequate mixing. Presence of a patent arterial duct and/or nonrestrictive atrial‐level shunt should be confirmed as soon as the diagnosis of TGA is suspected, as balloon atrial septostomy can be life‐saving. Balloon atrial septostomy can be performed under echocardiographic guidance and the success of the procedure can be assessed by 2D imaging, with color and pulsed Doppler interrogation demonstrating unrestrictive flow across the atrial septum. Transthoracic echocardiography is the imaging modality of choice in TGA and most patients are referred for surgical repair based solely on the detailed echocardiographic diagnosis. The parallel orientation of the great vessels can be clearly seen from subcostal or parasternal images (Figure 4.6). Slightly more than one‐half of patients have an intact ventricular septum, with VSDs present in 35–45%, associated with left ventricular outflow tract obstruction in ∼8%, which may be valvar or subvalvar due to posterior septal deviation, related to abnormal chordal attachments or coarctation [193, 194]. Coronary anomalies are present in 33–42% of patients [169, 195, 196]. The size of the VSD and coronary artery origins and course are key determinants of which procedure is performed and must be clearly defined to guide surgical planning. MR or CT may be used to clarify coronary artery anatomy preoperatively in patients with d‐TGA [197, 198]. The orientation of the semilunar valves is best assessed from the parasternal short‐axis view, which most commonly demonstrates the aorta anterior and rightward to the pulmonary artery in d‐looped TGA. The presence and location of any associated VSD can also be assessed with color sweeps in these planes. Tricuspid valve abnormalities, including attachments to the ventricular septum, straddling, or override, have been noted in more than 60% of patients with VSDs, most commonly inlet or malalignment‐type septal defects, and may result in postoperative tricuspid regurgitation [199]. In the setting of posterior malalignment VSDs, the subvalvar region and pulmonary valve must be clearly delineated from subcostal or apical views, as alternative surgical procedures other than the arterial switch, such as Rastelli or Nikaidoh repair, may be necessary [193, 194]. In the setting of right ventricular outflow tract obstruction (RVOTO), careful assessment of the aortic arch for possible coarctation is necessary. Table 4.6 Decision‐making for surgical or catheter intervention in congenital heart disease. ASD, atrial septal defect; PDA, patent arterial duct; RVOT, right ventricular outflow tract. Figure 4.5 These angiograms are from a patient who underwent transcatheter pulmonary valve replacement for stenosis of a right ventricle‐to‐pulmonary artery conduit after a Ross procedure for congenital aortic stenosis. (A) This pre‐implant angiogram demonstrates significant obstruction of the conduit (arrow). (B) This pulmonary arteriogram after a MelodyTM valve (Medtronic, Minneapolis, MN, USA) implant demonstrates a competent pulmonary valve and relief of the conduit obstruction. Coronary artery anatomy is variable, with anomalies present in about one‐third of patients, and determination of the coronary artery origins and branching are necessary prior to the arterial switch operation [169, 195, 200, 201]. Most commonly, the coronary arteries arise from the right‐ and left‐facing sinuses, with the left coronary artery branching to form the left anterior descending and left circumflex arteries. Clinically important coronary variants include a single coronary ostium with looping around the great vessels in 7–10% (Figure 4.7), multiple orifices from the same aortic sinus patterns in 3%, or intramural coronary patterns between the great arteries in 3–4% of patients [195]. Abnormal coronary artery variants have been associated with increased risk of death or reintervention following the arterial switch operation; while this risk has been attenuated at high‐volume centers, recent large single‐center studies still demonstrate increased mortality, particularly in the case of intramural coronary arteries [168, 169, 195, 196]. Figure 4.6 Transposition of the great arteries (TGA), echocardiogram. Parasternal long‐axis view showing d‐TGA with parallel orientation of the great vessels. The aorta (Ao) arises from the anterior right ventricle (RV) and the pulmonary artery (PA) arises from the left ventricle (LV). Figure 4.7 Single coronary artery in d‐transposition of the great arteries (d‐TGA), echocardiogram. Parasternal short‐axis view at the base of the heart showing the aortic valve (AV) anterior and rightward to the pulmonary valve (PV). There is a single coronary artery origin (asterisk) arising from the left‐facing sinus. The left anterior descending artery courses leftward with a normal course and the right coronary artery (arrow) courses anterior to the aortic root to the right atrioventricular groove. Coarctation of the aorta (CoA) refers to narrowing at the aortic isthmus and may be associated with more extensive hypoplasia of the aortic arch. CoA occurs in 3–4/10,000 live births and is present in 5–7% of patients with CHD [189, 190, 192]. Severe or critical coarctation presents in the neonatal period, while milder forms of coarctation can present throughout childhood, generally during evaluation for hypertension. CoA is frequently associated with other forms of left heart obstructive lesions, including abnormalities of the aortic and/or mitral valve. Echocardiographic assessment of the preoperative coarctation can demonstrate the severity and extent of aortic narrowing, adequacy of flow through the area of coarctation, and patency of the arterial duct. Hypoplasia of the more proximal aortic arch should be assessed to determine whether repair should be performed via lateral thoracotomy or median sternotomy [201–203]. Left ventricular hypertrophy and function must also be assessed. The anatomy of the aortic arch is best visualized from the suprasternal notch and from high infraclavicular imaging windows. In these views, the aortic arch may have an elongated appearance with distal displacement of the left subclavian artery (Figure 4.8A). The classic “3 sign” with posterior shelf is often seen, although the presence of a patent arterial duct may obscure this finding in neonates. In infants in whom the diagnosis of coarctation is difficult due to the presence of a patent arterial duct, a longer distance between the left common carotid and left subclavian arteries relative to the distal transverse arch dimension can be useful [204, 205]. Abdominal aortic pulsations are blunted, except in the case of a patent arterial duct with right‐to‐left systolic flow (Figure 4.8B–D). Continuous‐wave Doppler interrogation of the thoracic descending aorta may show a double envelope of lower‐velocity pulsatile flow proximal to the narrowing and high‐velocity flow with diastolic continuation distal to the coarctation. If echocardiography does not fully demonstrate the anatomy in patients with coarctation, CT or MRI angiography should be utilized; CT is also useful in the postoperative assessment of arch anatomy (Figure 4.9) [201, 206, 207]. Figure 4.8 Coarctation of the aorta, echocardiogram with Doppler. (A) Neonatal coarctation with a mildly hypoplastic transverse aorta with distal displacement of the left subclavian artery (LSCA). There is a posterior shelf and discrete coarctation (asterisk) at the isthmus. (B) Blunted abdominal aortic pulsations with continuation of flow in diastole consistent with significant aortic coarctation. (C) Discrete coarctation (asterisk) in a school‐aged child. (D) Double Doppler population showing the normal flow velocity (darker Doppler envelope) proximal to the coarctation and high‐velocity systolic flow with continuous antegrade flow throughout diastole consistent with coarctation. Figure 4.9 Aortic arch image, computed tomography (CT). 3D volume‐rendered reconstruction from a current‐generation CT of a patient with Shone complex and severe transverse aortic arch hypoplasia. Imaging was performed without sedation with a radiation dose of 1 milliSievert. TOF is the most common cyanotic congenital heart defect, occurs in 2–5/10,000 live births and accounts for 3–7% of CHD [189–192]. TOF includes a large malalignment VSD, position of the aortic valve over the interventricular septum, varying degrees of RVOTO, and right ventricular hypertrophy [208, 209]. TOF is frequently associated with noncardiac abnormalities and genetic syndromes in as many as 30% of infants, most frequently tracheoesophageal fistula and anal atresia [32]. The role of echocardiography in the preoperative assessment of TOF includes assessment of the size and extension of the VSD, determination of the anatomic level(s) of RVOTO, assessment of pulmonary valve annulus and branch pulmonary artery size and morphology for surgical planning, and assessment for any associated lesions [210]. The lesions most frequently associated with TOF include the presence of additional VSDs or ASDs, aortic arch anomalies, and coronary artery abnormalities. Right aortic arch is seen in approximately 25% of TOF cases and vascular rings should be excluded by transthoracic echocardiogram [211, 212]. Care should be taken to assess for associated coronary artery anomalies, which occur in 5–7% of patients with TOF [165, 213]. Recognition of a significant conal branch or origin of a left anterior descending artery from the right coronary artery with an anterior course across the RVOT is important to prevent damage to these vessels if an RVOT patch is necessary to relieve obstruction. Other rarer variations of TOF including associated complete AVSDs and TOF with absent pulmonary valve can be assessed by transthoracic echocardiogram. The location and size of the VSD as well as the degree of aortic override can be well seen from parasternal images (Figure 4.10). Subcostal sagittal or right anterior oblique imaging planes can also demonstrate the location and severity of RVOTO (Figure 4.10). The pulmonary valve annulus dimension should be measured and, if possible, valve morphology assessed from parasternal images. Parasternal short‐axis imaging shows the size and confluence of the branch pulmonary arteries and displays the length and degree of deviation of the conal septum. Late‐peaking or “dagger‐shaped” Doppler patterns are seen in dynamic or subvalvar RVOTO. The size of the pulmonary valve annulus may help guide the primary intervention in symptomatic neonates with TOF, as those with annular z‐scores greater than –4 are more likely to ultimately undergo valve‐sparing repair if initially palliated with a systemic‐to‐pulmonary artery shunt [214–216]. A larger pulmonary valve annulus is also associated with better outcomes in valve‐sparing repairs, with less need for postoperative reintervention [217]. Figure 4.10 Tetralogy of Fallot, echocardiogram. (A) Parasternal long‐axis image demonstrating a large anterior malalignment ventricular septal defect (VSD) (asterisk). The aortic root overrides the ventricular septum. The right ventricle is hypertrophied. (B) Subcostal right anterior oblique view demonstrating anterior malalignment of the infundibular septum (arrow), resulting in an elongated, narrow right ventricular outflow tract. The pulmonary valve annulus (x) is hypoplastic. There is a large VSD (asterisk) as a result of the malaligned septum. Ao, aorta; LA, left atrium; LV, left ventricle, RV, right ventricle. The aortic arch sidedness and anatomy are assessed from imaging in the suprasternal notch. In the most severe forms of TOF associated with pulmonary atresia, the supply of pulmonary blood flow via a patent arterial duct or major aortopulmonary collateral arteries (MAPCAs) arising from the aorta should be visualized. Depending on institutional expertise, CT or MR angiography can be used to delineate the number, course, and distribution of aortopulmonary collateral supply to all lung segments; CT compares favorably with angiography for delineation of collaterals [153, 177, 218]. Pulmonary atresia with intact ventricular septum is rare, occurring in less than 10% of infants with cyanotic CHD, and consists of pulmonary valve atresia with varying degrees of right‐sided hypoplasia [189]. The pulmonary vascular bed is usually supplied by normal‐sized branch pulmonary arteries, although branch pulmonary arteries are stenotic, hypoplastic, or discontinuous pulmonary arteries in at least 8% of cases [219–221]. Right ventricular and tricuspid valve morphology range along a spectrum from a severely hypoplastic and muscularized RV with a hypoplastic and competent tricuspid valve to a dilated RV with dilated tricuspid annulus and significant tricuspid regurgitation. An atrial‐level shunt (PFO or ASD) is generally present. Pulmonary blood flow is supplied by a patent arterial duct in almost all cases. Coronary artery anomalies including RV sinusoids, areas of stenosis, and/or ostial atresia are present in up to 50% of patients, especially in those with small, hypertensive RVs [166, 170, 219]. Coronary artery flow is determined to be RV dependent in 5–10% of patients and alters the management strategy [170, 171, 222]. Echocardiographic assessment of RV and tricuspid valve size and function, outflow tract characteristics, and presence of coronary fistulas and RV‐dependent coronary circulation is required to plan surgery [166, 170–172, 223]. Visualization of the RV is best performed from subcostal, parasternal, and apical views to allow visualization of the inflow, trabecular, and outflow portions of the RV (Figure 4.11). Tricuspid valve annular size is an important determinant in whether patients can undergo univentricular or biventricular repair, and tricuspid valve z‐score <2.5 is associated with RV‐dependent coronary arteries [166]. The mechanism of pulmonary valve atresia (membranous versus muscular) should be noted, as catheter decompression of the RV cannot be performed with infundibular or muscular atresia. Coronary ostial stenosis or atresia may be suggested by careful imaging of the aortic root. Cardiac catheterization with angiography to assess coronary artery anatomy and suitability for biventricular repair has been traditionally required, particularly in the setting of RV‐dependent coronary circulation (Figure 4.3) [224]. In the case of extensive MAPCAs, excellent diagnostic accuracy of CT angiography with lower radiation exposure may be necessary to assess the distribution and extent of collateral flow (Figure 4.12). Figure 4.11 Pulmonary atresia with intact ventricular septum, echocardiogram. (A) Apical four‐chamber view demonstrating a mildly hypoplastic and muscle‐bound right ventricle (RV) with dysplastic, thickened tricuspid valve (asterisks). This patient had tricuspid regurgitation and did not have RV‐dependent coronary arteries. (B) High parasternal short‐axis imaging demonstrating the atretic pulmonary valve (arrow) with a mildly hypoplastic main pulmonary artery and normal‐size branch pulmonary arteries. The outlet portion of the RV (asterisks) is muscularized and severely hypoplastic. (C) Color Doppler image demonstrating the branch pulmonary arteries fed by the patent arterial duct (PDA). There is no antegrade flow seen across the pulmonary valve (arrow). Ao, aorta; LA, left atrium; LPA, left pulmonary artery; LV, left ventricle; RA, right atrium; RPA, right pulmonary artery.
CHAPTER 4
Preoperative Diagnostic Evaluation
Age group
Procedure
# procedures
Discharge mortality
Neonates (0–30 days)
Arterial switch
Arterial switch + VSD closure
1888
785
2.7%
5.3%
Norwood procedure
2810
15.7%
Coarctation repair
3964
1.3%
Common arterial trunk
631
9.4%
Infants (31 days–1 year)
Bidirectional cavopulmonary connection (Glenn) and Hemi‐Fontan
4839
2.5
TOF repair
4648
1.1%
VSD repair
7250
0.6%
AV septal defect, complete repair
3169
3.0%
Lesion
Anatomic features
Associated anomalies
Considerations
ASD
Secundum ASD: 70%
Ostium primum ASD: 15–20%
Sinus venosus defects: 3–11%
Coronary sinus defects (unroofed coronary sinus)
Partial anomalous venous drainage with sinus venosus defects
Echocardiography sufficient except in case of sinus venosus ASD and PAPVC
MRI to assess pulmonary venous anatomy, Qp/Qs in sinus venosus defects
VSD
Perimembranous: tricuspid–aortic fibrous continuity
Muscular
Inlet
Doubly committed
Aortic valve prolapse
Double‐chambered RV
Subaortic membrane
ASD
Echocardiography generally sufficient for preoperative imaging
MRI in complex VSD anatomy associated with other major conotruncal abnormalities
AVSD
Ostium primum ASD
Inlet VSD
Atrioventricular valve “cleft”: zone of apposition between superior and inferior bridging leaflets
Gooseneck deformity of left ventricular outflow tract
Double‐orifice left atrioventricular valve
TOF
Common atrium
Trisomy 21
Heterotaxy syndrome
Echocardiography generally sufficient for preoperative imaging
MRI in unbalanced defects to assess ventricular size, relationship of valve to chamber
Anomalous origin of coronary artery from pulmonary artery
Anomalous origin of left coronary artery from pulmonary artery (ALCAPA)
Anomalous origin of right coronary artery from pulmonary artery (ARCAPA)
Anomalous single coronary artery from pulmonary artery
Slit‐like orifice
Intramural course
Acute angulation of coronary take‐off
Echocardiography often adequate for diagnosis, MRI or CT recommended for detailed visualization of coronary origin and intramyocardial course
Ebstein anomaly of the tricuspid valve
Apical displacement of tricuspid valve leaflets (septal)
Sail‐like anterior leaflet of TV
Atrialized (large) right atrium
Pulmonary stenosis/atresia
ASD/PFO
VSD
Left ventricular noncompaction
L‐TGA
Ventricular pre‐excitation
Echocardiography is main imaging tool
Preoperative electrophysiology study with possible ablation for pre‐excitation
Lesion
Surgical intervention
Imaging considerations
Functionally single ventricle
Stage 1 Norwood procedure
Stage 2 intervention: bidirectional Glenn
Stage 3 intervention: Fontan procedure
Traditionally diagnostic catheterization has been used; consider MR vs. CT angiography depending on center’s expertise
Tetralogy of Fallot
Pulmonary valve/conduit replacement
Consider CMR to delineate ventricular size, function, and degree of regurgitation
Common arterial trunk
Conduit replacement
Pulmonary valve insertion
Consider CT for superior delineation of extracardiac anatomy
Coarctation of aorta
Interposition graft placement
Transcatheter intervention
Consider CT scan for optimal visualization of arch and collaterals
Extracardiac and Genetic Evaluation
Lesion
Anatomic features
Associated anomalies
Considerations
Transposition of the great arteries
d‐TGA: aorta arises from RV; pulmonary artery arises from LV in parallel orientations.
Atrial‐level shunting
± PDA
Coronary arteries arise from right and left sinuses facing the pulmonary valve
VSD: 40–45%
Left ventricular outflow tract obstruction: 8%
Coronary artery variants: 33–42%
Noncardiac anomalies/genetic syndromes: 4%
Echocardiography may guide balloon atrial septostomy at bedside
Consider MRI or CT for complex coronary anatomy, complex aortic arch anatomy
Tetralogy of Fallot
Anterior malalignment of infundibular septum
Large malalignment VSD
Aortic valve overrides ventricular septum
Right ventricular outflow tract obstruction
Right ventricular hypertrophy
Branch pulmonary artery stenosis
Additional ASD or VSD
AV septal defects
Right aortic arch 25%
Coronary artery variants (∼5%)
Frequent noncardiac anomalies/genetic syndromes: 30% 22q11 deletion syndrome
Echocardiography typically adequate
Consider MRI or CT for coronary anatomy, discontinuous branch pulmonary arteries, subclavian isolation, vascular ring, etc.
Pulmonary atresia with intact ventricular septum
Membranous or muscular pulmonary atresia
Right ventricle hypoplasia
Hypoplastic tricuspid valve
Possible RV‐dependent coronary arteries: 5–10%
Branch pulmonary artery abnormalities: 8%
Coronary artery anomalies, including sinusoids present in up to 50%
Echocardiography for diagnosis, RV size, TV annulus and type of PV atresia
Angiography or advanced imaging necessary to assess for RV‐dependent coronary artery
Hypoplastic left heart syndrome
Hypoplastic or atretic left heart lesions
Aortic atresia/mitral atresia
Aortic atresia with mitral stenosis
Aortic stenosis with mitral stenosis
Aortic arch hypoplasia
Anomalous pulmonary venous drainage: 5%
Tricuspid valve abnormalities: 33%
Coronary artery anomalies
Noncardiac /genetic anomalies: 8–11%
Echocardiography generally sufficient for preoperative imaging
Angiography for coronary anomalies
Common arterial trunk
Single arterial vessel arising from heart, giving rise to aorta, coronary arteries, and pulmonary arteries
Large subtruncal VSD almost always present
Truncal valve abnormalities
Right aortic arch: 27–36%
Coarctation/interruption of aorta: 11–19%
ASD, additional VSDs
PDA
Frequent noncardiac/genetic anomalies: 37%, especially 22q11 deletion
Echocardiography generally sufficient for preoperative imaging
Consider MRI or CT for complex aortic arch anatomy, discontinuous branch pulmonary arteries, truncal valve assessment
Total anomalous pulmonary venous connection
Pulmonary venous confluence and anomalous drainage
Supracardiac 40–48%
Cardiac: 15–20%
Infracardiac: 10–26%
Mixed: 3–9%
Obligate atrial septal defect
Right heart dilation
Pulmonary venous obstruction: 30–80%
Heterotaxy syndrome, particularly with infracardiac venous drainage
Noncardiac/genetic anomalies: 3–12%
Echocardiography generally sufficient for preoperative imaging
Consider MRI or CT to delineate anomalous pulmonary venous drainage, especially mixed type
Coarctation of aorta
Narrowing of aortic isthmus with discrete stenosis distal to left subclavian artery
Posterior shelf with “3‐sign” in distal arch
Distal displacement of left subclavian artery
Hypoplasia of proximal aortic arch
± PDA
Bicuspid aortic valve ∼85%
Ventricular dysfunction, may be profound
Echocardiography
Consider CT or MRI for complex arch anatomy, severe coarctation
Electrocardiogram and Rhythm Evaluation
Noninvasive Imaging of Congenital Heart Disease
Echocardiography
Cardiac Magnetic Resonance Imaging
Cardiac Computed Tomography
Predischarge Imaging or Catheterization
Cardiac Catheterization
Catheterization data that may assist in decision‐making
Lesions
Assessment to determine whether surgical intervention is indicated
Angiographic evaluation in the setting of inconsistent or ambiguous data obtained from noninvasive imaging
Shunt size: assess systemic and pulmonary blood flows
Determine suitability of shunt lesions for closure
Balloon test occlusion of shunts/connections
Atrial septal defect
Systemic‐to‐pulmonary artery shunt
Left superior caval vein draining to the left atrium
Pressures or gradients
Valvar, subvalvar, intracavitary obstructions
Pulmonary hypertension
Pharmacologic evaluation of pulmonary vascular resistance (e.g., nitric oxide)
AV septal defect with upper airway obstruction, Down syndrome
Assessment to understand physiology and/or anatomy
Cardiac output
Pulmonary vascular resistance
Collateral vessels
Single ventricle lesions
Coronary artery anatomy
Pharmacologic evaluation of coronary flow reserve (e.g., fractional flow reserve)
Anomalous origin of coronary artery, acquired obstructions or aneurysms
Coronary–left ventricle connections
HLHS with mitral stenosis and aortic atresia
Assessment to aid in surgical planning
Complex vessel anatomy
Collaterals in tetralogy of Fallot with pulmonary atresia
Sites of obstruction
Valvar and subvalvar stenosis
Peripheral pulmonary artery stenoses
RV‐dependent coronary circulation
Pulmonary atresia with intact ventricular septum
Pharmacologic evaluation of pulmonary vascular resistance (e.g., nitric oxide)
Evaluate need for/potential benefit of therapy in perioperative period
Catheter‐Based Interventions
Neonatal Cardiac Surgery
d‐Transposition of the Great Arteries
Lesion
Anatomic considerations
Comments
PDA
Patient size
Size and length of PDA
Size of descending aorta and left pulmonary artery
Very few indications for surgical closure for isolated PDA, other than in very small infants (<2 kg), although there is growing experience with transcatheter closure in the very tiny premature infant
ASD secundum
Size and location of ASD
Presence of multiple defects
Presence and size of rims, particularly around the inferior and posterior aspects of the ASD
Adequate device closure difficult with absent posterior or inferior rims
Coarctation of aorta (COA), neonate
Size of arch and descending aorta
Additional levels of obstruction (e.g., transverse arch)
Relationship of COA to head and neck vessels
Anatomy of arch branching
Discrete coarctation is usually anatomically amenable to surgery or catheter intervention
Surgery preferred unless patient has contraindication to or is a poor candidate for surgery
Palliative catheter intervention may be an option in very low weight neonates
Coarctation of aorta, older child
Size of arch and descending aorta
Additional levels of obstruction (e.g., transverse arch)
Proximity of coarctation to head and neck vessels
Anatomy of arch branching
Tortuosity of arch
Often a matter of choice or physician preference
Transcatheter therapy may require an additional procedure in early adulthood to make sure the coarctation stent (if a stent is used) reaches adult size
Aortic stenosis
Annulus size
Calcification of leaflets
Leaflet morphology
Presence of aortic regurgitation
Most centers prefer balloon valvuloplasty for noncalcific aortic stenosis if there is minimal aortic regurgitation
Some single‐center studies suggest that longer‐term outcomes with surgical valvotomy are equivalent to or superior to balloon dilation
Pulmonic stenosis
Dysplastic valve
Supravalvar stenosis
Straightforward valvar pulmonary stenosis can essentially always be treated with catheter intervention, but dysplastic pulmonary valve or coexisting supravalvar stenosis may require surgery
Branch pulmonary artery stenosis
Number of vessels involved
Location of stenosis or multiple stenoses
Size of vessels adjacent to stenosis
Underlying etiology of disease (e.g., Williams syndrome, Alagille syndrome, postoperative stenosis, etc.)
For focal stenosis, balloon angioplasty is often very effective
For kinks, torsion, or other mechanical distortions, stenting or surgery is necessary
Very little data on relative effectiveness of surgery versus catheter interventions
Pulmonary valve replacement
RVOT size
Relationship between coronary arteries and conduit or RVOT
Involvement of pulmonary artery bifurcation, or presence of bifurcation stenosis
Transcatheter therapy effective for most stenotic or regurgitant large conduits and bioprosthetic valves
Catheter options not yet adequate for pulmonary regurgitation in the setting of large, patched, or native RVOT
Aortic valve replacement
Annulus size
Calcification of leaflets
Presence of subvalvar or supravalvar stenosis
Underlying cause of disease
Transcatheter aortic valve replacement (TAVR) not yet standard of care for aortic valve replacement for pediatric patients or young adults, or for primary aortic regurgitation
TAVR may be an option in young patients with very high surgical risk, and in young adults with multiple prior surgeries
Pulmonary vein stenosis
Extent of disease
Number of veins involved
Involvement of confluence if surgically repaired anomalous pulmonary venous return
For complex pulmonary vein disease, one attempt at surgical repair is typically indicated before resorting to catheter‐based balloon angioplasty or stenting
Catheter interventions are often effective acutely, but short‐term recurrence is common
Coarctation of the Aorta
Tetralogy of Fallot
Pulmonary Atresia with Intact Ventricular Septum
Stay updated, free articles. Join our Telegram channel

Full access? Get Clinical Tree
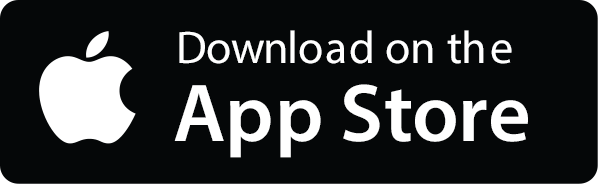
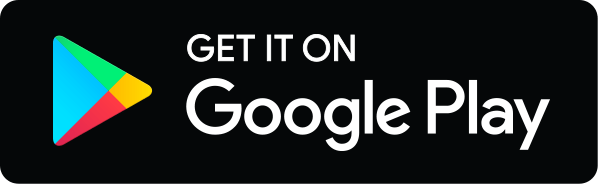