PRENATAL SCREENING OF CONGENITAL HEART DISEASE
Introduction
Congenital heart defects (CHDs) can present as a clinically significant structural heart disease at birth,1,2 or be asymptomatic and diagnosed at an older age.3 CHDs are of several different types and comprise different phenotypes, ranging from simple defects to complex defects with severe, life-threatening symptoms. The incidence of CHD in different studies varies but appears to be at least 1 per 100 live births, with a large number of cases not surviving to birth.2 The incidence of CHD is similar worldwide, with some minor differences in the subtypes varying with different countries. The etiology of CHD has been viewed as being multifactorial based on a study of recurrence and transmission risks as well as being a heterogeneous category of developmental anomalies, which is a product of genetic and environmental interaction.4 The options currently available to clinicians for prenatal diagnosis of CHD include a detailed maternal and family history, testing for genetic derangements associated with these defects, and image-based screenings.
Prenatal Screening by History
CHDs due to multifactorial inheritance Many common heart malformations have now been demonstrated to be due to a multifactorial pattern of inheritance, some environmental exposures being subtle and difficult to define,5 while others have been found to have more genetic influences with recurrences of CHD in families.6 Prenatal risk may contribute to the manifestations of the disease but may or may not cause the disease.7 In a retrospective study of 326 cases in diabetic mothers, it was found that diabetic women were at an increased risk of having a baby with CHD such as transposition of the great arteries (TGA), tetralogy of Fallot (TOF), and pulmonary atresia.8 In a case–control study, it was found that maternal smoking during pregnancy was associated with septal defects and right-sided obstructive lesions.9,10 Preconception multivitamin use defined as regular use during the first 3 months of pregnancy was found to be associated with a 24% reduced risk of nonsyndromic cardiac defects; there was a statistically significant reduction of 54% in outflow defects and 29% in ventricular septal defects (VSDs).11
Prenatal Diagnosis by Genetic Evaluation
While some CHDs have been found in children born without any other birth defects, in some cases CHDs can occur in a syndrome spectrum or in conjunction with other congenital anomalies, including facial, limb, organ, developmental, or growth abnormalities. These anomalies can now be diagnosed by genetic testing, including fluorescence in situ hybridization (FISH), cytogenic techniques, and DNA mutation analysis. Prenatal diagnosis and preimplantation genetic diagnosis of chromosomal anomalies and single-gene disorders are now routinely available, but raise several ethical and decision-making questions. These tests are usually performed by collection of fetal samples through invasive procedures such as amniocentesis and chorionic villus sampling, but these have been associated with some risk of complications such as spontaneous abortion, amniotic fluid leakage, infection, premature labor, and fetal injury. Noninvasive prenatal diagnosis techniques have now been found to be possible for prenatal diagnosis through examination of intact fetal cells and DNA material within maternal blood. Cell-free fetal DNA can be detected in maternal blood after 7 weeks.12 Noninvasive techniques, although safe in pregnancy, have poor accuracy, with false-negative rates between 12% and 23% and false-positive rates between 1.9% and 5.2%.13
CHDs due to chromosomal defects About one in eight CHDs are seen in relationship to a chromosomal anomaly.14 These chromosomal anomalies have been detected using chromosomal karyotyping in patients with CHD. Aneuploidy, microdeletion, and inversion/duplication account for a significant amount of CHD. About 50% of individuals with trisomy 21 have CHD ranging from atrial septal defects (ASDs) and VSDs, almost all patients born with trisomy 18 will have CHD, commonly as septal defects, and approximately a quarter of females with Turner syndrome will have CHD.15 Structural syndromes such as cri-du-chat, cat eye, and Jacobsen syndromes have been linked to septal defects, total anomalous pulmonary venous return (TAPVR) or TOF, and hypoplastic left heart syndromes (HLHS), respectively. Similarly, DiGeorge syndrome (conotruncal defects) and Williams–Beuren syndrome (supravalvular stenosis) have been associated with CHD in over half the cases.14
Single-gene defects associated with CHDs Single-gene defects in structural proteins, receptors, ligands, signaling proteins, transcription factors, and cofactors have been discovered in several of the CHDs (Table 5.1). Besides CHDs, single-gene defects have also been associated with cardiomyopathies and rhythm disturbances such as long QT syndromes. Single-gene defects associated with CHDs also include syndromes with a unique constellation of congenital defects such as cardiofaciocutaneous, Marfan, Duane-radial ray, Kabuki, Alagille, Holt–Oram, CHARGE, heterotaxy, Char, LEOPARD, Noonan, Ellis–van Creveld, and Costello syndromes.
Table 5.1. Gene mutations associated with CHDs
Congenital Heart Defect | Associated Gene Mutations |
Atrial and ventricular septal defects | TBX5, NKX2.5, GATA4, IRX4, MYH6, ACTC |
TOF | JARID2, TBX1, NKX2.5, JAG1, NOTCH1 |
Bicuspid aortic valve, coarctation of aorta | NKX2.5, NOTCH1, SMAD6, VEGF |
Endocardial cushion defects | ALK2, CRELD1, LEFTY2, GATA4, GATA6 |
Transposition of great arteries (TGA) | NODAL, LEFTY2, GDF1, CFC1, ZIC3, MED13L |
Ebstein’s anomaly | MYH7 |
Double-outlet right ventricle (DORV) | NKX2.5, NODAL, GDF1, ACVR2B, ZIC3, FOG2 |
Patent ductus arteriosus (PDA) | GATA6, TFAP2B, MYH11 |
Interrupted aortic arch (IAA) | NKX2.5, NKX2-5, CFC1, CRYPTIC, LEFT2 |
HLHS | GJA1, ZIC3, NKX2.5 |
Truncus arteriosus (TA) | NKX2-6 |
Tricuspid atresia | MYH6 |
TAPVR | ANKRD1, ZIC3, GJA1, PDGFRA, NODAL |
Marfan syndrome (MFS) is an autosomal dominant disorder caused by a mutation in the fibrillin-1 gene; the clinical symptoms of MFS involve the ocular, skeletal, and cardiovascular system.16 The genetic etiology associated with MFS was discovered using traditional positional clonal methods.16
Alagille syndrome (AGS) is another single-gene anomaly associated with CHD. AGS is an autosomal dominant, multisystem disorder affecting intrahepatic bile ductal paucity, as well as skeletal, renal, facial, ocular, and cardiac abnormalities including peripheral pulmonary artery (PA) stenosis, pulmonary valve (PV) stenosis, and TOF.17 Cardiac involvement also leads to calcific aortic valve disease.18 JAG1, one of the five Notch signaling ligands, is the primary gene mutation in AGS and is associated with a high mutation rate of about 60%. The penetrance of JAG1 mutations is about 95%, but expressivity is highly variable and many carriers only have subclinical manifestations.19 This JG1 gene mutation has been mapped in a commonly deleted location of 20p12; therefore, patients suspected of having AGS should undergo karyotyping and FISH analysis to detect a 20p12 mutation, and if the diagnosis is confirmed, the child should be further evaluated for other clinical features of the syndrome spectrum.19
Holt–Oram syndrome is an autosomal dominant syndrome affecting the heart and skeletal system, mainly the upper limbs, and is commonly referred to as the hand–heart syndrome. The common forms of CHD associated with Holt–Oram syndrome are ASDs and VSDs. Cardiac electrical conduction defects and atrial fibrillation can also occur. TBX5 is critical for development of limb and heart development, and that mutation in the TBX5 gene underlies Holt–Oram syndrome. TBX5 is a member of the T-box transcription factor family; both nonsense and missense mutations have been found in affected family members with Holt–Oram syndrome.20,21
Noonan syndrome is an autosomal dominant, genetically heterogeneous condition characterized by distinctive facial features, short stature, chest deformities, CHD, and other comorbidities. Diagnosis of Noonan syndrome was dependent mainly on clinical phenotypes, but more recently genetic mutations are identifiable in about 61% of patients.22,23 The CHDs observed in Noonan syndrome are PV stenosis, secundum-type ASD, atrioventricular septal defects (AVSDs), mitral valve abnormalities, coarctation of the aorta (CoA), TOF, and hypertrophic cardiomyopathy being the most common at 80% to 90%.4 Mutation of the KRAS gene encoding for the RAS protein is a known cause of Noonan syndrome. The KRAS mutation c.179G>T was identified by DNA sequence analysis from peripheral blood leukocytes in a patient with hypertrophy of both ventricles and thickened dysplastic atrioventricular (AV) and semilunar valves.24
Heterotaxy is another syndrome associated with single-gene defects characterized by multiple congenital malformations with abnormal arrangements of thoracic and abdominal organs along with complex cardiovascular malformations. The heart defects seen in heterotaxy syndrome are most commonly AVSDs and transposed great arteries. Cilia function is critical to the development of normal organ laterality, and thus heterotaxy syndromes are found to be related to primary ciliary dyskinesia.25 Familial cases with autosomal dominant, autosomal recessive, and X-linked inheritance are known, though it is more commonly sporadic. Mutations in ZIC3 and ACVR2B have been identified as a common cause of heterotaxy.26
Prenatal Diagnosis of CHDs by Imaging
Accurate diagnosis and treatment of diseases can be achieved by an effective screening program allowing for appropriate neonatal care. It is well known that the prevalence of cardiac abnormalities is higher in early gestation, and these lesions are an important cause of very early miscarriages. This study clearly outlines the importance of early diagnosis, encouraging well-informed decisions, specifying the importance of rareness of the disease as some of the crucial factors.27
Screening for CHD has been done by radiological methods using ultrasound including echocardiography. Currently, antenatal screening of CHD is being achieved by elective ultrasound scanning initially with fetal echocardiography from 18 weeks upwards. Despite all these evolving diagnostic modalities and increasing prenatal diagnoses, little is known about how to reveal the diagnosis of fetal CHD to parents and families and what are their expectations. A recent internet survey of 841 parents with CHD in their child gave some important information of parental experience with both the pediatric cardiologist and the perinatologist, where most parents felt they had little time to make decisions after diagnosis and a significant percent of them also felt more pressured by the perinatologist than by the pediatric cardiologist for termination of pregnancy. The reader is referred to Chapter 7 for a further discussion of ethical issues in managing fetal and neonatal cardiac lesions
Prenatal diagnosis of CHD has many advantages, but not just limiting to overall improvement in birth outcomes28 and presurgical morbidity and mortality.29 It helps in proper family counseling of postnatal outcomes, planning delivery in an appropriate facility, and also in coordinating multidisciplinary perinatal care by the perinatologist, neonatologist, pediatric cardiologist, and geneticist.30 Apart from initial diagnosis, intrauterine fetal imaging is also used to follow serial progression of disease and for appropriate timely induction of delivery31 with necessary delivery room arrangements pertinent to each diagnosis.32 Prenatal screening, which has been evolving over the last 30 years, started as a specialty primarily dealt with by pediatric cardiologists but now shared between maternal and fetal specialists and pediatric cardiologists.33
Variable prevalence of CHD was noticed among mothers with different indications for prenatal fetal cardiac screening, with the group suspected to have CHD on screening obstetric ultrasound having the highest incidence of CHD on fetal echocardiography, which is close to 60%.34 CHD can be diagnosed from available modalities with variable accuracy, anywhere from 31% to 96%.35
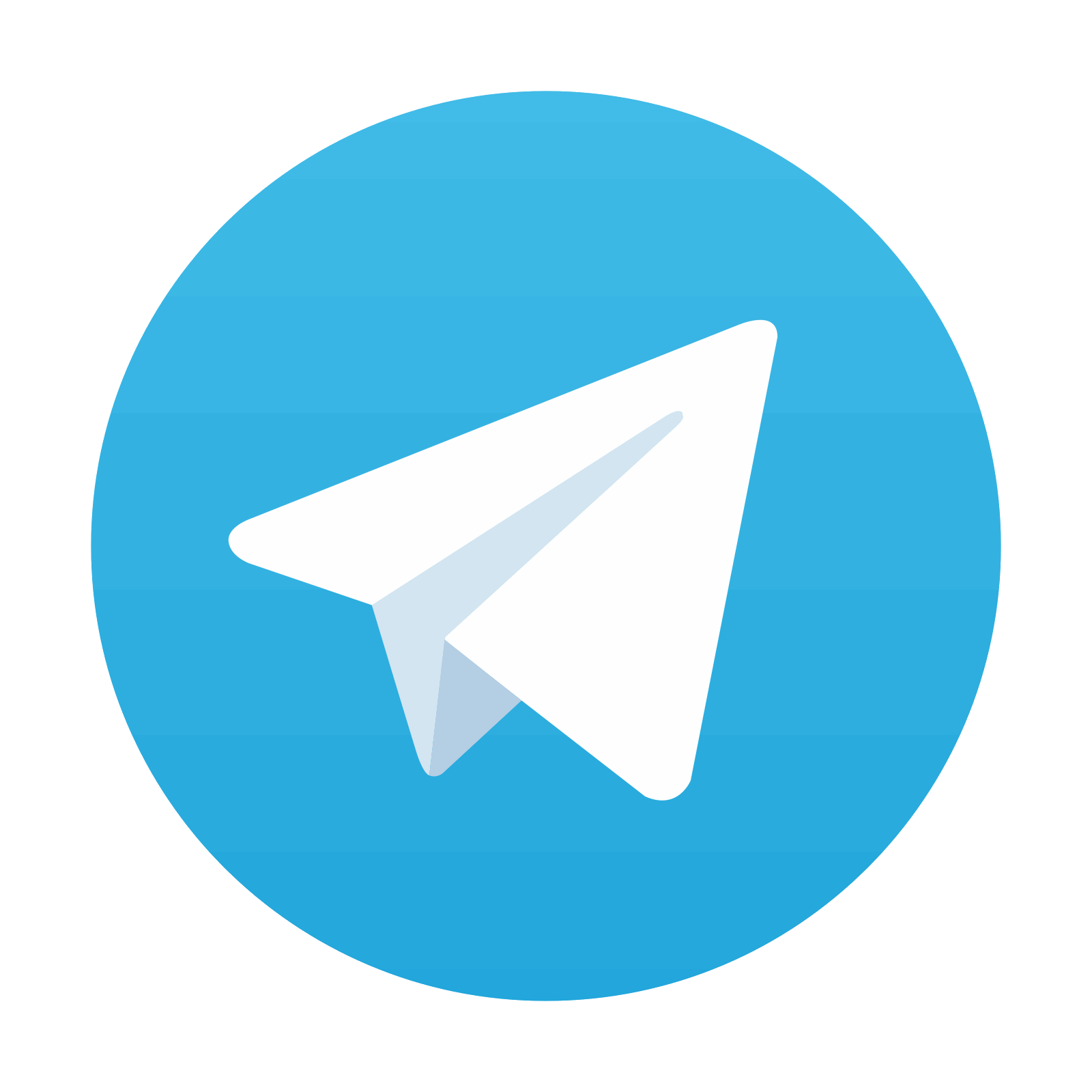
Stay updated, free articles. Join our Telegram channel

Full access? Get Clinical Tree
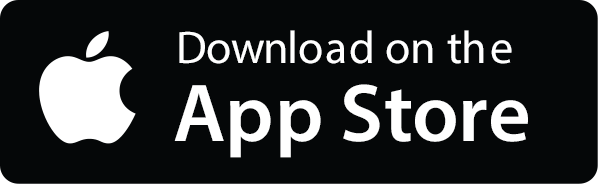
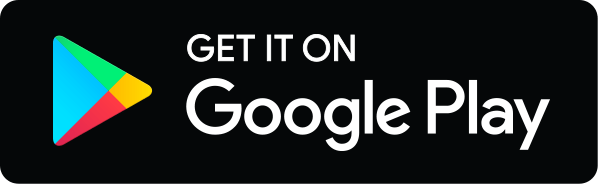