HYPOPLASTIC LEFT HEART SYNDROME
Introduction
In the preceding 5 chapters, the so-called “5Ts,” namely transposition of the great arteries (TGA), tetralogy of Fallot (TOF), tricuspid atresia, total anomalous pulmonary venous connection (TAPVC) and truncus arteriosus (TA) were discussed. In this chapter, we will review hypoplastic left heart syndrome (HLHS).
The term HLHS was initially proposed by Noonan and Nadas1 to describe a diminutive left ventricle with underdevelopment of aortic and mitral valves (MVs). A patent foramen ovale (PFO) is frequently present. The ventricular septum is typically intact. A patent ductus arteriosus (PDA) allows blood flow into the systemic circulation. Aortic coarctation is not infrequently present.
HLHS is a uniformly lethal congenital heart defect (CHD) unless it is surgically treated. Following the report of surgical palliation by Norwood in the early 1980s2,3 and description of allograft cardiac transplantation by Bailey in the mid-1980s4 for treatment of HLHS, attention to this lesion has extremely increased. Reported incidence of HLHS varies between 0.21 and 0.28 per 1000 live births, 5 comprising 1.2% to 1.5% of all CHD.6 HLHS accounts for 7% to 9% of all CHD diagnosed in the first year of life.5,6 Prior to the availability of surgical treatment, HLHS accounted for 25% of cardiac deaths in the neonatal period.7 The prevalence of HLHS is higher in males than in females with a 55% to 70% male preponderance. A higher incidence of HLHS is noted in infants with Turner syndrome, Noonan syndrome, Smith–Lemli–Opitz syndrome and Holt–Oram syndrome than in normal children. Certain chromosomal duplications, translocations and deletions are also seen in association with HLHS.
In this chapter, we will discuss anatomic, physiologic and clinical features, noninvasive evaluation and management of HLHS in the neonate. The readers interested in detailed discussion of HLHS, particularly beyond the neonatal period, are referred to our comprehensive reviews published elsewhere.8–11
Pathologic Anatomy
The left ventricle (LV) is generally a slit-like cavity with thick muscle, particularly when mitral atresia is coexisting. The aortic valve is severely narrowed or atretic with annular hypoplasia. Likewise, the MV is atretic, severely stenotic or hypoplastic. The left ventricular cavity is very small when the MV is not atretic. Endocardial fibroelastosis is frequently present. The ascending aorta (AAo) is very hypoplastic with its diameter no more than 2 to 3 mm. However, it is sufficient to supply adequate coronary blood flow in a retrograde manner. The left atrium (LA) is small and hypoplastic. The interatrial septum is typically thickened with a small PFO and infrequently the atrial septum is intact. A PDA is typically present and is essential for baby’s survival.
The LV may be severely hypoplastic in hearts associated with double-outlet right ventricle (DORV) with mitral atresia, unbalanced complete atrioventricular (AV) septal defect, and other complex CHD. In some studies, these variants constitute as many as 25% of HLHS babies.7,10
Pathophysiology
Prenatal Period
In the fetus, the oxygenated blood from the placenta comes into the inferior vena cava and mixes with the superior vena caval blood in the right atrium (RA) and is passed on into the RV instead of being shunted via the PFO into the LA. The pulmonary venous blood entering the LA is shunted across the PFO into the RA because of MV obstruction. This mixed right atrial blood is propelled into the RV (across the tricuspid valve) and then into the pulmonary artery (PA).12 Because the PDA is widely open and the pulmonary vascular resistance (PVR) is high in the fetus, the majority of the blood is shunted into the aorta (via the ductus) and only a small fraction of the blood enters the branch PAs and lungs. The blood entering the aorta gets redistributed into the aortic arch, brachiocephalic vessels, AAo and coronary arteries in a retrograde fashion and to the descending aorta (DAo) and placenta anterogradely. The relative distributions into these vascular beds depend on the respective vascular resistances. The venous return from the placenta as well as the fetus completely mixes in the RA with resultant identical oxygen (O2) saturation to all remaining parts of the fetal heart.
Postnatal Period
The cardiovascular physiology in the neonate with HLHS is complex. Pulmonary venous blood returns to the LA, but cannot enter into the left ventricle because of MV atresia, hypoplasia or stenosis. Consequently, fully saturated pulmonary venous blood is shunted across the atrial septum (Figure 33.1) and mixes with desaturated systemic venous blood in the RA. From there, it is passed on to the RV and PA. Blood leaving the RV and PA is distributed into the lungs via the branch PAs and into the aorta via the PDA (Figure 33.1). The blood flows into the upper part of the body and heart via aortic arch, AAo, and the coronary arteries in a retrograde direction (Figure 33.1). The rest of the body is perfused from the descending aorta. Thus, the RV pumps the pulmonary and systemic venous blood admixture into both the pulmonary and the systemic circulations which are connected parallel (rather than in series) by the ductus arteriosus (DA).
Figure 33.1. Box diagram of HLHS. The pulmonary venous return cannot exit into the LV, and its egress must be into the RA via the PFO. Because there is no forward flow from the left heart into the hypoplastic aorta, the systemic perfusion is dependent upon the PDA. Retrograde flow into the brachiocephalic vessels and coronary arteries is also shown. If the ductus constricts, the systemic perfusion is compromised. If the foramen ovale is obstructive, the infant will develop signs of pulmonary venous obstruction. Reproduced with permission from Neonatology Today 2011;6(12):1–9.
The relative flows to the pulmonary and systemic circulations depend on the respective resistances of the 2 vascular circuits. At or shortly after birth, the PVR declines, allowing a larger percentage of the right ventricular output into the lungs than the body. While increased pulmonary blood flow (PBF) produces higher O2 saturation, it decreases the systemic blood flow (SBF). When the SBF decreases below a critical level, the systemic perfusion becomes poor with resultant metabolic acidosis and oliguria. There is concomitant decrease in blood flow to the brain and coronary arteries with an attendant risk of cerebral or myocardial ischemia, as the case may be. On the other hand, if PVR is appreciably higher than systemic vascular resistance (SVR), the PBF will decrease and hypoxemia will ensue. Thus, the postnatal circulation in HLHS is dependent on adequacy of interatrial communication, patency of the DA and relative levels of PVR and SVR.
Clinical Features
Infants with HLHS usually present with symptoms within the first 24 to 48 hours of life. The symptoms begin as soon as the DA starts to constrict; ductal closure decreases SBF, produces shock and, without intervention, causes death. Infants who have pulmonary venous obstruction (absent or restrictive PFO) may become symptomatic even earlier. Infants with persistence of high PVR as well as widely patent ductus have balanced pulmonary and systemic circulations and may present much later, although such cases are very rare. Thus, the time of presentation depends on amount of atrial level obstruction, patency of the DA and PVR.
At the present time, however, a good number of HLHS babies are identified prenatally because of abnormal screening obstetric ultrasound. Prenatal identification of HLHS permits time for parental counseling and planning of delivery at a tertiary care hospital, which helps avoid transport-related morbidity. Immediately after delivery, prostaglandin E1 (PGE1) infusion should be started to maintain ductal patency and an echocardiogram performed to confirm HLHS diagnosis and to evaluate the adequacy of shunting across the PFO. In neonates without prenatal diagnosis, most neonates are born at term and appear normal initially. As the DA begins to constrict, usually over the first 24 to 48 hours after birth, symptoms of tachypnea, respiratory distress, cyanosis, pallor, lethargy, metabolic acidosis and oliguria develop. Without immediate treatment to reopen the DA, death quickly ensues. Similar symptoms may be seen if an abrupt drop in PVR takes place. Babies with restrictive PFO (approximately 2%–5%) may present with respiratory symptoms and severe cyanosis because of pulmonary venous obstruction.
Prior to initiation of PGE1 infusion to restore patency of the DA, babies exhibit signs of cardiogenic shock, including hypothermia, tachycardia, respiratory distress, central cyanosis, pallor, poor peripheral perfusion with weak pulses in all extremities and in the neck, and hepatosplenomegaly. Following restoration of SBF through the DA, signs of shock improve, leaving a stable infant with tachycardia, tachypnea and mild central cyanosis. In the presence of aortic coarctation, arterial pulses in the lower extremities are better felt than those in the arms, especially in the right arm. The right ventricular impulse is increased. Auscultation reveals normal to loud first heart sound and a loud, single second heart sound. Frequently no cardiac murmurs are appreciated; however, a nonspecific, ejection systolic murmur at the left upper sternal border, a high-pitched, holosystolic murmur at the lower left sternal border, indicating tricuspid regurgitation and a diastolic flow rumble over the precordium, indicating increased right ventricular diastolic filling may be auscultated in some infants.
Noninvasive Evaluation
Chest X-Ray
While the chest x-ray is not diagnostic, it does show severe cardiomegaly with increased pulmonary vascular markings (Figure 33.2). However, in babies with restrictive atrial shunt (small PFO or completely closed PFO) evidence of pulmonary edema is present and the heart size is not as large as that seen in babies with nonrestrictive atrial shunt.
Figure 33.2. Chest roentgenogram in an infant with HLHS demonstrating cardiomegaly and increased pulmonary vascular markings. Reproduced with permission from Neonatology Today 2011;6(12):1–9.
Electrocardiogram
The ECG is also not diagnostic for HLHS in the neonate. Right axis deviation and right ventricular hypertrophy are commonly seen, but not noticeably dissimilar from the ECG of normal newborn. Similarly, decreased left ventricular forces are noted in the left precordial leads (Figure 33.3), but again are not very specific for HLHS. A recent study suggested that wider QRS (68 ± 11 ms), lower S-wave voltages in V1 (1.2 ± 1.5 mV) and higher heart rates (154 ± 17 beats per minute [bpm]) are present in HLHS babies than in normal controls.13
Figure 33.3. ECG of an infant with HLHS showing right axis deviation and right atrial and right ventricular hypertrophy and decreased R waves in leads V6 and V7. Reproduced with permission from Neonatology Today 2011;6(12):1–9.
Echocardiogram
Echocardiogram is a useful test in confirming the diagnosis of HLHS. Hypoplastic LV (Figures 33.4 and 33.5) and aorta (Figure 33.6) as well as enlarged RA, RV (Figures 33.4 and 33.5) and main pulmonary artery (MPA) (Figure 33.7) are easily seen on two-dimensional (2D) imaging.
Figure 33.4. Selected video frame from 2D echocardiographic apical 4-chamber view in a neonate with HLHS showing markedly hypoplastic, slit-like (thick arrow) LV, markedly enlarged and hypertrophied RV and an enlarged RA. The atretic MV (thin arrow) and hypoplastic LALA are also shown.
Figure 33.5. Selected frame from 2D echocardiographic precordial short-axis view in another neonate with HLHS demonstrates a small LV and a large RV. A very small MV (arrow) is also shown.
Figure 33.6. Selected frame from 2D echocardiographic precordial long-axis view of a neonate with HLHS demonstrates a small LV, markedly hypoplastic aorta (Ao) (arrow) and a large RV. LA, left atrium.
Figure 33.7. Selected video frame from 2D echocardiographic apical 4-chamber view in a neonate with HLHS showing hypoplastic LV and markedly enlarged RV and MPA.
The aortic arch and thoracic aorta should be evaluated for evidence of coarctation. The ductus should be evaluated for patency (Figures 33.8). Hemodynamics are assessed by pulsed, continuous wave (CW) and color flow Doppler. Elevated Doppler velocity across the PFO suggests restrictive interatrial communication (Figure 33.9). Doppler examination of the transverse arch reveals retrograde systolic flow (Figures 33.10 and 33.11), which points out ductal-dependent systemic circulation and that the LV is inadequate for consideration of biventricular repair.
Figure 33.8. Selected video frame from 2D (A) and color Doppler (B) precordial short-axis view in a neonate with HLHS demonstrates somewhat constricted DA prior to administration of PGE1. DAo, descending aorta; MPA, main pulmonary artery.
Figure 33.9. Selected video frames from subcostal views of the atrial septum in a neonate with HLHS demonstrating a septal aneurysm (SAnu) (A and B), turbulent flow across the PFO (arrow in B) and a high velocity flow across the PFO (C). Doppler estimated gradient in excess of 10 mmHg (insert in C) and turbulent flow (B) suggest restrictive PFO. LA, left atrium; RA, right atrium.
Figure 33.10. Selected video frame from 2D (A) and color Doppler (B) suprasternal notch views of the aortic arch in a neonate with HLHS. Markedly hypoplastic AAo is shown and serves only to deliver blood in a retrograde fashion to the coronary arteries. Retrograde flow (RF) in the arch of the aorta (B) is suggestive of ductal-dependent systemic circulation. DAo, descending aorta; LSA, left subclavian artery.
Figure 33.11. Doppler interrogation of the DAo (A) and transverse aortic arch (TAA) (B) shows forward flow in the DAo and retrograde systolic flow in TAA; the latter finding indicates ductal-dependent systemic circulation.
Other variants of HLHS such as common AV canal with left ventricular hypoplasia may also be detected on echo studies (Figure 33.12).
Figure 33.12. Selected video frame from 2D echocardiographic apical 4-chamber view in a neonate with common AV canal and hypoplastic left ventricle (LV) with the AV valves closed (A) and open (B). Note the enlarged RA, RV, and a very small LV. LA, left atrium.
Other Imaging Studies
Other studies such as magnetic resonance imaging (MRI) and computed tomography (CT) scan studies are not needed because the echocardiography is adequate to identify most of the abnormalities related to HLHS. However, MRI or CT scan studies may rarely become necessary to more accurately define the PA, aortic arch or pulmonary venous anomalies. Routine head ultrasound to rule out central nervous system abnormality and abdominal ultrasound to assess for renal anomalies are done at most institutions, prior to the Norwood palliation or cardiac transplantation.
Other Lab Studies
Complete blood count, WBC count with differential, electrolytes, BUN/creatinine, liver function tests, arterial blood gases, lactic acid and karyotype may be carried out as necessary.
Cardiac Catheterization and Angiography
2D and Doppler echocardiographic features are amply characteristic so that cardiac catheterization and angiography are not necessary for diagnosis of HLHS in the neonatal period. However, it may be necessary in babies with markedly restrictive interatrial communication requiring transcatheter opening of the atrial septum.14,–17 Such procedures may enlarge or create an atrial septal defect and alleviate left atrial hypertension and pulmonary edema prior to Norwood surgery.2,3,7
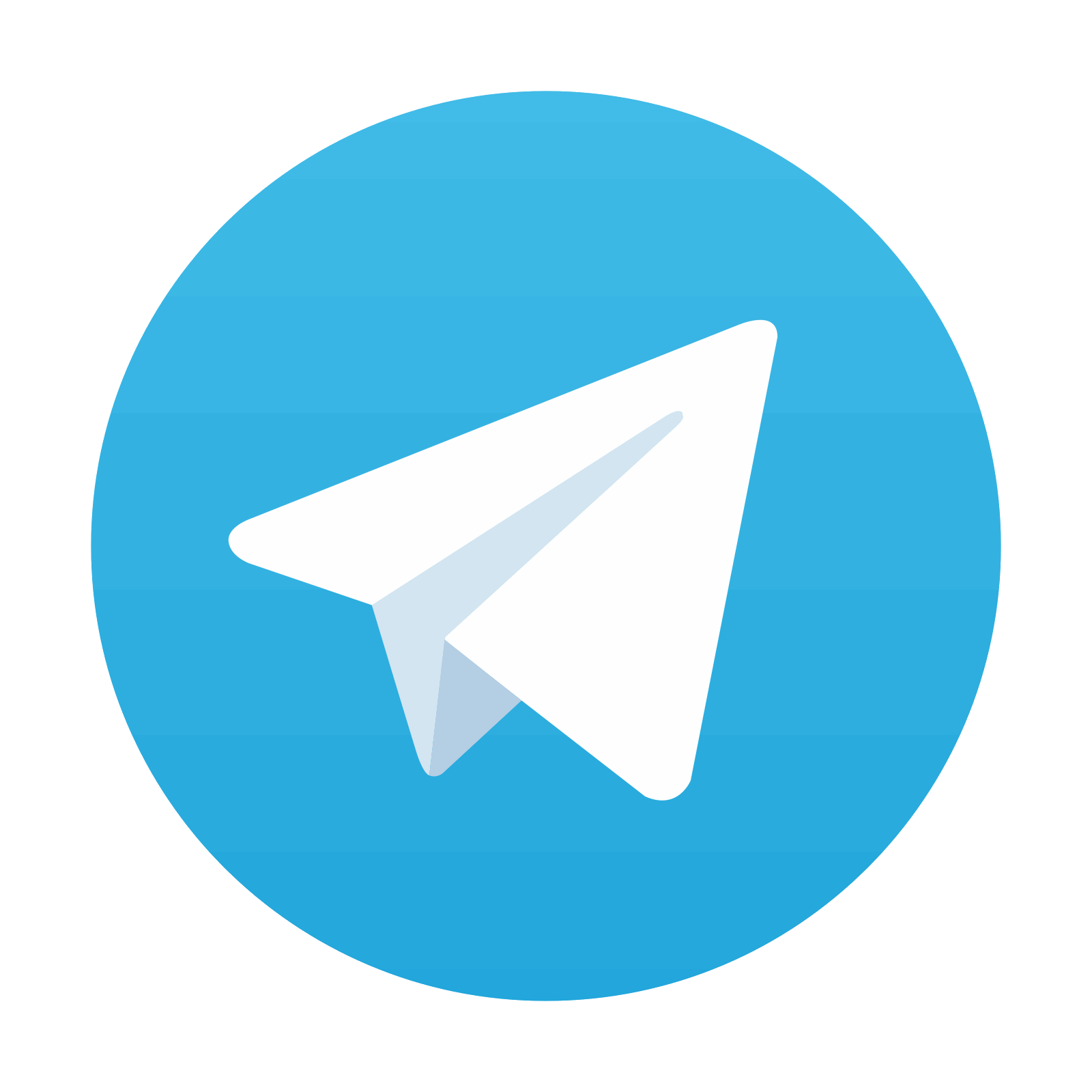
Stay updated, free articles. Join our Telegram channel

Full access? Get Clinical Tree
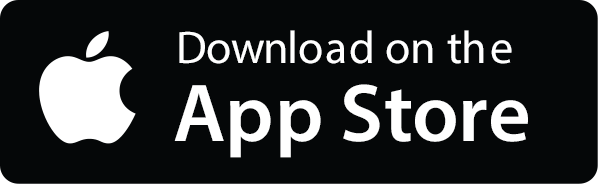
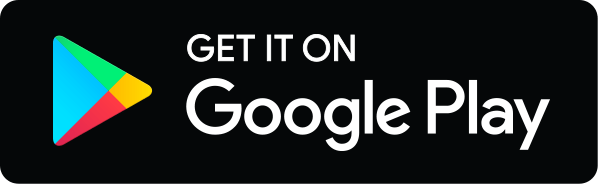