Cardiac involvement affects ≤40% of patients with sarcoidosis and accounts for ≤25% of deaths. The diagnosis of cardiac sarcoidosis is challenging using the existing screening tests and often relies on expensive cardiac magnetic resonance imaging (cMRI) and cardiac 18-fluorodeoxuyglucose positron emission tomography (FDG-cPET). We developed a scoring system using common clinical tests to predict positive imaging findings using cMRI or FDG-cPET. A retrospective chart review of subjects undergoing cMRI or FDG-cPET was performed. The data were extracted and scored using a predetermined system. Our cohort was predominantly white, with a mean age of 55 years, and 60% were women. The scoring system was compared with the findings from cMRI and FDG-cPET to determine the ability to predict the imaging results that define cardiac sarcoidosis. The scoring system for the patients who had undergone both FDG-cPET and cMRI suggested predictability, but the differences were not statistically significant. However, the positive results from just 1 study were as predictive as having positive findings from both studies. A 1-point increase in the total score increased the probability of positive findings from cMRI or FDG-cPET by 14% (95% confidence interval 3% to 25% increase; p = 0.01). The scoring system seemed to be driven more by the findings from cMRI than by those from FDG-cPET. In patients who had undergone cMRI alone, for each 1-point increase in the total score, the probability of positive cMRI findings increased 11% (95% confidence interval 1% decrease to 25% increase, p = 0.08). All screening modalities were analyzed. No modality was sensitive or specific, although major findings (defined in our scoring system) were most predictive of positive imaging findings. In conclusion, commonly available cardiac screening tools used together in a composite score provide reasonable results to predict positive cardiac sarcoidosis findings on imaging, but the system needs refinement. Our data suggest that major findings from screening studies are more likely to correlate with cMRI findings than with findings from FDG-cPET.
Sarcoidosis is a multisystem granulomatous disease affecting 10.9/100,000 whites and 35.5/100,000 blacks. Cardiac involvement occurs in ≤27% of sarcoidosis cases according to autopsy findings, and recent studies have suggested even greater rates of ≤39%. It is the second leading cause of death in patients with sarcoidosis, accounting for 13% to 25% of deaths, and sudden cardiac death can be the first manifestation. Most patients have subclinical disease, because it has been estimated that only 5% will demonstrate clinical cardiac involvement. The current recommendations for screening for cardiac sarcoidosis (CS) include an annual history, physical examination, and 12-lead electrocardiogram. No reference standard is available for the diagnosis of CS. Guidelines for diagnosing CS have been published by the Japanese Ministry of Health and Welfare and have been frequently referenced and followed in diagnosing CS. However, it is not clear whether these guidelines can be generalized to other groups. Cardiac magnetic resonance imaging (cMRI) and cardiac 18-fluorodeoxuyglucose positron emission tomography (FDG-cPET) have been used as the noninvasive diagnostic tests of choice for CS. However, both cMRI and FDG-cPET are expensive, not readily available, and are likely not the best choices for routine screening. We hypothesized that routine screening tests, including electrocardiography, echocardiography, signal-averaged electrocardiography, ambulatory monitoring, nuclear stress testing, and, when available and performed for a clinically indicated reason, an electrophysiology study, combined could be used to predict positive CS imaging findings from cMRI and FDG-cPET. As a secondary goal, we also evaluated the predictive value of each of the tests individually that were used in the scoring system for positive CS imaging.
Methods
The present study was a retrospective, chart review study. After institutional review board approval, we queried our electronic medical record for “International Classification of Diseases, 9th revisions” codes related to sarcoidosis in patients aged ≥18 years who had undergone cMRI and FDG-cPET from January 2008 through July 2010. The subjects were only included in the present study if they had met the American Thoracic Society/European Respiratory Society diagnostic criteria for sarcoidosis. All patients underwent CS surveillance according to each practitioner’s usual care. Basic demographic data, including age, gender, race, and smoking history, were extracted from the medical records. The clinical characteristics were recorded, including biopsy findings, use of immunosuppressant drugs, and the results of cardiac testing (i.e., electrocardiography, ambulatory monitoring, echocardiography, signal-averaged electrocardiography, electrophysiology study, if performed, and cMRI and FDG-cPET) for each patient, when available.
A priori, on the basis of published expert opinion and the clinical experience developed in our sarcoidosis center, we developed, by consensus, a scoring system by assigning a major or minor classification for the clinical tests (electrocardiography, echocardiography, signal-averaged electrocardiography, ambulatory monitoring, nuclear stress testing, and electrophysiology study) to predict whether a subject would have positive CS imaging findings ( Table 1 ). Major findings were assigned 5 points to weight them significantly, and each minor finding was assigned 1 to 3 points according to the schema listed in Table 2 . The total score consisted of the sum of all the minor points plus the number of major findings multiplied by 5 points. Using the clinical data extracted from the medical records, each patient was given a score according to the schema.
Criteria | Major | Minor |
---|---|---|
ECG/ambulatory monitoring | VT Second-degree type II AV block Third-degree AV block | Supraventricular arrhythmia First-degree AV block RBBB LBBB PVC Other AV block Conduction delays |
SAECG | Each abnormal domain | |
Echocardiogram | LV systolic dysfunction (EF <55%) | RV systolic dysfunction Wall motion abnormalities Wall thinning Diastolic dysfunction |
EP study (if performed for a clinically indicated reason) | Monomorphic VT | Polymorphic VT Abnormal voltage mapping Conduction delays |
Myocardial perfusion stress test | Reverse perfusion defect (stress images exhibit greater perfusion than images at rest) |
Point | Description |
---|---|
1 | Diastolic dysfunction Wall thinning RV dysfunction RBBB First-degree AV block Wall motion abnormalities |
2 | Supraventricular arrhythmia LBBB Abnormal SAECG domain Reverse SPECT perfusion defect (stress images exhibit greater perfusion than images at rest) |
3 | Abnormal voltage mapping Conduction delays on EP study |
The results from these clinical tests were used to provide a score to predict positive CS imaging using cMRI and FDG-cPET. The presence of positive findings from either cMRI or FDG-cPET was considered consistent with the presence of CS.
cMRI was performed at our institution using a Siemens 1.5T Avanto (Siemens Healthcare, Malvern, Pennsylvania). Cine and dark blood images were obtained in the short, horizontal, and vertical long-axis planes. After injection of gadolinium (0.2 ml/kg MultiHance [gadobenic acid]), delayed images were obtained at 15 minutes in all 3 planes to evaluate delayed hyperenhancement. FDG-cPET was performed after 24 hours of carbohydrate restriction (serum glucose <120 mg/dl), using a 40-slice Siemens Biograph PET-CT (Siemens Healthcare) with a standard dose of 10 to 13 mCi 18-FDG. Noncontrast, chest computed tomographic and positron emission tomographic images were acquired through the thorax and orthogonal maximum intensity projection images were reviewed for left ventricular activity.
Two reviewers (A.M.F., B.E.F.) independently and in a blinded fashion reviewed 80% of the images for the patients who underwent both FDG-cPET and cMRI. The findings from cMRI were defined as positive when delayed hyperenhancement in a pattern not specifically consistent with a coronary blood flow distribution was noted. The findings from FDG-cPET were defined as positive when patchy increased activity was seen in an otherwise quiet background or markedly increased activity was seen in patches superimposed on low-level background myocardial activity. We did not use other patterns of myocardial 18-FDG uptake, such as diffuse uptake or mild diffuse uptake throughout the myocardium as diagnostic, because these were considered nonspecific. We then performed an intraobserver correlation analysis between the independent readers and the initially reported radiologist interpretation to ensure the accuracy of the results. That analysis showed excellent agreement for the test interpretation between readers (PET, κ = 1.00; MRI, κ = 0.94; 95% confidence interval [CI] 0.83 to 1.00) and with the initial reading (PET, κ = 0.89; 95% CI 0.73 to 1.00; MRI, κ = 0.80; 95% CI 0.59 to 1.00).
Comparisons between the imaging groups were done using a 2-sample permutation test or Fisher’s exact test. The relationship between the total score and the probability of positive findings from MRI or PET was estimated using logistic regression analysis. The SAS/STAT software package, version 9.2 (SAS Institute, Cary, North Carolina) was used for all statistical analyses, except the permutation tests, which used R.
Results
We identified 126 patients who had undergone at least cMRI and/or FDG-cPET and 70 who had undergone both studies. The baseline demographics are listed in Table 3 . Our cohort was predominantly white, with a mean age of 55 years, and 60% were women. Of the 70 patients who had undergone both cMRI and FDG-cPET, 41 (59%) were identified as having ≥1 positive imaging test ( Table 3 ). To test our CS imaging scoring system, each subject’s score was compared with their imaging results. The scoring system provided an incremental value but was not statistically strong enough to predict positive imaging studies when both were performed. For every 1-point increase in the score, the probability of having positive findings from cMRI or from FDG-cPET increased 13% (logistic regression, p = 0.11; 95% CI 3% decrease to 32% increase). When we compared the 14 patients with positive findings from both cMRI and FDG-cPET with the 29 patients with negative findings with each procedure, the probability of positive findings from both cMRI and FDG-cPET increased 14% for each 1-point increase in score (logistic regression, p = 0.23; 95% CI 8% decrease to 40% increase). To understand this nonsignificant trend, an additional analysis was performed.
Variable | Entire Cohort (n = 70) | Positive Imaging (+MRI or +PET; n = 41) | Negative Imaging (−MRI or −PET; n = 29) | p Value ∗ |
---|---|---|---|---|
Age (yrs) | 0.91 | |||
Mean | 55 | 55 | 55 | |
Range | 31–83 | 35–74 | 31–83 | |
Gender (%) | 0.03 | |||
Male | 40 | 51 | 24 | |
Female | 60 | 49 | 76 | |
Race (%) | 0.56 | |||
White | 79 | 76 | 83 | |
Black | 13 | 12 | 14 | |
Other | 9 | 12 | 3 | |
Smoker (%) | 0.22 | |||
Never | 60 | 54 | 69 | |
Past or current | 40 | 46 | 31 | |
Oxygen use (n) | 11 (16) | 7 (17) | 4 (14) | 1.00 |
Biopsy-proven sarcoidosis (n) | 65 (93) | 39 (95) | 26 (90) | 0.64 |
Prednisone use (n) | 41 (59) | 31 (76) | 10 (34) | 0.001 |
Second-line immunosuppressant use (n) | 36 (51) | 27 (66) | 9 (31) | 0.007 |
Drug use at first imaging study † (n) | 19 (27) | 13 (32) | 6 (31) | 0.42 |
Interval between cMRI and FDG-cPET acquisition (mo) | ||||
Mean | 3.9 | |||
Interquartile range | 0.5–14 | |||
Overall range | 0–61 |
∗ Comparisons were done using 2-sample permutation test for age or Fisher’s exact test for all other variables.
For the 70 patients, each cardiac testing modality was compared with a positive imaging result, and the positive and negative predictive values were calculated ( Table 4 ). These tests demonstrated a range of sensitivity and specificity, with greater specificity and positive predictive value noted for the major criteria.
Procedure | n | Sensitivity (%) | Specificity (%) | PPV (%) | NPV (%) |
---|---|---|---|---|---|
Echocardiography | |||||
Any major or minor finding | 64 | 62 | 29 | 60 | 32 |
Major finding (reduced LVEF) | 32 | 32 | 70 | 70 | 32 |
Minor finding (RV systolic dysfunction, wall motion abnormalities, wall thinning, diastolic dysfunction) | 54 | 55 | 33 | 56 | 32 |
Electrocardiography | |||||
Minor finding ∗ | 55 | 58 | 55 | 66 | 46 |
VT or AV block | 30 | 26 | 64 | 56 | 33 |
Ambulatory monitoring | |||||
Any major or minor finding | 47 | 89 | 21 | 62 | 57 |
Major finding | 17 | 73 | 67 | 80 | 57 |
Minor finding | 37 | 85 | 24 | 57 | 57 |
SAECG, any abnormal domain | 49 | 28 | 80 | 67 | 43 |
Nuclear stress testing | 25 | 53 | 50 | 62 | 42 |
EP study | 29 | 40 | 71 | 60 | 53 |
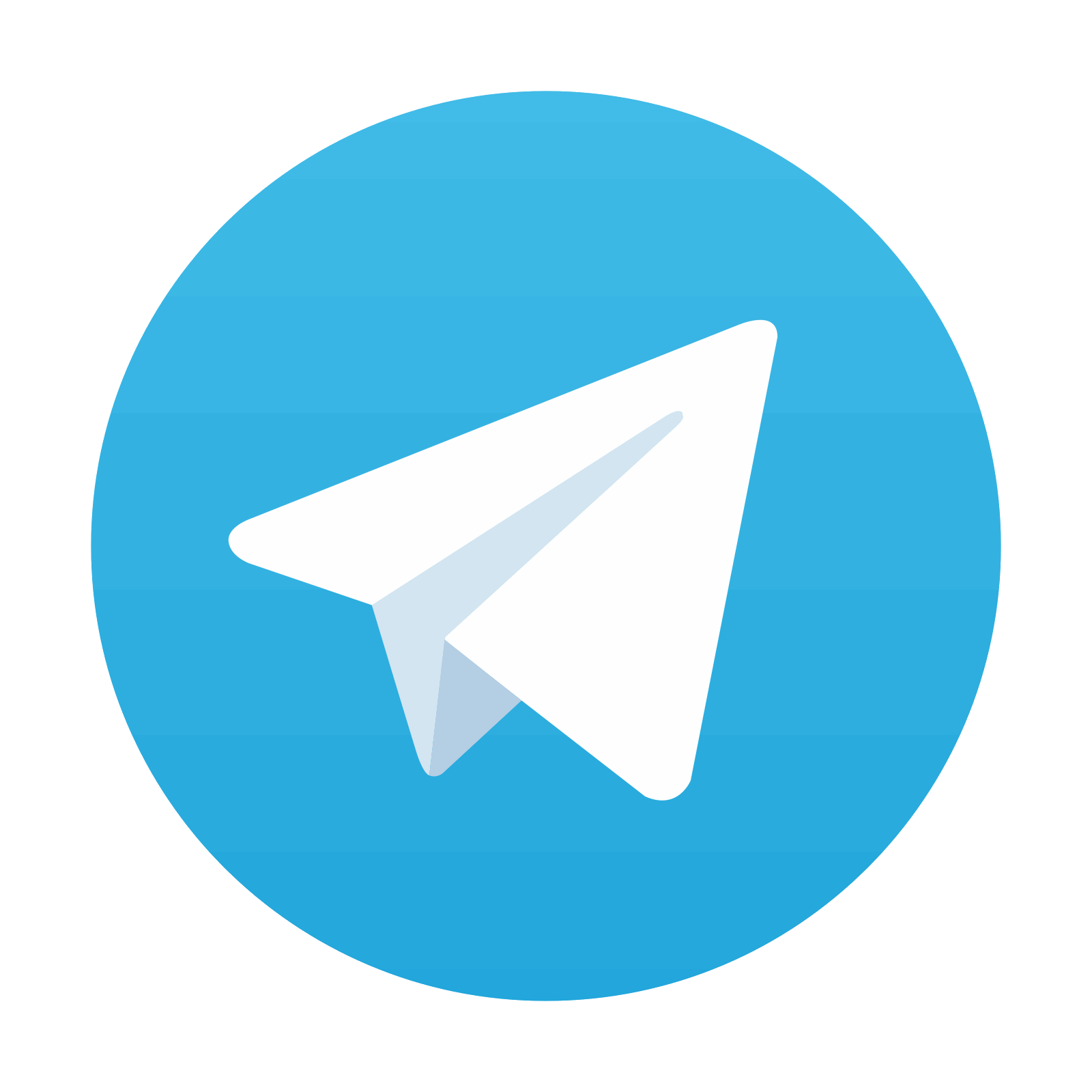
Stay updated, free articles. Join our Telegram channel

Full access? Get Clinical Tree
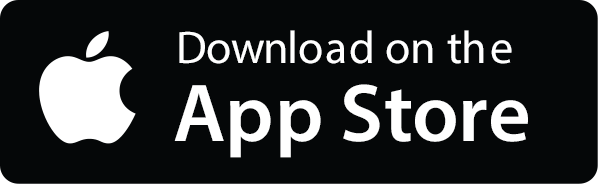
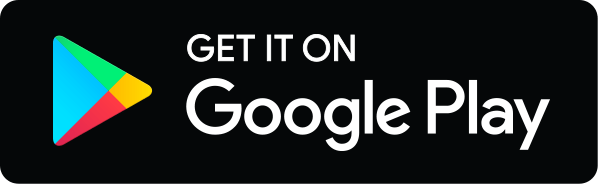
