Fig. 21.1
Decision making algorithm for small samples
Epidermal Growth Factor Receptor (EGFR) Gene Mutations as a Biomarker
The EGFR is a 170-kDa plasma membrane glycoprotein composed by an extracellular region, a single transmembrane domain, and an intracellular domain with tyrosine kinase activity and a C-terminal tail. Activation of the EGFR receptor via phosphorylation produces downstream signals to the phosphatidylinositol 3-kinase (PI3K)/AKT and RAS/RAF/mitogen-activated protein kinase (MAPK) pathways, which are responsible for the normal regulation of cellular proliferation and apoptosis. These intracellular signaling pathways are modified by neoplastic cells with EGFR mutations [13].
The Asia-Pacific patients with NSCLC adenocarcinoma (ADC) subgroup had the highest EGFR mutation frequency at 47%. EGFR-mutated lung cancers represent around 15–20% of all lung ADCs diagnosed in the United States [14], 6–41% in Europe (mean 15%) [15], and 36% (range 14–51%) [16] in Latin America, where there is important heterogeneity.
In the Caucasian population, a mutation of the EGFR gene is more frequent in patients with adenocarcinoma NSCLC with an acinar or papillary pattern (found in 27% of patients); the mucinous adenocarcinoma is usually negative.
Beyond the unequivocal influence of Asian ethnicity, prevalence of EGFR in different ethnic groups is unclear. Some studies have shown no significant difference in EGFR mutation rates between African-American (19%) and white patients (13%), while others suggest a lower frequency of EGFR in African-Americans [17]. Worldwide literature shows that EGFR mutation frequency in lung adenocarcinoma is higher in women than men and in never-smokers versus smokers (66% vs. 22%) [18].
However, the substantial lack of data from several geographic regions of the world (mainly Africa, Middle East, Central Asia, and Central America) and ethnic subgroups limits interpretation.
EGFR 6 (also known as HER-1 or Erb1) belongs to the ErbB receptor tyrosine kinase (RTK) family. This group also includes HER-2/neu (ErbB2), HER-3 (ErbB3), and HER-4 (ErbB4). These transmembrane receptor tyrosine kinases involved in signal transduction pathways regulate proliferation and apoptosis. Known EGFR mutations have been detected in the tyrosine kinase domain of the EGFR gene, which spans exons 18–24. Most mutations are deletions of exon 19 that affect a three-amino acid (LRE) sequence or a point mutation L858R on exon 21 [19]. On the other hand, mutations affecting exon 20, mainly small insertions in T790M (that explain 3–5% of all EGFR mutations), are related with primary resistance to EGFR TKIs. In contrast to the reported higher response rates, better quality of life (QoL), and longer PFS in the whole group of EGFR-mutated ADC compared to wild-type subgroup, a retrospective study in Korean patients with exon 20 insertion reported objective response in only 25% of patients treated with gefitinib [20]. Patients with exon 19 deletion-type EGFR mutation have also shown more significant benefit than patients with L858R mutation. Increased OS (38 months vs. 17 months) has been reported in patients with EGFR exon 19 deletion, compared with patients with L858R mutation [21]. The low frequency of the less common types of EGFR mutations involving exon 18 or 20 has not allowed the definition of the prognostic impact of these genetic alterations.
Based on the available clinical trial data, current guidelines indicate testing all patients with metastatic NSCLC adenocarcinoma for the presence of activating EGFR mutations and recommend first-line EGFR-TKI treatment for patients with adenocarcinoma and a known EGFR mutation [14]. Gefitinib (since 2009 in Europe/Asia and 2015 in the United States), erlotinib (since 2011 in the United States), and afatinib (since 2013 in the United States) have been approved with the condition that, in first-line setting, their use should be restricted to the treatment of lung ADCs with sensitizing EGFR mutations as exon 19 deletions or L858R mutations.
Secondary mutations in EGFR may appear in patients that develop resistance to EGFR TKIs. The most common resistance mutation is the T790M activating point mutation in exon 20 thus interfering with binding of reversible TKIs. Almost 50% of tumors from patients who develop acquired TKI resistance show a T790M, but they may also be found in patients who have never received TKI treatment.
KRAS Mutations
KRAS is a commonly detected mutation in NSCLC, harbored by approximately 20–25% of lung adenocarcinomas in the United States [22] and 14% in France [23]. Most frequently, these mutations involve codon 12 or 13 and, rarely, codon 6 [24].
KRAS mutations are more common in tumors with adenocarcinoma histology than in squamous type NSCLC. KRAS mutations are less common among patients of Asian ethnicity and more common in smokers (43% vs. 0%) [25]. That strong link between cigarette smoking and KRAS mutations in adenocarcinoma of the lung supports some pathogenic role of this mutation in the carcinogenetic properties of tobacco in NSCLA. In small studies, the occurrence of KRAS and EGFR mutations seems to be mutually exclusive [26].
Meta-analyses have shown that patients with KRAS mutations have a poorer response to EGFR-TKIs [27]. Most of the trials were small and this presumed negative association has not been indicated by larger studies [28]. On the other hand, some studies have shown that KRAS mutations have little impact on PFS in contradiction with the presumed nonresponse of KRAS-mutated patients to TKI therapy [29]. According to Cadranel et al., EGFR and KRAS status independently impacts outcomes in advanced NSCLC patients under EGFR-TKI therapy, but EGFR status impacts both PFS and OS while KRAS only impacts OS [23].
An association between KRAS mutational status and benefit of anti-EGFR monoclonal antibodies has not been demonstrated in NSCLC, and no trials can demonstrate the potential value of testing for KRAS mutations and according tailored therapy [30]. As a consequence, the role of KRAS mutations remains unclear. The results of ongoing clinical trials in KRAS-mutant NSCLC will establish if KRAS mutational status should or should not be included in the selection of treatment for NSCLC.
Anaplastic Lymphoma Kinase (ALK)
The oncogenic activity of ALK in anaplastic large cell lymphoma was reported in the 1990s [31]. ALK is a receptor tyrosine kinase normally expressed in several tissues like the small intestine, testes, and the nervous system. In 2007, a translocation in the gene encoding the receptor tyrosine kinase anaplastic lymphoma kinase (ALK), leading to the expression of ALK fusion proteins, was identified as an oncogenic driver in a subset of patients with NSCLC [32]. The genes encoding echinoderm microtubule-associated protein-like 4 (EML4) and ALK are both located on the short arm of chromosome 2 (2p21 and 2p23) [32]. The ALK gene rearrangement consists of a small inversion in the short arm of chromosome 2 between exon 20 of the ALK gene and different exons of the echinoderm microtubule-associated protein-like 4 (EML4) gene, resulting in the abnormal expression and activation of this tyrosine kinase in the cytoplasm of cancer cells. This translocation leads to a chimeric protein with constitutive activation of ALK that presents oncogenic activity demonstrated both in vitro and in vivo. This rearrangement occurs in 2–5% of NSCLC, predominantly in young (50 years or younger), never or light smokers with adenocarcinoma [33] without other genetic disorders, such as mutations of the epidermal growth factor receptor gene [34]. However, clinical characteristics are not as precise as predictive biomarkers to select patients for ALK-targeted therapies. Since the discovery of the ALK-EML4 fusion protein, at least 11 different ALK fusion variants have been identified [35]. Thus, the current guidelines of the International Association for the Study of Lung Cancer (IASLC) and the European Society for Medical Oncology (ESMO) recommend that all patients with advanced-stage lung adenocarcinoma or tumors with an adenocarcinoma component should be tested for ALK, regardless of clinical characteristics.
The presence of alterations in the ALK gene carries a poorer prognosis. It has been reported that patients with ALK translocation have an increased risk of brain and liver metastases (40% vs. 21%) and a greater number of metastatic sites [36] as well as a higher risk of disease progression in comparison with ALK negative patients [37].
Most importantly, ALK gene translocation has been shown to be a predictive biomarker of the efficacy of different agents targeting the ALK kinase activity like crizotinib , ceritinib, and alectinib. Preclinical and single-arm phase I studies have demonstrated that patients with ALK-rearranged NSCLC can be successfully treated with crizotinib. The first published human study of ALK inhibition used the dual ALK/c-MET inhibitor, crizotinib , in patients with ALK-translocated, advanced lung carcinoma [38]. The results of the PROFILE 1007 phase III trial confirmed significantly higher response rates (65 vs. 20%) and longer progression-free survival (7.7 months vs. 3.0 months) compared with chemotherapy as second-line treatment for ALK+ NSCLC [4] and proved better results than standard first-line platinum/pemetrexed chemotherapy in untreated advanced ALK+ NSCLC. Despite the efficacy of crizotinib therapy in patients with ALK-positive lung cancer, most patients develop resistance to crizotinib within the first 12 months [39]. Second-generation ALK inhibitors as ceritinib, alectinib, and brigatinib (AP26113) were developed to overcome crizotinib-resistant mutations. Ceritinib is 5–20 times more potent than crizotinib and has achieved a response rate of 58% and a median PFS of 7 months when tested in ALK-positive patients with a very acceptable profile of adverse effects [40]. These and other research results led to the accelerated approval of ceritinib by the FDA in April 2014 for the treatment of patients with ALK+ metastatic NSCLC with disease progression or who present intolerance to crizotinib. In February 2015, the EMA Committee for Medicinal Products for Human Use (CHMP) adopted a positive opinion, authorizing conditional marketing for ceritinib for patients with advanced ALK+ NSCLC previously treated with crizotinib [41]. Other new-generation ALK kinase inhibitors such as alectinib with potent in vitro activity are showing promising clinical efficacy at extracranial and intracranial sites in ALK-positive NSCLC.
C-Ros Oncogene 1
The c-ros oncogene 1 (ROS1) is a member of the insulin receptor family controlling cell cycling and proliferation. It has been identified as a driver mutation of lung cancer cells and primary tumor tissue and has recently been found rearranged in 2% of patients with NSCLC [42]. Multiple ROS1 fusion proteins have been described. ROS1 rearrangements are rarely accompanied by other mutations such as EGFR, ALK, or KRAS. Patients with ROS1 rearrangement have a very similar profile to those with ALK rearrangement, including a good response to crizotinib. Resistance to crizotinib has already been observed in patients with ROS1(+) NSCLC, and newer agents such as foretinib (GSK1363089) are being studied with initial promising results [43].
Other Molecular Markers
New molecular targets are being described. In October 2015, pembrolizumab was approved for the treatment of PD-L1-positive NSCLC, and the relevance of BRAF mutations in NSCLC by applying BRAF-targeted treatment is being studied. HER-2, human epithelial receptor 2 (HER-2/ErbB2), is a member of the HER group which is activated by homo- or heterodimerization with ErbB1–4 leading to the activation of the PI3K/AKT/mammalian target of rapamycin (mTOR) pathway. There are several ongoing clinical trials exploring newer-generation kinase inhibitors such as dacomitinib, afatinib, and neratinib for HER-2(+) lung cancer.
BRAF mutation —BRAF is a downstream signaling mediator of KRAS which activates the MAP kinase pathway. Small studies have shown that BRAF inhibition with selected TKIs (vemurafenib and dabrafenib) may be effective.
MET abnormalities are a tyrosine kinase receptor for hepatocyte growth factor (HGF). Increased MET expression may predict response to MET-targeted drugs and also appears to be associated with a worse prognosis. Initial studies show that a potential response to crizotinib and capmatinib (an investigational MET inhibitor) may be effective in patients with NSCLC and MET mutations.
Due to the widespread use of next-generation sequencing (NGS) , additional numerous potential targets will be developed in the next years, but only clinical trials will show which will be appropriate for clinical application.
Optimizing Tissue Sampling for Histologic and Molecular Analysis of Lung Cancer
Most lung cancer patients are diagnosed after examination of a small biopsy (transbronchial or endobronchial) or cytology (fine-needle aspiration or body fluid) specimen. Specimens are processed for diagnosis, staging, and molecular marker analysis. As personalized treatment is now the standard of care for advanced lung cancer, current guidelines recommend that rapid EGFR and ALK mutation testing be performed at diagnosis in all patients with advanced adenocarcinoma regardless of gender, race, smoking history, or other risk factors. Testing is not recommended for tumors without an adenocarcinoma component. However, it should also be considered in large cell carcinoma or poorly differentiated carcinoma because limited sampling does not effectively exclude an adenocarcinomatous component [44].
Molecular testing is essential for patients with unresectable advanced lung cancer who will be offered targeted therapies. Patients with a potential surgical cure may never need antineoplastic drugs. The initial surgical specimen at resection is a high-quality tissue sample and may avoid new biopsies in case of relapse. However, the cost-benefit ratio of potential unnecessary testing must be considered.
If molecular testing of lung cancer is easily available and adequately reimbursed, shortening the waiting time for treatment selection seems reasonable. The sequential performance of molecular testing may be justified in the case of financial constraints that exist in molecular testing. In those cases, starting with KRAS in Caucasian and EGFR in Asian patients may be reasonable. Alternatively, IHS screening for ALK- or ROS1-negative lung cancer may be performed to detect cases that require FISH testing for ALK or ROS1 translocations. Sequential molecular testing of lung cancer can reduce the volume and cost of analysis by roughly 30%; however, this must be offset with the delay in obtaining the necessary information for the right treatment choices.
Early studies [45] have shown discordance in the results of mutational analysis between primary tumor and metastatic disease. However, the current consensus is that discordance in the rates of mutation detection between primary or metastatic tumors is infrequent and consequently the primary or metastatic site can be tested [46].
On the other hand, there is no evidence of intratumoral heterogeneity of mutations in NSCLC, and current data suggest that it is not necessary to test multiple areas of the same tumor. Synchronous primary tumors should be tested independently as different tumors. Molecular analysis of synchronous lesions may contribute to define if lesions are likely to be clonally related.
Despite the multiple biomarkers mentioned above, tissue should be prioritized for EGFR and ALK testing. The cautious use of material should be taken into consideration to avoid new invasive diagnostic procedures.
Different Assay Technologies for Histologic and Molecular Diagnosis
The current classification of lung adenocarcinoma calls for more frequent use of IHC. At least 20–30% of tumor samples cannot provide an accurate diagnosis using routine histology or cytology and require the additional use of IHC [47]. Consequently, most guidelines recommend privileging biopsies over cytology since the former can provide more appropriate material for morphological and molecular diagnosis [48]. The use of FFPE tissue slides obtained from small biopsies (as those obtained by bronchoscopy) is usually sufficient for IHC. The cell block technique requires more cells than simple smears but offers better quality for diagnosis.
For molecular analysis, a variety of diagnostic assays can be used to identify abnormalities.
IHC shows poor or no correlation with EGFR mutations; therefore, it is not considered an acceptable test for EGFR TKI treatment selection. IHC is being explored in regard to overexpression of ALK. Though IHC was not initially considered reliable for ALK rearrangement screening, the development of new monoclonal antibodies has increased its potential [49]. The sensitivity of these new methods (if properly validated) is considered high enough, and the current recommendation is that tumors that fail to demonstrate ALK immunoreactivity with the new IHC methods need not be tested by FISH. However, tumors that test positive for ALK IHC should still be referred to FISH since there is no evidence yet for the use of ALK IHC as a sole determinant for ALK TKI therapy.
FISH is a widely used technology for the detection of gene fusions in lung cancer. It may be performed on tissue, cytology cell block, or smears. It detects genomic and chromosomal changes through hybridizing labeled DNA probes to a sample. Those fluorescently labeled DNA probes bind to specific parts of the chromosomes and thus may be visualized by fluorescence microscopy [50]. FISH is not a validated methodology for assessing patients for EGFR mutational status. Guidelines do not recommend EGFR copy number analysis (i.e., FISH or chromogenic in situ hybridization) for selection of EGFR TKI therapy because EGFR copy number testing (by FISH or chromogenic in situ hybridization) is less predictive than mutation testing [51]. On the other hand, published evidence indicates that FISH assay is acceptable for treatment selection with an ALK TKI. The assay is considered positive for ALK rearrangement if more than 15% of tumor cells have split green and red signals.
There are several extraction methods of DNA, and there is no consensus on which is the best. There are also several methods to detect EGFR mutations including direct sequencing, the amplification refractory mutation system (ARMS) , length analysis, and denaturing high-performance liquid chromatography. Direct DNA sequencing is performed by different commercially available mutation-specific kits. Direct sequencing of PCR-amplified regions is a very common method for identifying EGFR and KRAS mutations [52].
The 2013 guideline from the College of American Pathologists, International Association for the Study of Lung Cancer, and Association for Molecular Pathology does not recommend any particular method but emphasizes the need that laboratories validate their methods for acceptable quality [51]. EGFR test methods should detect mutations in specimens with at least 50% cancer cell content. If a laboratory cannot perform these more sensitive tests, it should share that limitation with the lung cancer team so that clinicians know clearly on what grounds they are making their decisions.
The serial mutational tests are time-consuming, and multiplex testing is being explored in the last years. The cost of multiplexing is higher than conventional serial mutation testing. New platforms such as Sequenom or SNaPshot allow for detection of multiple genomic abnormalities with sequencing, and some of them (like Foundation One) also incorporate detection of gene rearrangements as EML4-ALK and changes in gene copy number. Currently, high costs and amounts of tissue required are limiting factors, but the constant developments in these technologies may make them more easily available and affordable in the next future.
In some countries, there are accreditation protocols to certify laboratories with appropriate quality control standards. Even in the absence of official accreditation bodies, only laboratories that are able to comply with standards should carry out this type of molecular testing. It is recommended that different laboratories share protocols and exchange positive and negative control samples to improve their performance by an external quality control. The availability of such laboratories is limited, which complicates the coordination among teams and the integration of all available data. The workup proposed by the IASLC-European Thoracic Oncology Platform multidisciplinary workshop suggests that the final results (including the molecular testing) should be available in 1 week. In the daily life scenario, that may be possible only for a few selected big academic centers but is not for smaller community hospitals or facilities that refer the molecular testing outside the original institution. In any case, the process should not take longer than 2 weeks. The achievement of this goal usually requires a huge effort for smaller institutions which must organize multiple teams from pathologists, oncologists, pulmonologists, and thoracic surgeons in addition to reference laboratories.
Minimal Requirements for Specimens Used for Molecular Analysis
Since the different techniques [immunohistochemistry, molecular diagnostics, fluorescent in situ hybridization (FISH) ] require a predetermined minimal cell number and a tumor-cell-to-normal cell ratio of the sample [53], the main initial goal is to obtain a high-quality, high-volume tumor specimen, which would largely depends on the biopsy technique but also on the optimization of the available tissue by a correct processing technique.
Handling of tumor specimens has to be standardized in each institution through fluent communication between clinicians and pathologists. In general, required specimens are formalin-fixed, paraffin-embedded (FFPE) samples or fresh, frozen, or alcohol-fixed ones for polymerase chain reaction (PCR)-based EGFR mutation tests. Other tissue treatments such as acidic or heavy metal fixatives or decalcifying solutions should not be used in specimens that will be sent for EGFR testing. The most widely used fixative is 10% neutral-buffered formalin. The fixation time should be as short as possible yet sufficient to permit diagnosis. Best results have been rendered with 6–12 h fixation time for small biopsy samples and 8–18 h for larger surgical specimens. For other techniques, such as DNA extraction and polymerase chain reaction (PCR) , the optimal fixation times have yet to be established [54].
Cytology specimens such as malignant pleural effusions may be useful. Cell blocks are strongly recommended over smear preparations because they correlate with malignant cell content. Recent research has shown that cytology smears can be effectively used to detect ALK gene rearrangements using FISH [55]. However, tissue biopsy samples are preferred because there is not enough evidence to accept the clinical reliability of mutational data obtained from cytology.
The minimum number of tumor cells required for adequate mutation testing is not fully determined. Although an ideal sample should contain at least 200–400 tumor cells, it is not easy to obtain this type of specimen in routine clinical practice. The performance of DNA sequencing requires at least 50% of tumor cells. As mentioned above, more sensitive techniques allow reliable results with as little as 10–20% of tumor cells. More sophisticated next-generation sequencing technologies are being developed, and probably, in the near future, 5% of tumor cells will be sufficient for reliable results.
Recommendations for Handling the Diagnostic Material for Histologic Examination and Molecular Analysis
The different diagnostic tools available for the study of lung cancer (transthoracic needle aspiration, bronchoscopy, etc.) will mainly provide two different kinds of specimens: cytology and biopsies. Each requires careful processing considerations in order to preserve the obtained material and optimize its diagnostic value.
Cytology Specimens
The presence of a pathologist at the moment of retrieval of the specimen is essential to qualify its appropriateness. The material should be examined in situ to assess the presence of blood, necrotic areas, and small tissue fragments and granular material suitable for ulterior examination. In the presence of carcinomatous tissue, the granular material is often grossly visible. The rapid on-site evaluation or ROSE has shown to increase the diagnostic yield [56]. If the cytopathologist notifies that there is not sufficient or adequate material under microscopic examination, additional passes should be performed to obtain additional samples. A meta-analysis demonstrated that ROSE has a sensitivity of 80–88% in EBUS-TBNA without increasing procedure length [57]. Although some data are encouraging about the ability of ROSE to optimize cell aspiration procedure and ensure that sufficient material is collected for cytologic diagnosis and for cell block preparation, the effect of ROSE itself has not been adequately studied, and evidence has been considered insufficient to recommend its use in every procedure by the WABIP evidence-based guideline [58].
Slides are usually prepared by the cytopathologists, but the operator performing the procedure (surgeon or pulmonologist) may be in charge of that step according to the organization of each working team. The slides are prepared generally with Diff-Quik (DQ)-stained air-dried smears, H&E/Pap-stained alcohol-fixed smears, or Pap-stained ThinPrep monolayer slides with cells transferred in suspension. If possible, H&E-stained cell blocks (paraffin-embedded cell pellets prepared using centrifugation of CytoLyt fluid after the addition of HistoGel) are prepared. All those procedures must be standardized according to the routine of each anatomic pathology laboratory.
Special care is required for the samples obtained by EBUS. The bronchoscopist has the greatest responsibility in that scenario, as he is the one coordinating a more complex team of different parties.
Technical aspects of sample retrieval are important. The diagnostic yield of conventional TBNA appears to be better when 19-gauge needles are used, instead of 22-gauge needles. Concerning EBUS, retrospective studies have failed to find a difference in the overall diagnostic yield between 22- and 21-gauge needles. On the other hand, the number of aspirates per lymph affects the diagnostic yield, quantity, and quality of the obtained specimen (Fig. 21.2). There is enough evidence that three aspirations with EBUS-TBNA and three to four aspirations with conventional TBNA provide near the maximum yield, higher than 90% [58]. There is no evidence that the type of needle, use of miniforceps, suctioning and type of sedation, time spent inside the node, and a number of revolutions inside the node have any influence on the ulterior molecular test.


Fig. 21.2
EBUS-TBNA and cTBNA have a similar yield for specimen adequacy of driver mutations
Once obtained, the aspirates are placed onto a glass slide. Such procedure may be performed by blowing air with a syringe through the needle or replacing the stylet. Saline is then poured with a syringe through the EBUS needle into CytoLyt solution to obtain any remaining cells. Some of the resulting material will be whitish tissue particles that should be separated from the blood/mucus and smeared on at least two different slides. The remaining material on the original slide is used to prepare a clot. One of the two slides should be prepared with the white material, and the other is air-dried for ROSE (usually stained with DQ stain or Giemsa) and placed in 95% ethanol for Pap staining which will enhance nuclear cytological detail [59]. The collection of fresh material and the clot should be encouraged. The main advantage of direct smears over cell block for molecular analysis is the possibility of immediate analysis to assess specimen adequacy and amount at the time of retrieval. When collection of tissue core is possible, it should be placed on filter paper to eliminate the excess of blood and fixed with 10% neutral buffered formalin. The FFPE sample is later stained with H&E for histologic diagnosis and IHC.
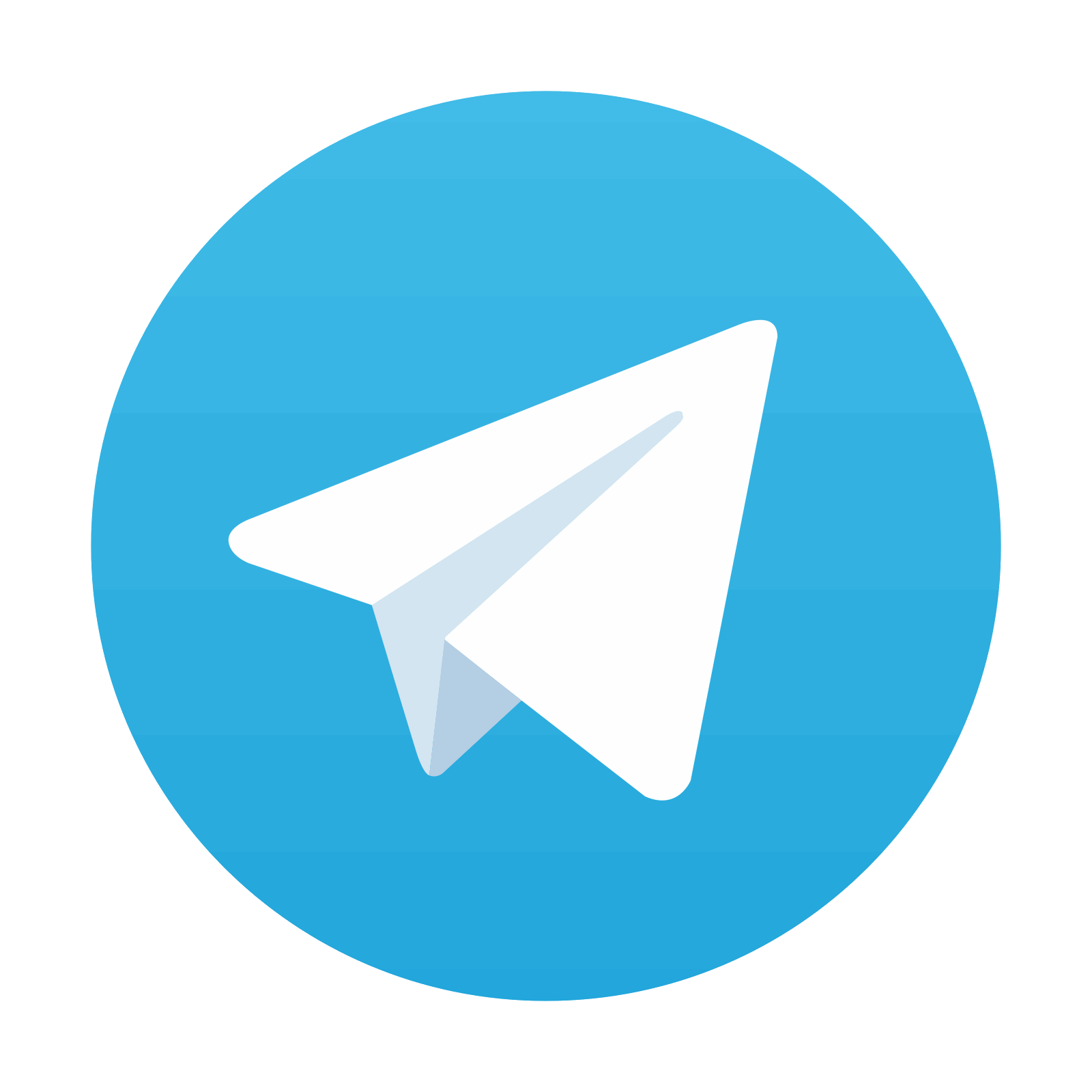
Stay updated, free articles. Join our Telegram channel

Full access? Get Clinical Tree
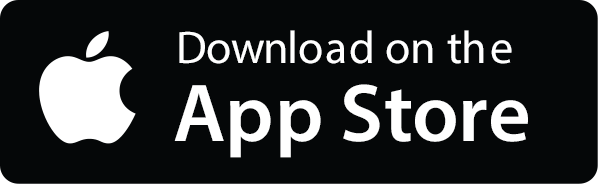
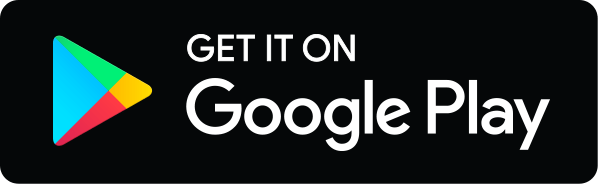