Modality
NLST
PanCan
Diagnosticmethod (%)
Positiverate (%)
Diagnosticmethod (%)
Positiverate (%)
Bronchoscopy
34
55.5
20
55.6
CT-FNA/core
19
66.5
38
81.1
Surgery
47
73.9
42
77.6
History and Historical Perspective
Optical coherence tomography (OCT) was originally developed for noninvasive cross-sectional imaging of biological systems [14, 15]. This optical imaging method offers near histologic resolution for visualizing cellular and extracellular structures at and below the tissue surface up to 2–3 mm. The utility of this imaging modality was first demonstrated in ophthalmology and cardiology [16, 17]. It was later developed as an optical imaging and biopsy tool in other organs such as the esophagus and lung [18–21].
OCT is similar to B-mode ultrasound. Instead of sound waves, light waves are used for imaging. Optical interferometry is used to detect the light that is scattered or reflected by the tissue to generate a one-dimensional tissue profile along the light direction. By scanning the light beam over the tissue, two-dimensional images or three-dimensional volumetric images can be recorded. For bronchoscopic application, the imaging procedure is performed using fiber-optic probes that can be miniaturized to enable imaging of airways down to the terminal bronchiole. These probes can be inserted down the instrument channel during standard bronchoscopic examination under conscious sedation. The axial and lateral resolutions of OCT range from approximately 5 to 30 μm, and the imaging depth is 2–3 mm depending on the imaging conditions. This combination of resolution and imaging depth is ideal for examining changes originating in epithelial tissues such as airways. Unlike ultrasound, light does not require a liquid coupling medium and thus is more compatible with airway imaging. There are no associated risks from the weak near-infrared light sources that are used for OCT.
In time domain OCT, a depth-resolved line profile of tissue is obtained by measuring the autocorrelation function [14, 22] using a low-coherence time light source and an interferometer comprised of a variable-length reflective reference arm and a sample arm where the tissue is illuminated. A signal is generated when the path length of light scattered from a particular tissue depth matches that from the reference arm. In frequency domain OCT, the spectral density function is measured to obtain a depth-resolved optical scattering of the tissue through Fourier transformation. The spectral density function can be measured with interferometers using either a broadband light source and a spectrometer or a wavelength-swept light source and a square-law detector. This approach was shown to provide orders of magnitude enhancement in detection sensitivity compared to time domain OCT [23–27].
In Doppler OCT , the energy of photons from a moving system is transformed according to the four-vector momentum and the Lorentz transformation. According to the special theory of relativity, the energy of photons emitted from an object moving relative to an observer is transformed the same way leading to different energies compared to those seen by an observer that is stationary relative to the photon source. These different energies that correlate with different frequencies are called Doppler effect that can be used to detect moving sources by measuring a change in the frequency of the optical field emitted from the source. The OCT signal contains the information about the phase of the optical field scattered from a tissue sample. Therefore, moving objects can be detected by evaluating frequency shifts in their OCT signals [28, 29]. This technique can be used to visualize pulmonary vasculature in vivo during endoscopic imaging [30]. Doppler signals are created by analyzing the OCT data stream using the Kasai velocity estimator to evaluate the Doppler phase shift between A-scans in each frame. Endoscopic Doppler OCT can be difficult due to the motion artifacts such as from cardiac pulsations and breathing movement. Bulk tissue motion correction algorithms are used to reduce artifacts.
Polarization-sensitive OCT (PS-OCT) is another extension to OCT to improve detailed tissue differentiation. By analyzing the polarization state of backscattered light, PS-OCT can provide information about tissue birefringence, diattenuation, optical axis orientation, and depolarization. Using PS-OCT, highly organized, anisotropic tissue layers such as muscles, bones, and blood vessel walls can be identified by their innate birefringence. Clinical applications of PS-OCT have been demonstrated in the determination of burn depth in vivo [31], the measurement of collagen and smooth muscle cell content in atherosclerotic plaques [32], the differentiation of benign lesions from malignant lesions in the larynx [33], and the detection of nerve fiber bundle loss in glaucoma [34, 35]. Obtaining polarization-dependent optical properties of tissue with PS-OCT entails two essential requirements. Firstly, the incident light on the tissue needs to have known polarization states (commonly circular polarization) [36, 37] or multiple sequential polarization states (not necessarily known) with defined polarization relation between them [38, 39]. Secondly, the polarization state of light scattered from tissue needs to be detected using a polarization diversity detection scheme. Polarization-sensitive detection can also be used to reduce the effects of polarization in structural OCT imaging that uses rotary probes. As the spinning fiber-optic probe is continuously flexing and in motion, the polarization state of the light exiting the tip of the probe is constantly varying, creating artificial intensity variations during OCT imaging. These variations can be significantly reduced using polarization diversity detection [40].
A recent advance in OCT imaging is co-registered autofluorescence OCT (AF-OCT) [41]. Autofluorescence imaging makes use of fluorescence and absorption properties to provide information about the biochemical composition and metabolic state of endogenous fluorophores in tissues [42, 43]. Most endogenous fluorophores are associated with the tissue matrix or are involved in cellular metabolism. The most important fluorophores are structural proteins such as collagen and elastin and those involved in cellular metabolism such as nicotinamide adenine dinucleotide (NADH) and flavins [43]. Upon illumination by violet or blue light (380–460 nm), normal tissues fluoresce strongly in the green (480–520 nm). Malignant tissues have a markedly reduced and redshifted autofluorescence signal due to the breakdown of extracellular matrix components as well as increased absorption by blood. These differences have been exploited to detect preinvasive and invasive bronchial cancers in central airways [44]. AF-OCT overcomes the limitation of autofluorescence bronchoscopy because the OCT imaging probes are much smaller than flexible video bronchoscopes allowing access to small peripheral airways beyond bronchoscopic view. AF-OCT allows rapid scanning of airway vasculature less prone to motion artifacts compared to Doppler OCT [45].
Endoscopic AF-OCT System
Figure 16.1 illustrates the components of an AF-OCT prototype system is shown in Fig. 1, and an AF-OCT prototype is shown in Fig. 16.1. A Mach-Zehnder interferometer driven by a wavelength-swept source comprises the OCT subsystem (Fig. 16.2a). The AF subsystem uses a 445 nm excitation laser and a photomultiplier tube for the detection of autofluorescence emission. Endoscopic imaging of airways is implemented using fiber-optic catheters that scan in a rotational manner using proximal motors. A large-scale motor actuates the rotor of a fiber-optic rotary joint (FORJ) that is connected to an imaging catheter, enabling proximally driven rotational scans of the catheter’s fiber assembly. The imaging catheter consists of a double-clad fiber (DCF) catheter. This fiber assembly is fixed inside a torque cable that transfers rotational and pullback motions from the proximal end to the distal end (Fig. 16.2b). The rotating assembly is placed inside a close-ended 900 μm diameter stationary plastic tube if the catheter is going to be reused.



Fig. 16.1
Illustrates the components of an AF-OCT prototype system

Fig. 16.2
Schematic diagram of OCT and AF-OCT. (a) OCT, (b) inner cladding AFI excitation, (c) core AFI excitation subsystems, and (d) optical elements at the tip of the DCF catheter. DM dichroic mirror, ExF excitation filter, EF emission filter, PMT photomultiplier, WDM wavelength division multiplexer, DCFC double-clad fiber coupler, FORJ fiber-optic rotary joint, DCF double-clad fiber, MMF (step-index) multimode fiber, GRIN graded index fiber, NCF no-core fiber
In one configuration (Fig. 16.2b), the AF excitation light is coupled to the DCF inner cladding [41], and in another system configuration (Fig. 16.2c), the AF excitation light is coupled to the DCF core using fused fiber components [46–48] and a custom-designed FORJ [49]. The latter allows a tightly focused AF excitation light that exits the catheter, enabling higher-resolution AF imaging.
Preclinical Studies
Ex vivo studies have shown that OCT can visualize structural features in airways, adjacent alveoli, and pulmonary nodules that correspond closely to the histopathology (Fig. 16.3) [20, 50–54]. The basement membrane can be clearly seen between the epithelial and submucosal layer. Cartilage usually appears as darker signal-poor regions due to its low scattering properties. OCT measurements of mean luminal diameter, inner luminal area, airway wall area, and percent airway wall thickness prior to surgical resection were found to correlate significantly with the histology down to the ninth-generation bronchi in the resected specimens [55].


Fig. 16.3
Correlation of OCT image with histopathology in porcine airway. Black = nuclei, elastic fibers; yellow = collagen, reticular fibers; blue = ground substance, mucin; bright red = fibrin; red = muscle
Clinical Studies
Endoscopic OCT imaging is performed during flexible bronchoscopy under local anesthesia applied to the upper airways and conscious sedation [21, 56]. The OCT probe can be inserted inside a guide sheath similar to radial endobronchial ultrasound through the working channel of the bronchoscope into the targeted airways. When clinically indicated, following removal of the catheter, histological and/or cytological samples are collected. OCT imaging adds about 5–10 min to the standard procedure time. It is usually well tolerated by patients. Repeat OCT measurements of airways were found to be reproducible and hence can be used for longitudinal assessment of changes in airway morphology [57].
Lung Cancer
The ability of OCT to discern invasive cancer versus CIS or dysplasia was investigated [21]. Normal or hyperplasia is characterized by one or two cell layers above a highly scattering basement membrane and upper submucosa. As the epithelium changes from normal/hyperplasia to metaplasia, various grades of dysplasia, and CIS, the thickness of the epithelial layer increases; quantitative measurement of the epithelial thickness showed that invasive carcinoma is significantly thicker than carcinoma in situ (p = 0.004) and dysplasia is significantly thicker than metaplasia or hyperplasia (p = 0.002). The nuclei become more readily visible in high-grade dysplasia or CIS. The basement membrane is still intact in CIS but became discontinuous or no longer visible with invasive cancer [21]. Squamous cell carcinoma has different OCT features than adenocarcinoma [52, 53] (Fig. 16.4).


Fig. 16.4
(a) OCT and histological image of a squamous cell carcinoma showing the in situ component and invasion through the basement membrane (arrows). (b) AF-OCT of an adenocarcinoma with lepidic growth. In the AF image, there is a loss of green autofluorescence
The morphology of the peripheral lung nodules has been characterized. Lung parenchyma can be identified by the presence of signal-void alveolar spaces that appear as a honeycomb-like structure. Pulmonary nodule is identified by replacement of alveoli with solid tissue [45, 58, 59]. Adenocarcinomas with lepidic growth pattern are recognized by their thickened alveolar walls [45] (Fig. 16.4). After OCT interpretation training sessions, clinicians can diagnose common primary lung cancers (adenocarcinoma, squamous cell carcinoma, and poorly differentiated carcinoma) with an average accuracy of 82.6% (range 73.7–94.7%) [60]. Although OCT cannot replace histology in the diagnosis of lung carcinoma, it has the potential to aid in diagnosing lung carcinomas as a complement to tissue biopsy, particularly when insufficient tissue is available for pathology assessment. OCT may be useful for confirming the nature of the lesion before taking a biopsy. Since OCT probes can be miniaturized, they can be inserted inside biopsy needles/catheters to guide biopsy under real time without removing the imaging probe from a guide sheath and reinserting the biopsy forceps or needle with the possibility of displacement or migration to a different airway [56].
Asthma
It is known that asthma phenotypes are heterogeneous and influence the response to treatment. Bronchial thermoplasty (BT) is a non-pharmacologic method to treat patients with chronic persistent asthma [61]. Currently, there is no method to select patients who will benefit from BT. OCT imaging was performed in two patients with chronic persistent steroid-dependent asthma prior to and immediately after bronchial thermoplasty as well as at 3 weeks, 6 weeks, 6 months, and 2 years after bronchial thermoplasty. Prior to BT, distinct asthma phenotypes were observed between the patient (patient A) who had sustained benefit from BT for over 2 years and the one who did not (patient B) (Fig. 16.5) [62]. PS-OCT [36, 63, 64] that can define highly organized tissue layers such as smooth muscle and collagen may be a useful non-biopsy tool to study the effect of pharmacologic and non-pharmacologic therapies.


Fig. 16.5
OCT images of two patients before bronchial thermoplasty (BT) illustrating different phenotypic features. (a) Long-term responder following BT; (b) nonresponder with BT. EPI epithelium, BM basement membrane, SM smooth muscle
Chronic Obstructive Pulmonary Disease
Chronic obstructive pulmonary disease (COPD) is a heterogeneous disease characterized by both small airway and parenchymal abnormalities. There is increasing evidence to suggest that these two morphologic phenotypes, although related, may have different clinical presentations, prognosis, and therapeutic responses to medications. A recent ex vivo study using micro-CT showed that narrowing and disappearance of small conducting airways occur prior to the onset of emphysematous destruction and that these changes can explain the increased peripheral airways resistance reported in COPD [65]. Clinical CT using an acceptable dose of radiation provides airway images up to the fifth generation. Unfortunately, the resolution of CT is not adequate to image critical events that begin at the seventh branching generation nor can it measure morphological changes in different layers of the airway wall. OCT can overcome this limitation with small optical probes that can image airways as small as terminal bronchioles with high resolution [55, 65, 66]. Coxson et al. compared OCT measurements with CT scans and lung function in COPD patients [67]. In 44 current and former smokers, OCT imaging was used to measure the airway dimensions in specific bronchial segments. These data were compared with CT measurements of the exact same airway using a three-dimensional reconstruction of the airway tree (Pulmonary Workstation 2.0; VIDA Diagnostics, Inc., Iowa City, IA). A strong correlation between CT and OCT measurements of lumen and wall area was observed. The correlation between FEV1%-predicted and CT- and OCT-measured wall area (as percentage of the total area) of fifth-generation airways was good for both imaging modalities, but the slope of the relationship was much steeper using OCT than using CT, indicating greater sensitivity of OCT in detecting changes in wall measurements that relate to FEV1. They concluded that OCT is more sensitive for discriminating the changes in the more distal airways of subjects with a range of expiratory airflow obstruction compared with CT. In addition to airway wall remodeling, alveolar wall destruction in COPD can also be clearly visualized using OCT with the emphysematous alveoli appearing as large voids compared with the small alveoli seen in those with normal lung function [53] (Fig. 16.6).


Fig. 16.6
OCT image of terminal bronchiole and adjacent alveoli. (a) Normal bronchiole; (b) patient with moderate emphysema; (c) patient with severe dysplasia showing progressive destruction of alveolar walls
Sex differences in airway remodeling in COPD have also been investigated using OCT to help understand why women have a 50% increased risk of COPD compared with men after adjustment for the amount of smoking. Female human smokers have significantly thicker airway walls compared to male human smokers similar to the changes in a mouse model of COPD [68].
Airway and Lumen Calibration
Airway diameter measurement via bronchoscopy is not reliable due to optical distortion of the bronchoscope lens that varies among bronchoscopes and limited ability to gauge depth. Respiratory motions interfere with airway measurement. Airway measurement can be performed using CT scans. However, real-time examination is not always available, and radiation exposure is a concern. Using phantoms, excised pig airways, and in vivo human airways during bronchoscopy, Williamson et al. demonstrated that airway measurements using anatomic OCT are accurate and reliable and compare favorably with CT imaging [69]. OCT was used to measure airway diameter in patients with subglottic tracheal stenosis, main bronchial stenosis, and tracheomalacia. The real-time OCT information was found to be helpful for determining the length of the stenosis, extent of tumor involvement beyond the bronchoscopic view, and severity of the tracheomalacia or guide the choice of airway stent. The investigators conclude anatomic OCT with conventional bronchoscopy allows accurate real-time airway measurements and may assist bronchoscopic assessment [70].
Obstructive Sleep Apnea
Changing of the upper airway sizes during sleep is the key pathophysiologic change in patient with obstructive sleep apnea (OSA) . Reduction in pharyngeal size correlates with increased sleep disorder breathing and degree of nocturnal desaturation [71]. CT scan has been used to measure the upper airway size. However, measuring upper airway dimension during sleep and awake with CT is not practical plus concern with radiation exposure. Anatomic OCT offers a real-time quantitative measurement of the upper airway shape and size during sleep or awake comparable to CT scan [72]. Individuals with OSA were found to have a smaller velopharyngeal cross-sectional area than BMI-, gender-, and age-matched control volunteers, but comparable shape suggesting it is an abnormality in size rather than shape that is the more important anatomical predictor of OSA [73].
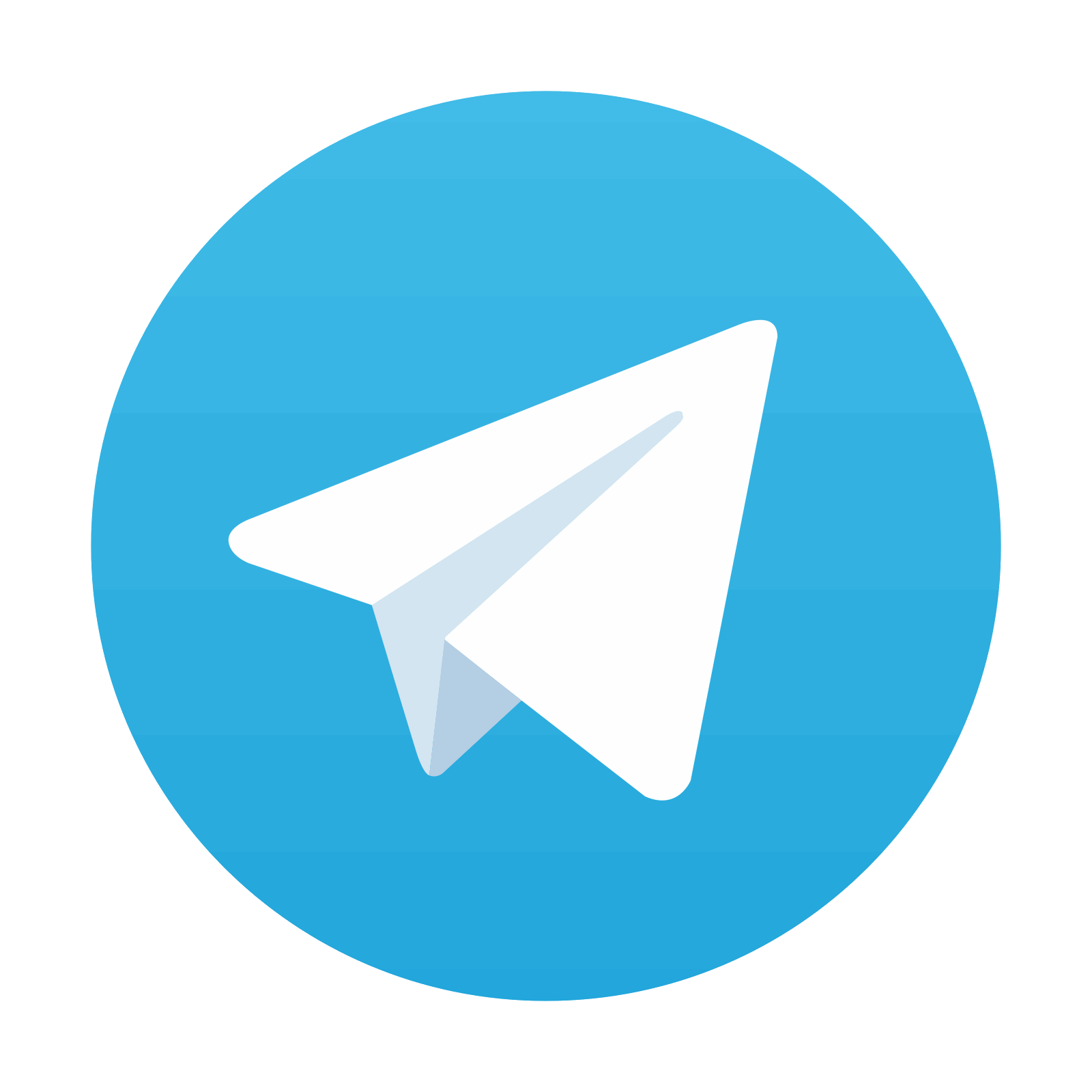
Stay updated, free articles. Join our Telegram channel

Full access? Get Clinical Tree
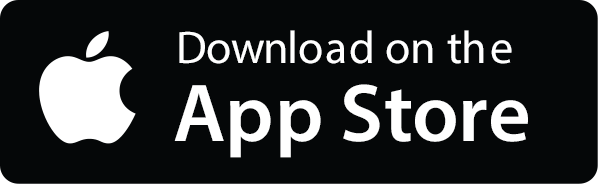
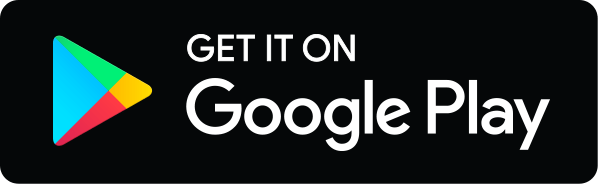