Mark A. Creager, Peter Libby
Peripheral Artery Diseases
Peripheral artery disease (PAD) generally refers to a disorder in which blood supply to the lower or upper extremities is obstructed.1 Most commonly caused by atherosclerosis, PAD may also result from thrombosis, embolism, vasculitis, fibromuscular dysplasia, or entrapment. The term peripheral vascular disease is less specific because it encompasses a group of diseases affecting blood vessels that include other atherosclerotic conditions such as renal artery disease and carotid artery disease, as well as vasculitides, vasospasm, venous thrombosis, venous insufficiency, and lymphatic disorders.
PAD correlates strongly with risk for major cardiovascular events because it associates frequently with coronary and cerebral atherosclerosis.2 Moreover, symptoms of PAD, including intermittent claudication, jeopardize quality of life and independence for many patients. PAD is commonly underdiagnosed and undertreated; thus practitioners of cardiology have increasing interest in its diagnosis and management. This chapter provides a framework for the diagnosis and management of patients with PAD.
Epidemiology
The prevalence of PAD varies according to the population studied, the diagnostic method used, and whether symptoms are included to derive estimates. Most epidemiologic studies have used a noninvasive measurement, the ankle-brachial index (ABI), to diagnose PAD. The ABI is the ratio of ankle to brachial systolic blood pressure (described in greater detail later). The prevalence of PAD based on abnormal ABI values ranges from approximately 6% in persons 40 years and older to 15% to 20% in those 65 years and older.3–5 The prevalence of PAD is greater in men than in women in most studies.5–7 Taking into consideration the total number of women and men in the U.S. population, however, there are more women than men with PAD.7 Blacks have a higher prevalence of PAD than non-Hispanic whites do.4 In MESA (Multi-Ethnic Study of Atherosclerosis), blacks had odds for the development of PAD that was 1.47 times higher than in non-Hispanic whites, whereas in Hispanics and Chinese it was less than 0.5 times that of non-Hispanic whites.8 These aggregate data indicate that some 8 to 10 million individuals in the United States have PAD.
Questionnaires specifically designed to elicit symptoms of intermittent claudication can serve to assess the prevalence of symptomatic disease in these populations. Estimates vary by age and sex but generally indicate that only 10% to 30% of patients with PAD have claudication. Overall, the estimated prevalence of claudication ranges from 1.0% to 4.5% in a population older than 40 years.5,6 The prevalence and incidence of claudication increase with age and are greater in men than in women in most studies (Fig. 58-1).4,5,7 Less information is available on the prevalence and incidence of critical limb ischemia. Its estimated incidence is 500 to 1000 per million population per year, and it affects 1% to 2% of patients with PAD.5,6 The incidence of amputation ranges from 112 to 250 per million population per year.
Risk Factors for Peripheral Artery Disease
The well-known modifiable risk factors associated with coronary atherosclerosis also contribute to atherosclerosis of the peripheral circulation (see Chapter 42). Cigarette smoking, diabetes mellitus, dyslipidemia, and hypertension increase the risk for PAD (Table 58-1). Data from several observational studies indicate a twofold to fourfold increase in the prevalence of PAD in current smokers in comparison to never smokers.4 There is a dose-response relationship between lifetime exposure to cigarettes and the incidence of symptomatic PAD. In the Women’s Health Study, the hazard ratio for incident symptomatic PAD in smokers of more than 15 cigarettes per day was 17 (95% confidence interval [CI], 11 to 27); the risk decreased following smoking cessation.9 Patients with diabetes mellitus often have extensive and severe PAD and a greater propensity for arterial calcification.10,11 Involvement of the femoral and popliteal arteries resembles that in nondiabetic persons, but distal disease affecting the tibial and peroneal arteries occurs more frequently. The risk for development of PAD increases twofold to fourfold in patients with diabetes mellitus.4 Among patients with PAD, diabetic patients are more likely than nondiabetic patients to undergo an amputation, and diabetes increases the risk for critical limb ischemia.12 In NHANES (National Health and Nutrition Examination Survey), insulin resistance was associated with a greater prevalence of PAD,13 and patients with metabolic syndrome had an increased risk for the development of symptomatic PAD in the Women’s Health Study,14 but not in the Edinburgh Artery Study.15 Abnormalities in lipid metabolism also associate with an increased prevalence of PAD. Elevations in total or low-density lipoprotein (LDL) cholesterol increase the risk for development of PAD and claudication in most studies. Hypertriglyceridemia predicts risk for PAD when considered as an independent variable, but its effect diminishes when considered in the context of other lipid fractions.4 In addition, hypertension increases the risk for PAD by 1.3- to 2.2-fold.4 In the Women’s Health Study, incident symptomatic PAD related to the severity of hypertension.16 Chronic kidney disease also augments PAD.17 The risk for development of PAD and intermittent claudication increases progressively with the burden of contributing factors.
TABLE 58-1
Odds Ratio of Peripheral Artery Disease in Persons with Risk Factors
RISK FACTOR | ODDS RATIO (95% CI) |
Cigarette smoking | 4.46 (2.25-8.84) |
Diabetes mellitus | 2.71 (1.03-7.12) |
Hypertension | 1.75 (0.97-3.13) |
Hypercholesterolemia | 1.68 (1.09-2.57) |
Hyperhomocysteinemia | 1.92 (0.95-3.88) |
Chronic kidney disease | 2.00 (1.08-3.70) |
Insulin resistance | 2.06 (1.10-4.00) |
C-reactive protein | 2.20 (1.30-3.60) |
Data derived from reports of the National Health and Nutrition Examination (Selvin E, Erlinger TP: Prevalence of and risk factors for peripheral arterial disease in the United States: Results from the National Health and Nutrition Examination Survey, 1999-2000. Circulation 110:738, 2004; Pande RL, Perlstein TS, Beckman JA, Creager MA: Association of insulin resistance and inflammation with peripheral arterial disease: The National Health and Nutrition Examination Survey, 1999 to 2004. Circulation 118:33, 2008; O’Hare AM, Glidden DV, Fox CS, Hsu CY: High prevalence of peripheral arterial disease in persons with renal insufficiency: Results from the National Health and Nutrition Examination Survey 1999-2000. Circulation 109:320, 2004; and Guallar E, Silbergeld EK, Navas-Acien A, et al: Confounding of the relation between homocysteine and peripheral arterial disease by lead, cadmium, and renal function. Am J Epidemiol 163:700, 2006.)
The pathobiology of PAD involves inflammation, as does atherosclerosis in other beds.18 High levels of fibrinogen associate with risk not only for coronary events but also for the development of PAD. Current analyses, however, suggest that adjustment for inflammatory markers, such as C-reactive protein, eliminates the risk for PAD associated with fibrinogen. Thus the elevated fibrinogen levels in patients with PAD may reflect inflammation as much as or more than a procoagulant effect. Considerable evidence links leukocytes, cells that mediate the inflammatory response, with the development of PAD. Levels of the soluble forms of leukocyte adhesion molecules correlate with the development and extent of PAD and with the risk for complications.19,20 Levels of C-reactive protein and monocytes in peripheral blood independently associate with PAD, consistent with a role of innate immunity and chronic inflammation in its pathogenesis.21,22 Conversely, serum bilirubin, an endogenous antioxidant with anti-inflammatory properties, associates with reduced PAD prevalence.23 Inflammation provides the mechanistic link between many of the common risk factors for atherosclerosis and the pathophysiologic processes in the arterial wall that lead to PAD.
Clinical Features
Symptoms
The cardinal symptoms of PAD include intermittent claudication and pain at rest. The term claudication is derived from the Latin word claudicare, “to limp.” Intermittent claudication refers to a pain, ache, sense of fatigue, or other discomfort that occurs in the affected muscle group with exercise, particularly walking, and resolves with rest. The location of the symptom is often related to the site of the most proximal stenosis. Buttock, hip, or thigh claudication typically occurs in patients with obstruction of the aorta and iliac arteries. Calf claudication is caused by femoral or popliteal artery stenoses. The gastrocnemius muscle consumes more oxygen during walking than other muscle groups in the leg do and hence causes the most frequent symptoms reported by patients. Ankle or foot claudication occurs in patients with tibial and peroneal artery disease. Similarly, stenoses of the subclavian, axillary, or brachial arteries may cause shoulder, biceps, or forearm claudication, respectively. The symptoms should resolve several minutes after cessation of effort. Calf and thigh pain that occurs during rest, such as nocturnal cramps, should not be confused with claudication and is not a symptom of PAD. The history obtained from persons reporting claudication should note the walking distance, speed, and incline that precipitate claudication. Such baseline assessment is used to evaluate disability and provides an initial qualitative measure with which to determine stability, improvement, or deterioration during subsequent encounters with the patient. Symptoms other than claudication can also limit functional capacity. Patients with PAD walk more slowly and have less walking endurance than do patients without PAD.27
Several questionnaires can be used to assess the presence and severity of claudication. The Rose Questionnaire was developed initially to diagnose both angina and intermittent claudication in epidemiologic surveys. It questions whether pain develops in either calf with walking and whether the pain occurs at rest, while walking at an ordinary or hurried pace, or on walking uphill. Several modifications of this questionnaire have been developed, including the Edinburgh Claudication Questionnaire and the San Diego Claudication Questionnaire,28 both of which are more sensitive and specific than a physician’s diagnosis of intermittent claudication based on walking distance, walking speed, and nature of the symptoms. Another validated instrument, the Walking Impairment Questionnaire, asks a series of questions and derives a point score based on walking distance, walking speed, and nature of the symptoms.29
Symptoms resembling limb claudication occasionally result from nonatherosclerotic causes of arterial occlusive disease (Table 58-3), including arterial embolism; vasculitides such as thromboangiitis obliterans, Takayasu arteritis, and giant cell arteritis; aortic coarctation; fibromuscular dysplasia; irradiation; endofibrosis of the external iliac artery; and extravascular compression as a result of arterial entrapment or an adventitial cyst (see Chapter 84). Several nonvascular causes of exertional leg pain enter into the differential diagnosis of intermittent claudication (see Table 58-3). Lumbosacral radiculopathy resulting from degenerative joint disease, spinal stenosis, and herniated discs can cause pain in the buttock, hip, thigh, calf, or foot with walking, often after very short distances or even with standing. This symptom has been called neurogenic pseudoclaudication. Lumbosacral spine disease and PAD both preferentially affect the elderly and hence may coexist in the same individual. Arthritis of the hips and knees also provokes leg pain with walking. Typically, the pain is localized to the affected joint and can be elicited on physical examination by palpation and range-of-motion maneuvers. Exertional compartment syndrome most often occurs in athletes with large calf muscles; increased tissue pressure during exercise limits microvascular flow and results in calf pain or tightness. Symptoms improve after cessation of exercise. Rarely, skeletal muscle disorders such as myositis can cause exertional leg pain. Muscle tenderness, an abnormal finding on neuromuscular examination, elevated skeletal muscle enzyme levels, and normal findings on pulse examination should distinguish myositis from PAD. Glycogen storage disease type V, also known as McArdle syndrome, in which skeletal muscle phosphorylase is deficient, can cause symptoms mimicking the claudication of PAD. Patients with chronic venous insufficiency sometimes report leg discomfort with exertion, a condition designated venous claudication. Venous hypertension during exercise increases arterial resistance in the affected limb and limits blood flow. In the case of venous insufficiency, elevated extravascular pressure caused by interstitial edema further diminishes capillary perfusion. Peripheral edema, venous stasis pigmentation, and occasionally venous varicosities demonstrated on physical examination will identify this unusual cause of exertional leg pain.
TABLE 58-3
Differential Diagnosis of Exertional Leg Pain
Vascular Causes |
Nonvascular Causes |
Symptoms may occur at rest in patients with critical limb ischemia. Typically, patients complain of pain or paresthesias in the foot or toes of the affected extremity. This discomfort worsens on leg elevation and improves with leg dependency, as might be anticipated by the effect of gravity on perfusion pressure. The pain can be particularly severe at sites of skin fissuring, ulceration, or necrosis. Frequently, the skin is very sensitive, and even the weight of bedclothes or sheets elicits pain. Patients may sit on the edge of the bed and dangle their legs to alleviate the discomfort. In contrast, patients with ischemic or diabetic neuropathy can experience little or no pain despite the presence of severe ischemia.
Critical limb and digital ischemia can result from arterial occlusions other than those caused by atherosclerosis, including conditions such as thromboangiitis obliterans, vasculitides such as systemic lupus erythematosus or scleroderma, vasospasm, atheromatous embolism, and acute arterial occlusion secondary to thrombosis or embolism (see later). Acute gouty arthritis, trauma, and sensory neuropathy such as that caused by diabetes mellitus, lumbosacral radiculopathies, and complex regional pain syndrome (previously known as reflex sympathetic dystrophy) can cause foot pain. Leg ulcers also occur in patients with venous insufficiency or sensory neuropathy, particularly that related to diabetes. These ulcers appear to be distinct from those caused by arterial disease. The ulcer of venous insufficiency usually localizes near the medial malleolus and has an irregular border and a pink base with granulation tissue. Ulcers attributable to venous disease produce milder pain than do those caused by arterial disease. Neurotrophic ulcers occur at sites of pressure or trauma, usually on the sole of the foot. These ulcers are deep, frequently infected, and not generally painful because of the loss of sensation.
Physical Findings
A complete cardiovascular examination includes palpation of the peripheral pulses and auscultation of accessible arteries for bruits (see also Fig. 11-4). Pulse abnormalities and bruits increase the likelihood of PAD.30 Readily palpable pulses in healthy individuals include the brachial, radial, and ulnar arteries in the upper extremities and the femoral, popliteal, dorsalis pedis, and posterior tibial arteries in the lower extremities. The aorta can also be palpated in thin people. A decreased or absent pulse provides insight into the location of arterial stenoses. For example, a normal right femoral pulse but absent left femoral pulse suggests the presence of left iliofemoral arterial stenosis. A normal femoral artery pulse but absent popliteal artery pulse would indicate a stenosis in the superficial femoral artery or proximal popliteal artery. A palpable popliteal artery pulse with absent dorsalis pedis or posterior tibial artery pulses indicate disease of the anterior and posterior tibial arteries, respectively. Bruits are often a sign of accelerated blood flow velocity and flow disturbance at sites of stenosis. A stethoscope should be used to auscultate the supraclavicular and infraclavicular fossae for evidence of subclavian artery stenosis; the abdomen, flank, and pelvis for evidence of stenoses in the aorta and its branch vessels; and the inguinal region for evidence of femoral artery stenoses. Pallor can be elicited on the soles of the feet of some patients with PAD by performing a maneuver in which the feet are elevated above the level of the heart and the calf muscles are exercised by repeated dorsiflexion and plantar flexion of the ankle. The legs are then placed in the dependent position, and the time until the onset of hyperemia and venous distention is measured. Each of these variables depends on the rate of blood flow, which in turn reflects the severity of stenosis and adequacy of collateral vessels.
The legs of patients with chronic aortoiliac disease may show muscle atrophy. Additional signs of chronic low-grade ischemia include hair loss, thickened and brittle toenails, smooth and shiny skin, and atrophy of the subcutaneous fat of the digital pads. Patients with severe limb ischemia have cool skin and may also have petechiae, persistent cyanosis or pallor, dependent rubor, pedal edema resulting from prolonged dependency, skin fissures, ulceration, or gangrene. The ulcers caused by PAD typically have a pale base with irregular borders and usually involve the tips of the toes or the heel of the foot or develop at sites of pressure (Fig. 58-3). These ulcers vary in size and may be as small as 3 to 5 mm.
Categorization
Classification of patients with PAD depends on the severity of the symptoms and abnormalities detected on physical examination. Categorization of the clinical manifestations of PAD improves communication among professionals caring for these patients and provides a structure for defining guidelines for therapeutic interventions. Fontaine described one widely used scheme in which patients are classified into one of four stages progressing from asymptomatic to critical limb ischemia (Table 58-4). Several professional vascular societies have adopted a contemporary, more descriptive classification that includes asymptomatic patients, three grades of claudication, and three grades of critical limb ischemia ranging from rest pain alone to minor and major tissue loss (Table 58-5).31
TABLE 58-4
Fontaine Classification of Peripheral Artery Disease
STAGE | SYMPTOMS |
I | Asymptomatic |
II | Intermittent claudication |
IIa | Pain free, claudication walking >200 m |
IIb | Pain free, claudication walking <200 m |
III | Rest and nocturnal pain |
IV | Necrosis, gangrene |
Testing for Peripheral Artery Disease
Segmental Pressure Measurement
Measurement of systolic blood pressure along selected segments of each extremity is one of the simplest and most useful noninvasive measures for ascertaining the presence and severity of stenoses in the peripheral arteries. In the lower extremities, pneumatic cuffs are placed on the upper and lower portions of the thigh, on the calf, above the ankle, and often over the metatarsal area of the foot. Similarly, for the upper extremities, pneumatic cuffs are placed on the upper part of the arm over the biceps, on the forearm below the elbow, and at the wrist. Systolic blood pressure at each respective limb segment is measured by first inflating the pneumatic cuff to suprasystolic pressure and then determining the pressure at which blood flow occurs during deflation of the cuff. The onset of flow is assessed by placing a Doppler ultrasound flow probe over an artery distal to the cuff. In the lower extremities, it is most convenient to place the Doppler probe on the foot over the posterior tibial artery, as it courses inferior and posterior to the medial malleolus, or over the dorsalis pedis artery on the dorsum of the metatarsal arch. In the upper extremities, the Doppler probe can be placed over the brachial artery in the antecubital fossa or over the radial and ulnar arteries at the wrist.
Left ventricular contraction imparts kinetic energy to blood, which is maintained throughout the large and medium-sized vessels. Systolic blood pressure may be higher in the more distal vessels than in the aorta and proximal vessels because of amplification and reflection of blood pressure waves. A stenosis can cause loss of pressure energy as a result of increased frictional forces and disturbance of flow at the site of the stenosis. Approximately 90% of the cross-sectional area of the aorta must be narrowed before a pressure gradient develops. In smaller vessels, such as the iliac and femoral arteries, a 70% to 90% decrease in cross-sectional area will cause a resting pressure gradient sufficient to decrease systolic blood pressure distal to the stenosis. Taking into consideration the precision of this noninvasive method and the variability in blood pressure during even short periods, a blood pressure gradient in excess of 20 mm Hg between successive cuffs is generally used as evidence of arterial stenosis in the lower extremity, whereas a gradient of 10 mm Hg indicates a stenosis between sequential cuffs in the upper extremity. Systolic blood pressure in the toes and fingers is approximately 60% of the systolic blood pressure at the ankle and wrist, respectively, because pressure diminishes further in the smaller distal vessels.
Figure 58-4 provides examples of leg segmental pressure measurements in a patient with left calf claudication. In the right leg there are no pressure gradients between the upper and lower parts of the thigh and between the calf and ankle. In the left leg, pressure gradients between the upper and lower parts of the thigh, between the lower part of the thigh and calf, and between the calf and ankle indicate stenoses in the superficial femoral and popliteal arteries and in the tibioperoneal arteries.
Ankle-Brachial Index
Determination of the ABI is a simplified application of leg segmental blood pressure measurements that can readily be used at the bedside (see Fig. 58-4 and Fig. 11-4). This index is the ratio of systolic blood pressure measured at the ankle to systolic blood pressure measured at the brachial artery.32 A pneumatic cuff placed around the ankle is inflated to suprasystolic pressure and subsequently deflated while the onset of flow is detected with a Doppler ultrasound probe placed over the dorsalis pedis and posterior tibial arteries, thus denoting ankle systolic blood pressure. Brachial artery systolic pressure can be assessed in a routine manner with either a stethoscope to listen for the first Korotkoff sound or a Doppler probe to listen for the onset of flow during cuff deflation. According to the 2011 American College of Cardiology Foundation/American Heart Association (ACCF/AHA) update of the PAD guidelines, the normal ABI range is 1.00 to 1.40. An ABI value of 0.91 to 0.99 is borderline, and an ABI of 0.90 or less is abnormal.33 An ABI of 0.90 or lower has a specificity of 83% to 99% and a sensitivity of 69% to 73% in detecting stenoses greater than 50%.33 The sensitivity of an ABI less than 1.0 approaches 100%. The ABI is often used to gauge the severity of PAD. Patients with symptoms of leg claudication often have ABIs ranging from 0.5 to 0.8, and patients with critical limb ischemia usually have an ABI lower than 0.5. A low ABI is associated with shorter walking distance and lower speed. Less than 40% of patients whose ABI is lower than 0.40 can complete a 6-minute walk.34 In patients with skin ulcerations, ankle pressure below 55 mm Hg would predict poor ulcer healing. Leg blood pressure recordings are not reliable in patients with calcified vessels, as might occur in those with diabetes mellitus or renal insufficiency. Inflation of the pneumatic cuff cannot compress the calcified vessel; the Doppler probe consequently indicates continuous blood flow, even when the pressure exceeds 250 mm Hg. An ABI higher than 1.40 indicates a noncompressible artery.
Treadmill Exercise Testing
Treadmill exercise testing can be used to evaluate the clinical significance of peripheral artery stenoses and provide objective evidence of the patient’s walking capacity. The claudication onset time is defined as the time at which symptoms of claudication first develop, and the peak walking time occurs when the patient is no longer able to continue walking because of severe leg discomfort. This standardized and more objective measure of walking capacity supplements the patient’s history and provides a quantitative assessment of the patient’s disability, as well as a metric for monitoring therapeutic interventions.
Treadmill exercise protocols use a motorized treadmill that incorporates fixed or progressive speeds and angles of incline. A fixed workload test usually maintains a constant grade of 12% and a speed of 1.5 to 2.0 mph. A progressive, or graded, treadmill protocol typically maintains a constant speed of 2 mph while the grade gradually increases by 2% every 2 to 3 minutes. Repeated treadmill test results have better reproducibility with progressive than with constant grade protocols.
Treadmill testing can be used to determine whether arterial stenoses contribute to the patient’s symptoms of exertional leg pain. During exercise, blood flow through a stenosis increases as vascular resistance falls in the exercising muscle. According to the Poiseuille equation, described previously, the pressure gradient across the stenosis increases in direct proportion to flow. Thus ankle and brachial systolic blood pressure is measured during resting conditions before treadmill exercise, within 1 minute after exercise, and repeatedly until baseline values are reestablished. Normally, the increase in blood pressure that occurs during exercise should be the same in both the upper and lower extremities, with a constant ABI of 1.0 or greater being maintained. In the presence of peripheral artery stenoses, however, the ABI decreases because the increase in blood pressure observed in the arm is not matched by a comparable increase in ankle blood pressure. A 25% or greater decrease in the ABI after exercise in a patient whose walking capacity is limited by claudication is considered diagnostic and implicates PAD as a cause of the patient’s symptoms.
Many patients with PAD also have coronary atherosclerosis. The addition of cardiac monitoring to the exercise protocol may provide adjunctive information about the presence of myocardial ischemia. Claudication can limit attainment of a workload sufficient to increase myocardial oxygen demand and provoke myocardial ischemia. Nonetheless, electrocardiographic changes, particularly during low levels of treadmill exercise, may provide evidence of severe coronary artery disease (CAD).
Pulse Volume Recording
The pulse volume recording graphically illustrates the volumetric change in a segment of the limb that occurs with each pulse. Plethysmographic instruments, typically using strain gauges or pneumatic cuffs, can transduce volumetric changes in the limb, which can be displayed on a graphic recorder. These transducers are strategically placed along the limb to record the pulse volume in its different segments, such as the thigh, calf, ankle, metatarsal region, and toes or the upper part of the arm, forearm, and fingers. The normal pulse volume contour depends on both local arterial pressure and vascular wall distensibility and resembles a blood pressure waveform. It consists of a sharp systolic upstroke rising rapidly to a peak, a dicrotic notch, and a concave downslope that drops off gradually toward the baseline. The contour of the pulse wave changes distal to a stenosis, with loss of the dicrotic notch, a slower rate of rise, a more rounded peak, and a slower descent. The amplitude becomes lower with increasing severity of disease, and the pulse wave may not be recordable at all in a critically ischemic limb. Segmental analysis of the pulse wave may indicate the location of an arterial stenosis, which probably resides in the artery between a normal and an abnormal pulse volume recording. The pulse volume wave also provides information about the integrity of blood flow when blood pressure measurements cannot be obtained accurately because of noncompressible vessels.
Doppler Ultrasonography
Continuous-wave and pulsed-wave Doppler systems transmit and receive high-frequency ultrasound signals. The Doppler frequency shift caused by moving red blood cells varies directly with the velocity of blood flow. Typically, the perceived frequency shift is between 1 and 20 kHz and is within the audible range of the human ear. Therefore placement of a Doppler probe along an artery enables the examiner to hear whether blood flow is present and the vessel is patent. Processing and graphic recording of the Doppler signal permit a more detailed analysis of the frequency components.
Doppler instruments can be used without or with gray-scale imaging to evaluate an artery for the presence of stenoses. The Doppler probe is positioned at approximately a 60-degree angle over the common femoral, superficial femoral, popliteal, dorsalis pedis, and posterior tibial arteries. The normal Doppler waveform has three components: a rapid forward-flow component during systole, a transient flow reversal during early diastole, and a slow anterograde component during late diastole. The Doppler waveform becomes altered if the probe is placed distal to an arterial stenosis and is characterized by deceleration of systolic flow, loss of the early diastolic reversal, and diminished peak frequencies. Arteries in a limb with critical ischemia may not show any Doppler frequency shift. As with pulse volume recordings, change from a normal to an abnormal Doppler waveform as the artery is interrogated more distally suggests the location of a stenosis.
Duplex Ultrasound Imaging
Duplex ultrasound imaging provides a direct, noninvasive means of assessing both the anatomic characteristics of peripheral arteries and the functional significance of arterial stenoses. The methodology incorporates gray-scale B-mode ultrasound imaging, pulsed Doppler velocity measurements, and color coding of the Doppler shift information (Fig. 58-5). Real-time ultrasonographic scanners emit and receive high-frequency sound waves, typically ranging from 2 to 10 MHz, to construct an image. The acoustic properties of the vascular wall differ from those of the surrounding tissue, thus enabling them to easily be imaged. Atherosclerotic plaque may be present and visible on gray-scale images. Pulsed-wave Doppler systems emit ultrasound beams at precise times and can therefore sample the reflected ultrasound waves at specific depths to enable the examiner to determine blood cell velocity within the lumen of the artery. By positioning the pulsed Doppler beam at a known angle, the examiner can calculate blood flow velocity according to the equation

where Df is the frequency shift, V is the velocity, F is the frequency of the transmitted sound, θ is the angle between the transmitted sound and the velocity vector, and C is the velocity of sound and tissue. For optimal measurements, the angle of the pulsed Doppler beam should be less than 60 degrees. With color Doppler, the frequency shift information within the entire field sampled by the ultrasound beam can be superimposed on the gray-scale image. This approach provides a composite real-time display of flow velocity within the vessel.
Color-assisted duplex ultrasound imaging is an effective means of localizing peripheral arterial stenoses (Fig. 58-6). Normal arteries have laminar flow, with the highest velocity occurring at the center of the artery. The corresponding color image is usually homogeneous with relatively constant hue and intensity. In the presence of an arterial stenosis, blood flow velocity increases through the narrowed lumen. As the velocity increases, there is progressive desaturation of the color display, and flow disturbance distal to the stenosis causes changes in hue and color. Pulsed Doppler velocity measurements can be made along the length of the artery and particularly at areas of flow abnormalities suggested by the color images. A twofold or greater increase in peak systolic velocity at the site of an atherosclerotic plaque indicates a 50% or greater stenosis (see Fig. 58-6). A threefold increase in velocity suggests a 75% or greater stenosis. An occluded artery generates no Doppler signal. With contrast-enhanced angiography as a reference standard, duplex ultrasound imaging for identification of sites of arterial stenosis has approximately 89% to 99% specificity and 80% to 98% sensitivity.35,36
Magnetic Resonance Angiography
Magnetic resonance angiography (MRA) can be used to noninvasively visualize the aorta and peripheral arteries (see Chapters 17 and 60). Resolution of the vascular anatomy with gadolinium-enhanced MRA approaches that of conventional contrast-enhanced digital subtraction angiography (Fig. 58-7). A meta-analysis of 32 studies comparing MRA with intra-arterial digital subtraction angiography found a pooled sensitivity for MRA of 94.7% (95% CI, 92.1% to 96.4%) and a pooled specificity of 95.6% (95% CI, 94.0% to 96.8%) for detecting segmental stenotic and occlusive lesions.37
MRA currently has its greatest usefulness in the evaluation of symptomatic patients to assist in decision making before endovascular and surgical intervention or in patients at risk for renal, allergic, or other complications during conventional angiography.
Computed Tomographic Angiography
New computed tomography scanners use multidetector technology to acquire cross-sectional images (see Chapter 18). This advance permits imaging of peripheral arteries with excellent spatial resolution during a relatively short time and with a reduced amount of radiocontrast material (Fig. 58-8). Image reconstructions in three dimensions permit rotation to optimize visualization of arterial stenoses. When compared with conventional contrast-enhanced angiography, the sensitivity and specificity for stenoses greater than 50% or occlusion reported for computed tomographic angiography (CTA) using multidetector technology are 95% (95% CI, 92% to 97%) and 96% (95% CI, 93% to 97%), respectively.38 CTA offers advantages over MRA in that it can be used in patients with stents, metal clips, and pacemakers, although it has the disadvantage of requiring radiocontrast material and ionizing radiation.
Contrast-Enhanced Angiography
Conventional angiography can aid in evaluation of the arterial anatomy before a revascularization procedure. It still has occasional usefulness when the diagnosis is in doubt. Most contemporary angiography laboratories use digital subtraction techniques after intra-arterial administration of contrast material to enhance resolution. Injection of the radiocontrast material into the aorta permits visualization of the aorta and iliac arteries, and injection of contrast material into the iliofemoral segment of the involved leg permits optimal visualization of the femoral, popliteal, tibial, and peroneal arteries (Fig. 58-9). In patients with aortic occlusion, catheterization of the femoral arteries is not feasible. The aorta can be approached by brachial or axillary artery cannulation or, if necessary, directly via a translumbar approach.
Prognosis
Patients with PAD have increased risk for adverse cardiovascular events, as well as for limb loss and impaired quality of life.2,4,6,27 Such patients frequently have concomitant CAD and cerebrovascular disease.5,6 The relative prevalence of each of these manifestations of atherosclerosis depends in part on the criteria used to establish the diagnosis. Patients with abnormal ABIs are twofold to fourfold more likely than those with normal ABIs to have a history of myocardial infarction (MI), angina, congestive heart failure, or cerebrovascular ischemia.5,6 Angiographically significant CAD occurs in approximately 60% to 80% of patients with PAD,6 and 15% to 25% of patients with PAD have significant carotid artery stenoses as detected by duplex ultrasonography. Two international registries have detected a high prevalence of concomitant CAD and cerebrovascular disease in patients with PAD. In the REACH (Reduction of Atherothrombosis for Continued Health) registry, 62% of the patients had either or both coronary and cerebrovascular disease.39 Approximately 25% of the patients with PAD had a history of MI, 30% had angina, 16% had a previous stroke, and 15% had a previous transient ischemic attack. In the AGATHA (A Global Atherothrombosis Assessment) registry, approximately 50% of the patients with PAD had established CAD and 50% had previous stroke, transient ischemic attack, or carotid artery revascularization.40 The specificity of an abnormal ABI in predicting future cardiovascular events is approximately 90%.32,41 The risk for death from cardiovascular causes increases 2.5- to 6-fold in patients with PAD, and their annual mortality rate is 4.3% to 4.9%.5,6,42,43 Those with the most severe PAD have the greatest risk for death, and mortality correlates with decreasing ABI (Fig. 58-10).32,44,45 Approximately 25% of patients with critical limb ischemia die within 1 year, and the 1-year mortality rate in patients who have undergone amputation for PAD may be as high as 45%.5
Worsening symptoms develop in approximately 25% of patients with claudication. Clinical progression occurs in 7% to 9% of patients with claudication in the first year after diagnosis and in approximately 2% to 3% each year thereafter.5,6 Moreover, loss of mobility occurs more commonly in patients with PAD than in those without PAD, even in patients without classic symptoms of claudication.46
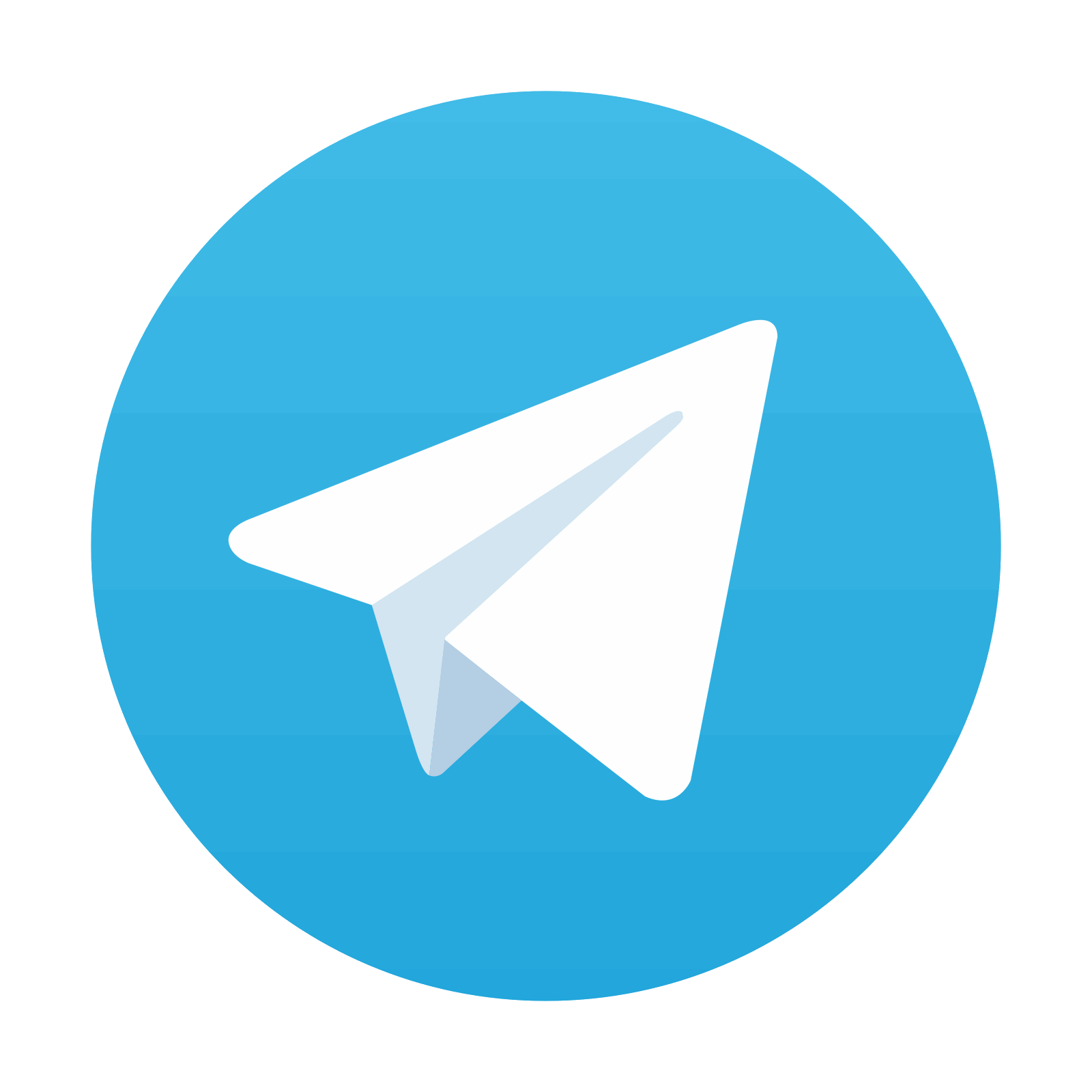
Stay updated, free articles. Join our Telegram channel

Full access? Get Clinical Tree
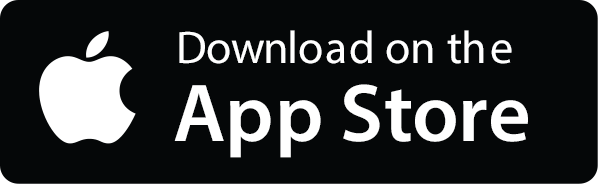
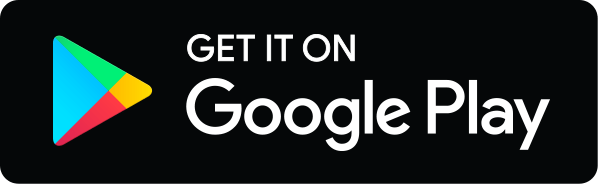