Introduction to Pericardial Diseases
- Itzhak Kronzon, MD
Hippocrates (c. 460-370 BC) described the normal pericardium as follows: “The pericardium is a smooth mantle surrounding the heart and containing a small amount of fluid resembling urine.” That description is fairly close to our understanding of the normal pericardium.
The normal pericardial sac consists of an outer layer, the fibrous pericardium, which is a few millimeters thick, and an inner layer, the serous pericardium, which lines the pericardial cavity. The normal serous pericardium is thin, usually just few layers of cells. The serous pericardium can be divided into the visceral pericardium (epicardium), which lines the heart, and the parietal pericardium, lining the fibrous pericardium. Normally, the pericardial cavity is mostly physiologic and contains up to 50 mL of pericardial fluid in the adult.
The pericardium has a role in maintaining the heart in place within the thorax, avoiding shift to the left. It also lubricates the heart to avoid friction with surrounding organs. It may provide protection from the spreading of extracardiac infection to the heart. It prevents acute dilatation of chambers and may also prevent hypertrophy under conditions of strenuous exercise. The pericardium is also responsible for ventricular interdependence, which normally exists to some degree and becomes exaggerated in conditions such as cardiac tamponade and constrictive pericarditis. The intact pericardium is also responsible for the drop in intrapericardial pressure during ventricular systole, which leads to better atrial filling.
However, the pericardium is not essential to health, functional capacity, or longevity. Patients with congenital or acquired absence of the pericardium can have normal hemodynamics and are usually asymptomatic.
The role of the pericardium in clinical disorders was amazingly well recognized and defined by Richard Lower (1631-1691), whose concepts of the pathophysiology of cardiac tamponade are almost identical to the way we describe them today: “So it happens when the covering of the heart is filled with effusion, and the walls are compressed with water on every side, so they cannot dilate to receive the blood.” ,
Lower’s description of constrictive pericarditis was also quite astute: “When such (pericardial fluid) is lacking (the pericardium) adheres closely to the heart… it is inevitable that the motion (of the pericardium) is combined and united with it (the heart)… this must be regarded as a great impediment and inconvenience.”
As with many other structures, patients and physicians were aware of the pericardium only when it became diseased. The range of pericardial symptoms is wide, from pleuritic chest pain due to stretching of the inflamed pericardium by cardiac chamber dilation during inspiration, to limitation of diastolic filling that results in elevation of diastolic intracardiac pressure, heart failure, and even death in situations such as cardiac tamponade and constrictive pericarditis. Pericardial abnormalities may be observed and diagnosed by professionals in many medical fields and subspecialties. This includes allergists, immunologists, endocrinologists, oncologists, nephrologists, infectologists, chest and heart surgeons, trauma experts, emergency room staff, radiologists, and cardiologists.
Early diagnosis may be crucial and is sometimes lifesaving; however, it may also be challenging and difficult. The history and physical examination are important. Both may frequently suggest the diagnosis but almost always require confirmation by further testing and imaging. Occasionally, the differential diagnosis demands additional workup.
In the past, accurate diagnosis of many pericardial disorders required invasive procedures. The presence of pericardial effusion was established by intravenous injection of carbon dioxide, which demonstrated the gap between the cardiac silhouette and the radiolucent right heart cavity, or by contrast angiography. The diagnosis of cardiac tamponade or constrictive pericarditis could be established only by cardiac catheterization.
In a groundbreaking publication, Feigenbaum and colleagues , reported the ability to use ultrasound to demonstrate the echo-free space between the immobile pericardium and the moving left ventricular posterior wall, and to diagnose pericardial effusion ( Fig. 139.1 ). The term “echocardiography” was later coined by Feigenbaum. This seminal work led to the clinical use of echocardiography in the United States, and later elsewhere.

Over the next five decades, echocardiography made great progress, and it now includes two-dimensional and three-dimensional imaging, transesophageal echocardiography, spectral and color Doppler, tissue Doppler, and speckle tracking. Each of these ultrasound modalities improves the ability to accurately and noninvasively diagnose the entire spectrum of pericardial disorders. Although for most conditions echocardiography alone is sufficient for diagnosis and initiation of treatment, other modern imaging modalities are sometimes needed to further refine the diagnosis and better initiate and tailor the treatment. These imaging modalities include cardiac computed tomography and cardiac magnetic resonance. Each of these can be useful in the evaluation of the structures, hemodynamics, and functional abnormalities of pericardial disease.
Table 139.1 describes and compares the strength and weakness of each of those modalities. Table 139.2 summarizes protocols and findings for the multimodality imaging modalities in the evaluation of pericardial diseases.
Echocardiography | Cardiac CT | CMR | |
---|---|---|---|
Main indications/ advantages |
|
|
|
Main limitations/ disadvantages |
|
|
|
Echocardiography | Prospective or Retrospective, ECG-Gated Multidetector CT | CMR |
---|---|---|
2-D imensional E chocardiography | A xial I maging | B right B lood S ingle -S hot SSFP and B lack B lood A xial S tacks (H alf F ourier SSTSE, ECG-G ated ) |
|
|
|
D oppler —S imultaneous R espirometry * | M ultiplanar R econstruction | B lack B lood I mages |
|
| (T1+T2-weighted fast spin echo) Optional: T2-weighted STIR (edema weighted) fast spin echo
|
M-M ode | V olume R endered I maging | T agged C ine I mages |
|
| (T1-weighted gradient echo)
|
C ine I maging (R etrospective G ated S tudy O nly ) | B right B lood C ine I mages (SSFP) | |
|
| |
L ate G adolinium E nhancement I mages | ||
(P hase -S ensitive I nversion R ecovery S equence )
| ||
R eal -T ime G radient E cho C ine I mage | ||
|
* Echo Doppler measurements should be repeated in the sitting position (reducing preload) in case of nondiagnostic findings and suspicion for constriction.
Invasive hemodynamic studies may still be needed to further assess clinical issues not resolved by the noninvasive armamentarium. The chapters in this section concentrate mainly on the echocardiographic findings of common and not-so-common pericardial disorders.
Normal Pericardial Anatomy
- Steven Giovannone, MD
- Robert Donnino, MD
- Muhamed Saric, MD, PhD
- Robert Donnino, MD
The pericardium is a membranous sac that envelops almost the entire heart (with the exception of the region of the left atrium around the pulmonary venous ostia) as well as the origins of the great cardiac vessels (the ascending aorta, the main pulmonary artery, and the venae cavae). The term “pericardium” is a Latinized version of the Greek word πɛρικάρδιον, which literally means “that which is around the heart.” As an anatomic term, the word has been used at least since the time of the Greco-Roman physician Galen; for instance, in around AD 160 he used it in describing stab wounds of gladiators resulting in pericardial effusions. In English, the word “pericardium” first appears in print around 1425 in a Middle English translation of Chirurgia Magna , a surgical treatise written in Latin by the French physician Guy de Chauliac (c. 1300-1368).
Phylogeny and embryology
The pericardium envelops the heart of all vertebrates including fishes, amphibians, reptiles, birds, and mammals. As such a phylogenetically ancient structure, it forms very early during embryologic development in humans (starting at around 5 weeks’ gestation) by the division of the coelom—the original visceral cavity—into pericardial, pleural, and peritoneal spaces. Through incompletely understood mechanisms, embryologic mishaps may result in congenitally absent pericardium or pericardial cysts.
Basic anatomy
The normal pericardium ( Fig. 140.1 ) consists of a double-layered sac: an outer fibrous envelope (fibrous pericardium) and an inner serous sac (serous pericardium). The serous pericardium can be divided into an outer (parietal) and an inner (visceral) layer. The parietal layer normally fuses with the fibrous pericardium to create an inseparable outer layer of the pericardium. The fibrous pericardium is contiguous with the adventitia of the great arteries.

The visceral layer of the serious pericardium is synonymous with the epicardium. Between these two layers there is a virtual space that contains a very small amount of clear serous fluid, as discussed later.
The pericardium spans the space between the third and the seventh rib. Strong superior and inferior sternopericardial ligaments anchor the pericardial sac to the posterior aspect of the sternum. In addition, loose fibrous tissue binds the pericardium to the diaphragm and surrounding thoracic structures, including pleurae. The right and left phrenic nerves travel in this loose tissue between the fibrous pericardium and the pleurae. The arterial supply to the pericardium is provided by the branches of the internal mammary arteries (especially the pericardiophrenic artery) and the descending thoracic aorta. The pericardiophrenic vein, ultimately draining into the brachiocephalic vein, provides the principal venous drainage of the pericardium. The nerves of the pericardium are derived from the sympathetic trunks as well as the vagus and phrenic nerves.
Pericardial thickness
Normal pericardial wall thickness is approximately 1 to 2 mm. It is important to emphasize that transthoracic echocardiography (TTE) does not delineate the pericardial wall boundaries well enough, and therefore TTE is not recommended for measurements of pericardial thickness by either the American Society of Echocardiography or the European Society of Cardiology guidelines on proper use of echocardiography in pericardial disorders. In contrast, pericardial thickness can be obtained by transesophageal echocardiography (TEE). TEE measurements approach the gold standard of computed tomography (CT) and cardiac magnetic resonance (CMR) imaging ( Fig. 140.2 ). Increased pericardial thickness due to fibrosis and calcification is the hallmark of constrictive pericarditis.

Pericardial fluid
Under physiologic conditions, there is only a very small amount of clear straw-colored pericardial fluid (typically < 50 mL) representing an ultrafiltrate of plasma. On echocardiography, the separation between parietal and visceral layers of the serous pericardium either is imperceptible or is seen only during ventricular systole as a slitlike echolucent area between the two pericardial layers. This small amount of fluid has multiple physiologic roles: It diminishes friction between the two pericardial layers; by being an incompressible fluid, it protects the heart from minor injuries; and it provides a source of vasoactive substances that may regulate the function of the heart and the coronary arteries.
Intrapericardial pressure
The intrapericardial pressure (P) is a product of the intrapericardial fluid volume (V) and the pericardial stiffness (ΔP/ΔV):
P = V ∗ Δ P Δ V
Pericardial stiffness, an inverse of pericardial compliance, is the slope of the intrapericardial pressure-volume curve. Because a normal pericardium is not an impediment for transmission of intrathoracic pressure changes into the pericardial space during physiologic respiration and because the physiologic amount of pericardial fluid is small, a normal intrapericardial pressure is close to 0 mm Hg or even negative (subatmospheric).
Under pathologic conditions, the intrapericardial pressure may rise either because of an increase in the amount of pericardial fluid (as with pericardial effusion) or because of pronounced pericardial stiffness (as in rapidly accumulating pericardial effusion or with effusive-constrictive pericarditis). The pericardial pressure-volume relationship is nonlinear; initially the slope is flat but subsequently becomes very steep. This nonlinear relationship explains why increases in the size of pericardial effusion initially may only modestly elevate intrapericardial pressures when the slope is flat. However, once the steep portion of the curve is reached, even a small additional increase in the size of pericardial effusion leads to marked increases in intrapericardial pressure ( Fig. 140.3 , A ).

When the intrapericardial pressure exceeds the pressure in any of the cardiac chambers during at least part of the cardiac cycle, tamponade physiology develops. Conversely, even removal of a relatively small amount of pericardial effusion may rapidly relieve signs and symptoms of tamponade. With slowly accumulating pericardial effusions, pericardial stiffness gradually falls, and thus the intrapericardial pressure remains near normal for longer periods of time when compared with acute pericardial effusions. Mathematically, this corresponds to a shift of the pressure-volume relationship to the right (see Fig. 140.3 , B ).
Intrapericardial versus extrapericardial heart structures
To fully understand pericardial physiology and pathology, it is important to recognize which heart structures lie within and which lie outside the pericardial sac. As noted earlier, the proximal portions of the great arteries (the ascending aorta and the main pulmonary artery) are located within the pericardial sac, whereas the superior portion of the left atrium and the ostia of the pulmonary veins are outside of the pericardial sac. Therefore dissections or other injuries of proximal portions of the great arteries may result in the development of a pericardial effusion.
In contrast, the extrapericardial location of the pulmonary veins contributes to exaggerated respiratory variations in tamponade and constrictive pericarditis. Briefly, under physiologic conditions, during inspiration the intrathoracic pressure drops, which leads to a drop in both pulmonary vein and intracardiac pressures. The opposite occurs during expiration. Because intrathoracic pressures changes affect the pulmonary veins and the left heart almost equally, the pressure gradient between the pulmonary vein and the left atrium does not change substantially. Therefore, under physiologic conditions there is only a minor decrease of left heart filling during inspiration.
Significant pericardial effusions and constrictive pericarditis insulate intracardiac chambers from changes in intrathoracic pressures during respiration. Thus, in such pathologic conditions, the normal inspiratory drop in intrathoracic pressures will lead to a drop in the pulmonary vein pressure without a concomitant drop in the left atrial pressure. The resulting decrease in the pressure gradient between the pulmonary vein and the left atrium leads to a marked drop in the filling of the left heart during inspiration. The concept of these exaggerated respiratory variations in tamponade and constrictive pericarditis is further discussed in other chapters of this book.
Pericardial fat
A variable amount of fat may be present in and around the pericardial sac; collectively this adipose tissue is referred to as the pericardial fat pad. Intrapericardial fat accumulates preferentially along the coronary arteries and in the atrioventricular groove; this is referred to as epicardial fat. Additional fat tissue may also accumulate outside the pericardium in the nearby mediastinum, particularly anterior to the right heart; this is referred to as mediastinal fat. On imaging, the epicardial and mediastinal fat layers should not be mistaken for a loculated pericardial effusion. Echocardiographically, pericardial fat is a noncircumferential accumulation of ultrasonographically heterogeneous material that moves in concert with the heart. In contrast, pericardial effusions are typically stationary, echolucent, and circumferential rather than restricted to the region around the right heart. Pericardial fat can also be well visualized by cardiac CT or MRI ( Fig. 140.4 and Video 140.4, A ).

Pericardial extensions
The main pericardial sac communicates with several extensions that are referred to as sinuses and recesses. There are two sinuses (oblique sinus and transverse sinus) and multiple recesses. The two sinuses do not communicate directly. Occasionally, pericardial effusion may only be present in one or more of these sinuses and recesses and absent from the main pericardial cavity ( Fig. 140.5 and Videos 140.5, A-C ). The oblique sinus is a blind pouch or cul-de-sac that overlies the posterior aspect of left atrium, normally between all four pulmonary veins, as well as a portion of the right atrium. The transverse sinus is bounded anteriorly by the origins of the great arteries, inferiorly by the roof of the left atrium, and posteriorly by the superior vena cava, the atria, and the left atrial appendage.
Extensions of the transverse sinus include the superior aortic recess (between the ascending aorta and the superior vena cava), the inferior aortic recess (between the ascending aorta and the right atrium), and the right and left pulmonic recesses (around the right and left pulmonary arteries). Pericardial effusions localized in the transverse sinus and its recesses should not be mistaken for other pathologies such as type A aortic dissection. The postcaval recess is an extension of the main pericardial cavity; it lies posterior and to the right of the superior vena cava. ,
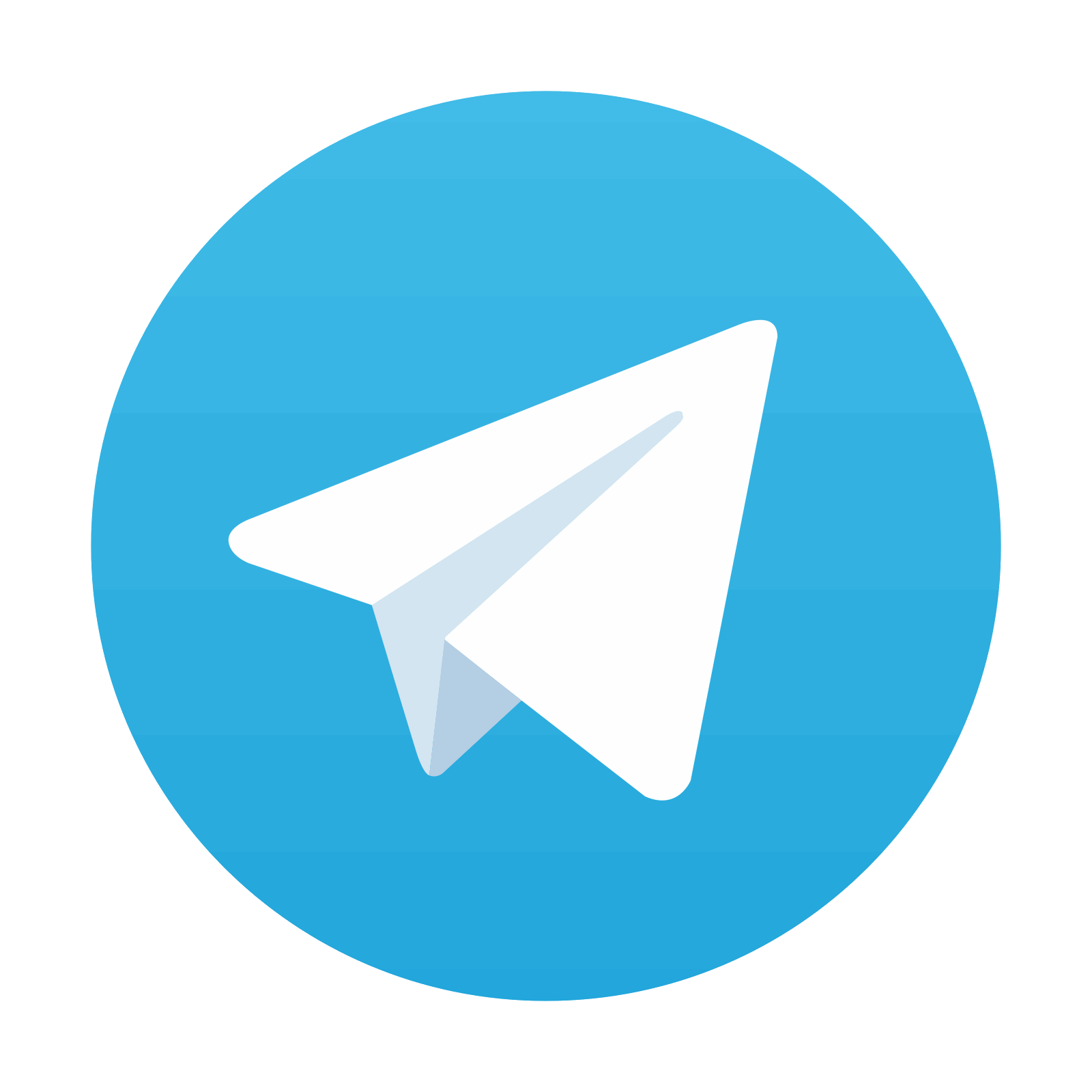
Stay updated, free articles. Join our Telegram channel

Full access? Get Clinical Tree
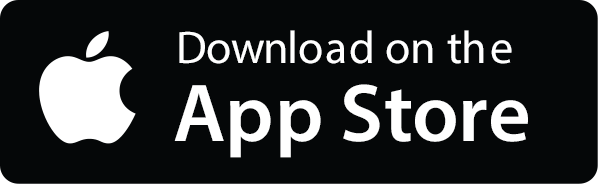
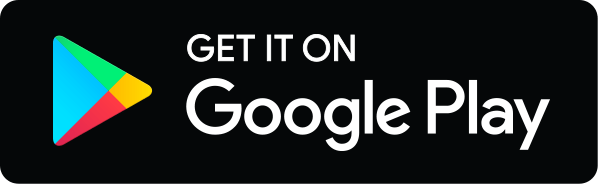