43 Pericardial Disease
Diagnosis and Hemodynamics
Pericardial pathology can present as an outpatient disease, in a setting requiring invasive diagnostic testing and surgery, or anywhere in between (see Chapter 42). This chapter focuses on the more significant presentations that result in hemodynamic compromise. In these cases, the common underlying physiologic abnormality, regardless of the specific pericardial pathology, is impaired diastolic filling of the heart. Significant constrictive pericarditis presents with evidence of right-sided heart failure, whereas pericardial tamponade presents with distinctive systemic hypotension. Diagnosis of these conditions is not always simple, since combinations of the disease processes can exist (effusive-constrictive pericarditis) and milder forms may require acute fluid loading to bring out characteristic hemodynamic findings (occult constrictive pericarditis). In addition, localized and transient forms of constrictive pericarditis have been described. The differential diagnosis between constrictive pericarditis and restrictive cardiomyopathy, addressed primarily in Chapter 20, can also be a challenge, because myocardial involvement may accompany the pericardial component and all three can indeed be present in the same patient. The hemodynamics can be further complicated if the patient has another disease altering the hemodynamics, such as pulmonary hypertension, an associated dilated cardiomyopathy, or significant valvular heart disease. Attention to details is mandatory in an attempt to sort out the presence of pericardial disease under these circumstances.
Etiology and Pathogenesis
Normal Physiology
Intracardiac pressures are a reflection of the contraction and relaxation of individual cardiac structures and the changes imparted to them by the pleural and pericardial pressures (Fig. 43-1). Changes in pleural or pericardial pressure affect the intracardiac diastolic pressure. With inspiration, the intrapleural pressures drop and the abdominal cavity pressure increases. Blood flow to the right side of the heart increases, whereas blood return to the left side of the heart decreases slightly. The fall in the intrapleural pressures also causes an increase in the transmural aortic root pressure, slightly increasing impedance to left ventricular (LV) ejection. The reverse occurs during expiration. In the normal setting, the respiratory changes are reflected in the intrapericardial and intracardiac pressures, with inspiration lowering the measured right atrial (RA) pressures and the systolic right ventricular (RV) pressure more than the left-sided heart pressures.
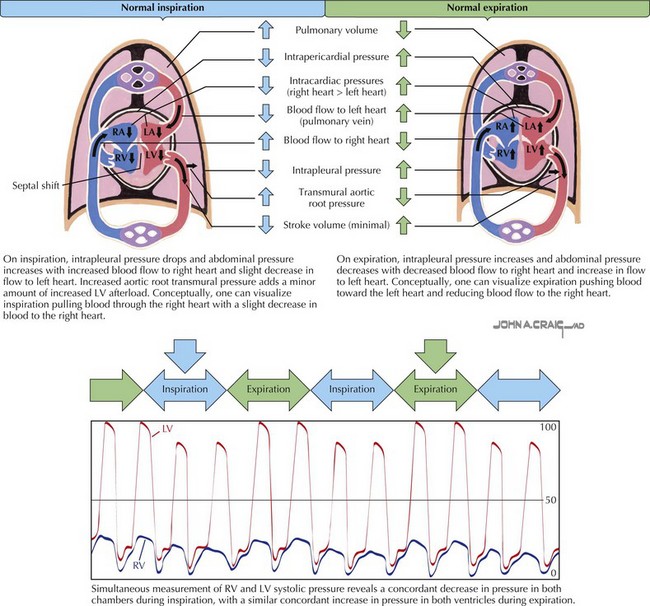
Figure 43-1 Normal cardiac blood flow during inspiration and expiration.
LA, left atrial; LV, left ventricular; RA, right atrial; RV, right ventricular.
The normal atrial and ventricular waveforms are shown in Figure 43-2. With atrial contraction, the atrial pressures rise (a wave). With the onset of ventricular contraction, the atrioventricular (AV) valves bulge toward the atria and a small c wave results (the c wave is evident on hemodynamic tracings but usually is not visible to the examiner observing the jugular venous pulsations). As ventricular contraction continues, the AV annulus is pulled into the ventricular cavity and the atria begin diastole, enlarging the atria and decreasing the atrial pressure (represented by the x descent). Passive filling of the atria during ventricular systole produces a slow rise in the atrial pressure (the v wave) until the AV valves reopen at the peak of the v wave; the pressure then falls rapidly as the ventricles begin active relaxation. Passive filling of the ventricles then follows until atrial contraction recurs, and the cycle repeats. Ventricular diastole can be conceptually divided into an initial active phase (a brief period when the ventricle fills about halfway) and a later passive filling phase. The nadir, or lowest, diastolic pressure during ventricular diastole occurs during the early active relaxation phase (suction effect).
Hemodynamics of Pericardial Constriction and Pericardial Tamponade
Constrictive pericarditis and pericardial tamponade alter the normal intracardiac pressures in several ways. Some of the hemodynamic abnormalities, such as ventricular interdependence, are seen in both processes, whereas others, such as the magnitude of the y descent, are unique to each (see Fig. 43-2).
Pericardial Constriction
Pressure Measurements
Constrictive pericarditis was recognized at autopsy in the 19th century and described as a “chronic fibrous callous thickening of the wall of the pericardial sac that is so contracted that the normal diastolic filling of the heart is prevented” (Fig. 43-3). The variable severity of the constrictive process results in a spectrum of hemodynamic change. Table 43-1 outlines the major features of the subacute (elastic) and the more chronic (rigid shell) forms of pericardial constriction.
Table 43-1 Comparison of Features Characteristic of Subacute (Elastic) and Chronic (Rigid Shell) Constrictive Pericarditis
Subacute (Elastic) | Chronic (Rigid Shell) |
---|---|
Paradoxical pulse usually present. Signs of ventricular interdependence prominent | Paradoxical pulse usually minimal or absent |
Ventricular interdependence less prominent | |
Prominent x and y descents (“M” or “W” waveform in the JVP) | Prominent y descent; x descent sometimes minimal |
Dip and plateau pattern less obvious | Conspicuous dip and plateau pattern |
Early diastolic nadir may not approach zero. | Early diastolic nadir approaches zero |
Calcification of pericardium rare | Calcification of pericardium more likely |
Pericardial effusion may be present. | Pericardial effusion absent |
Constriction primarily due to visceral pericardium | Constriction due to fusion of visceral and parietal pericardium and with epicardium of the heart |
ECG “P” waves usually normal | ECG “P” waves wide, notched, and low amplitude |
Atrial fibrillation or flutter uncommon | Atrial fibrillation or flutter common |
ECG, electrocardiogram; JVP, jugular venous pressure.
With permission from Hancock EW. Differential diagnosis of restrictive cardiomyopathy and constrictive pericarditis. Heart. 2001;86:343–349.
The difference between the subacute and the more chronic forms of constrictive pericarditis probably relates to whether only the visceral pericardium is fused to the epicardium of the heart (subacute) or both the visceral and the parietal pericardial layers are fused together (chronic). In both instances, the diastolic pressures in the atria are elevated due to the restriction of ventricular diastolic inflow. In constriction, the elevated atrial pressures and the normal early LV filling result in a rapid decrease in atrial pressure after the AV valves open and are responsible for the rapid y descent seen (see Fig. 43-2). However, the constraint imposed by the pericardium as the ventricle fills results in the sudden halting of this rapid early filling and an abrupt rise in pressure producing the “square root sign” or “dip and plateau” in the pressure tracings. The x descent is generally minimally affected; thus, the atrial y descent is greater than the x descent in constrictive pericardial disease. RV and pulmonary pressures are usually normal or only mildly elevated, with the result that the RV end-diastolic pressure (EDP) tends to be greater than one third the RV systolic pressure. At the end of ventricular diastole, both the right and left ventricles are confined by the pericardium, and there is equalization of the RV and LV EDPs.
Because the atrial and ventricular septa are unaffected by the pericardial process, changes in atrial and ventricular filling on the right side of the heart can affect left-sided filling (ventricular interdependence). Demonstration of ventricular interdependence is generally accepted as a fundamental requirement for diagnosing constrictive pericarditis. In constriction, as the negative intrapleural pressure draws blood through the RV with inspiration, the increase in RV filling into the confined right ventricle results in a rise in the RV systolic pressure while the normal fall in the LV systolic pressure occurs. This phenomenon is illustrated in Figure 43-2. An additional finding is that the width and area of the RV pressure tracing also increase with inspiration. Since LV filling falls with inspiration, the systolic areas of the RV and LV pressure-time tracing can be determined and the ratio of the RV systolic area to the LV systolic area determined during each respiratory phase. If constriction is present, the ratio of the RV systolic area to the LV systolic area is expected to be greater than 1.1. This ratio remains constant in restrictive disease and increases in constrictive pericarditis as the RV systolic pressure and area rise while the LV systolic pressure and area fall (discordance). In a review from the Mayo Clinic of this index, the average ratio in a group of 59 patients with constriction was 1.4 as compared with 0.92 for a group of 41 without constriction. The sensitivity of a systolic area index greater than 1.1 was 97% with a specificity of 100%, a positive predictive value of 100%, and a negative predictive value of 95%. In addition, reduced LV filling results in a lower pulmonary capillary wedge pressure to LV diastolic gradient in inspiration and a greater gradient in expiration (see Fig. 43-3).
