Fig. 9.1
(a) Current device for percutaneous pulmonary valve implantation (PPVI), Melody™, manufactured by Medtronic, Inc.; (b) mounted at the tip of the balloon catheter system; and (c) after implantation in a patient’s right ventricular outflow tract (RVOT)/pulmonary trunk
In this chapter, we review PPVI clinical experience with the current device—Melody® Transcatheter Pulmonary Valve (Medtronic, Inc., Minneapolis, MN, USA)—and its major limitations, primarily stent fractures and limited applicability. The shortcomings of the present valved stent have created a need for the next generation PPVI devices. The last part of this chapter describes one new device, its first-in-man implantation, and the optimization study performed on its design.
9.2 Current PPVI Device: Melody® Transcatheter Pulmonary Valve
The first device used for PPVI was developed from commercially available products—Contegra® Pulmonary Valved Conduit (Medtronic, Inc.) and CP Stent™ (NuMed, Inc., Hopkinton, NY, USA)—with proven utility in the clinical setting [8]. Contegra conduits are made from a segment of bovine jugular vein with a central native valve, which can have perfectly formed bicuspid or tricuspid arrangements, and are available up to 22 mm of diameter. The CP stent is a balloon-expandable device created by six platinum–10% iridium wires, formed into a zigzag-shaped pattern (eight crowns). The segments are joined together at the crowns to create the full length of the stent (34 mm), by welding of the platinum. Since the platinum welds were prone to fracture, this component of the PPVI was modified during the early clinical experience by introducing a gold braising process to reinforce the crowns. The stent can be crimped to a size of 6 mm before being re-expanded up to 24 mm. The valve itself can undergo multiple compressions and re-expansions inside the stent.
The bovine vein is attached to the stent by continuous sutures around the entire circumference at the inflow (clear sutures) and outflow (blue sutures) (Fig. 9.1a). Since the central portion of the valve created a “hammock” effect once implanted in patients, discrete sutures were added later at each strut intersection. The device undergoes sterilization using a proprietary sterilant containing glutaraldehyde and isopropyl alcohol, in which it is then packaged. Before the procedure in the catheterization laboratory, the valved stent assembly is prepared in three sequential saline baths to wash off the glutaraldehyde and hand crimped on a custom-made delivery system.
The delivery system (Ensemble®, Medtronic, Inc.) is comprised of a balloon-in-balloon (BiB) deployment design at its distal end onto which the valved stent is front loaded (Fig. 9.1b). The system is available with three outer balloon diameters (18 mm, 20 mm, and 22 mm); the inner balloon diameters equal ½ of the outer diameter (9 mm, 10 mm, and 11 mm, respectively). The tip of the system is blue to correspond with the outflow sutures of the device for correct orientation. A retractable sheath covers the valved stent during delivery and is pulled back just prior to deployment. Contrast can be delivered via the retracted sheath from a side port to confirm positioning of the device prior to deployment. Proximally, there are three ports for the guidewire (green), the inner balloon (indigo), and the outer balloon (orange). The balloons are inflated in succession, the inner balloon first which helps further in positioning the stent, followed by the outer balloon which fully deploys the device.
9.2.1 Clinical Experience
In September 2000, the first PPVI device was successfully implanted at Hôpital Necker Enfants Malades (Paris, France) in a 12-year-old boy who had developed dysfunction of his RVOT/pulmonary trunk conduit [6]. Over the last 10 years, PPVI has shown a marked learning curve [7] with safety and efficacy improvements that have led to the CE mark in 2006, Food and Drug Administration (FDA) Humanitarian Device Exemption (HDE) approval in 2010, and successful worldwide clinical use of this procedure (>1,500 implants).
PPVI is performed to prolong the lifespan of right ventricle to pulmonary artery conduits in both obstructive and regurgitant scenarios, and thereby postpone open-heart surgery for RVOT/pulmonary trunk dysfunction [7]. Criteria for bare stenting and surgical PVR have provided general guidance when determining indications for PPVI [9, 10]. Patients are considered for this procedure if they had previously undergone surgery of the RVOT during repair of congenital heart disease and have symptoms or RVOT dysfunction of a sufficient degree to warrant further surgical intervention, based on conventional practices for surgical indications. All potential candidates are discussed in a multidisciplinary meeting of cardiologists, cardiothoracic surgeons, and intensivists and are studied before and after intervention. The patients have a detailed clinical examination, electrocardiogram (ECG), chest radiograph, and echocardiography including color Doppler, continuous-, and pulsed-wave Doppler. Magnetic resonance (MR) imaging is performed to assess right ventricular volume and function, outflow obstruction, pulmonary regurgitation, outflow tract morphology, and exercise capacity.
Since the first implant, PPVI has been shown to be feasible at relatively low risks. Serious adverse events associated with Melody™ have been relatively uncommon. Worldwide, two procedure-related deaths have been reported thus far because of coronary dissection and coronary compression [11]. Though rare, other procedural complications have included conduit rupture, branch pulmonary artery injuries, and entrapment of the delivery system in the tendinous cords of the tricuspid valve causing significant tricuspid regurgitation [7].
The follow-up of patients has revealed reduced RVOT obstruction, a competent pulmonary valve, improved functional status and peak exercise parameters, and in some patient subsets, improved biventricular function and efficiency [7, 11]. Stent fractures, which occur in approximately 20% of the patients [12], and recurrent RVOT obstruction are the only device-related adverse events reported during follow-up [7, 12], often requiring re-intervention. A stent-in-stent approach, which consists of the placement of a second Melody™ valve within the first [13], has recently been adopted to care for PPVI failure.
Despite its clinical success, Melody™ is only suitable for those patients with an RV to PA conduit that is small, cylindrical, and rigid (<15% of patients requiring treatment of pulmonary valve dysfunction) [14], meaning that 85% of the patients requiring treatment for pulmonary valve dysfunction still undergo open-heart surgery. These two device-related issues (stent failure and limited applicability), along with potential innovative solutions, will be discussed in the next paragraphs.
9.2.2 Stent Fracture
9.2.2.1 Predicting Stent Fractures
During the development phases of the current PPVI device, simple bench testing and animal experiments were performed as part of routine preclinical testing. These in vitro and in vivo experiments predicted valve degeneration with no stent fracturing. However, in our clinical practice, the opposite occurred—good valve function and ∼20% stent fractures [12]. These discrepancies are most likely due to the fact that the in vivo loading conditions were incorrectly understood and therefore experimental setups did not accurately mimic the actual in vivo environment. For example, in the bench fatigue assessment, PPVI stents were placed in distensible cylindrical tubes, where they were tested with cyclic pressures over millions of cycles. In such a setup, no stent fractures were seen to occur in the PPVI stent. Indeed, finite element (FE) analysis performed on the device with the same conditions (cylindrical expansion) confirmed the in vitro findings [15]. Therefore, fracture should not occur. However, these loading conditions are simplified and not representative of the real situation, where the final shape of the stent in situ is far away from being uniformly cylindrical.
In order to better understand and predict stent mechanical performance and durability, the implantation site morphology must be taken into account when in vitro testing is performed. To demonstrate this concept, a patient-specific approach was adopted in a computational simulation. The patient (25-year-old woman with significant pulmonary homograft stenosis and mild pulmonary regurgitation) who had undergone successful PPVI subsequently experienced stent fractures [16].
The patient’s pre-procedural MR images were used to create an FE model of the implantation site (Fig. 9.2a). The MR data were elaborated using imaging post-processing software (Mimics, Materialise, Leuven, Belgium); a rigid shell of the implantation site was defined [17]; and an FE mesh was generated (ABAQUS/Standard, Simulia, Providence, RI, USA). An FE model of the PPVI stent was also created [15]. A simulation of stent deployment inside the patient-specific RVOT model was then carried out by pressure loading, using a contact interaction algorithm (Fig. 9.2b). When the device was fully expanded, the pressure was reset to zero to enable elastic recoil, and a new pressure (0.1 MPa) was applied to the external surface of the stent to mimic different cardiac cycle conditions: early systole and diastole. Biplane fluoroscopy images from the actual PPVI procedure were used to verify the computer-simulated configurations of the stent during early systole and diastole [16].
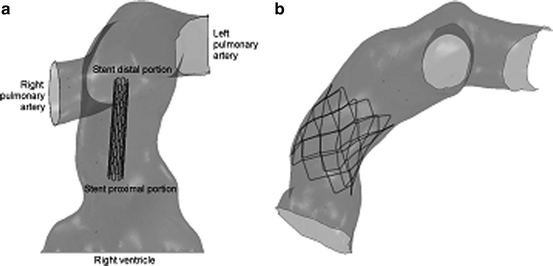
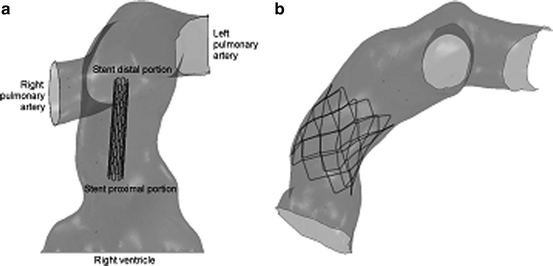
Fig. 9.2
Patient-specific RVOT/pulmonary trunk finite element (FE) model with the stent (a) before and (b) after expansion
The stent model expanded into the patient’s reconstructed implantation site resembled the outline of the arterial wall and thus resulted in an asymmetrical shape in all three directions (Fig. 9.2b), as confirmed by the biplane fluoroscopy image reconstructions (Fig. 9.3a). In particular, some of the stent struts were at an early stage of expansion, but more than half of the cells were over-deployed compared to a cylindrically deployed stent. The most expanded cells were located in correspondence to the fracture positions as detected in the patient. Furthermore, the stent was noncircular in shape; it was more expanded at the terminal sections compared to the central portion. Consequently, stresses in this stent were higher if compared to the same stent uniformly expanded in a cylinder, and the risk of reaching the endurance limit of the material was higher. The maximum stress (750 MPa) was measured in the portion of the stent where fractures were detected (Fig. 9.3b).
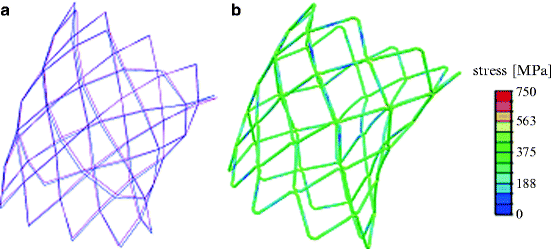
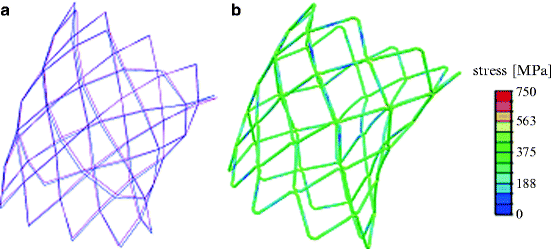
Fig. 9.3
(a) 3D stent reconstructions from fluoroscopy images in diastole (magenta) and early systole (blue) and (b) stresses distribution in the stent deployed inside the patient’s RVOT/pulmonary trunk model
A fatigue analysis was performed applying the Goodman method [18, 19], which uses a graphical approach to relate alternating stress (σ a) and mean stress (σ m) during the cardiac cycle with the material strength limits (Fig. 9.4). During the cyclic loading condition, the stent in this patient worked at high σ m and σ a, thus indicating a high number of areas at risk of fatigue failure. The portions with highest potential for fracture were nonuniformly distributed, with highest values in the areas of wire intersection in the proximal portion of the stent, where indeed such fracture occurred.
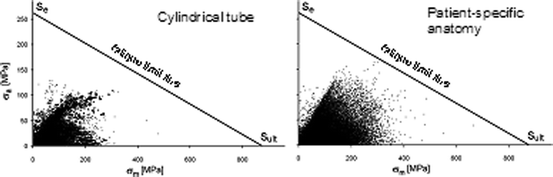
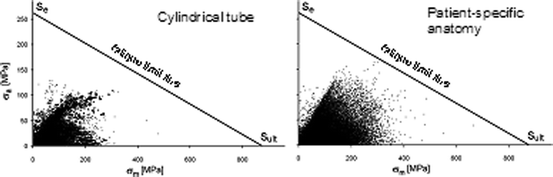
Fig. 9.4
Goodman diagrams for the fatigue analysis of the PPVI stent uniformly expanded into a cylinder and deployed inside the patient’s RVOT/pulmonary trunk model
Despite the use of this simplified FE model for the patient’s RVOT/pulmonary trunk, we can clearly see that by introducing realistic implantation site morphology into FE analysis, a more accurate prediction of nonuniform stent expansion and potential stent fracture sites can be performed compared to the conventional bench methodologies/FE analyses of free/cylindrically constrained expansion.
9.2.2.2 Pre-stenting
Placement of a bare stent into the RVOT/pulmonary trunk prior to the PPVI device has recently been shown to be a potential solution to reduce the risk of developing a stent fracture [20]. This is based on the concept that an optimized combination of different stents can enhance the strength of the system, thus decreasing the stresses on the PPVI stent. This was computationally tested by expanding two PPVI stents, one inside the other [6]. The mechanical performance of the coupled device was compared with that of a single stent, expanded and cyclic loaded at the same conditions. The stresses in the outer stent of the coupled device were similar to those of the single-valved stent. However, the stresses in the coupled device inner stent, which holds the valve, were lower when compared to the stresses in the single-valved stent. Therefore, the implantation of a stent before the PPVI device acts functionally to bolster the vessel and reduce the stresses on the valved stent, thus decreasing the risk of PPVI fracture.
9.2.3 PPVI Patient Suitability
The Melody™ valve does not fit all the sizes of patients requiring pulmonary valve treatment. New devices have to be developed to offer this minimally invasive procedure to the entire patient population. In order to address this issue, the first step was to identify the morphological characteristics of those patients who still undergo open-heart surgery.
Using 3D MR data from all patients assessed for PVR, we were able to identify subgroups of implantation sites that were suitable and unsuitable for percutaneous procedures with Melody™ and document their prevalence in our patient population (Fig. 9.5) [14]. Importantly, this analysis showed that <15% of patients requiring PVR could be treated with Melody™, with the remaining 85% requiring open-heart surgery. The majority of these patients are those with dilated, dynamic RVOT/pulmonary trunk anatomy (patients with tetralogy of Fallot and previous RVOT patches) in whom the current percutaneous device is too small. Importantly, for this morphological problem, animal testing is of very limited use, as there are no models that encompass the wide variations of anatomy seen in these patients with congenital heart disease. Hence, development of a new device to deal with the clinical problem and its translation into man is difficult using the conventional pathway of bench followed by animal testing and ultimately “first-in-man” application.
