Angioplasty expands the external elastic membrane of the stenotic segment, creating a larger total vessel area that compensates for the intimal plaque; this is similar to the positive remodeling phenomenon (Figure 38.1). Some axial plaque redistribution, proximally and distally, may also reduce the stenosis, at the expense of creating edge stenoses. Non-compliant balloons do not expand much beyond their nominal size even at high pressures (<10% overexpansion), allowing one to exert high pressure across a calcified, unyielding lesion without balloon overexpansion across the soft spots. 4. Balloon angioplasty with a balloon that is scored with blades (cutting balloon) or wires (Angiosculpt) allows the plaque to yield more easily, at lower inflation pressures. Theoretically, this increases the success of angioplasty and may be associated with less severe dissections. Also, a scored balloon is less likely to slip during balloon inflation. Standard, non-scored balloons tend to slip when inflated across a heavily fibrotic stenosis (“watermelon seeding”). Longer balloons, slower inflation, and scored balloons reduce this phenomenon. Figure 38.1 Main mechanism of action of balloon angioplasty. Angioplasty pushes the plaque and its media out, making the media larger at the treatment site. In order for this to happen, the plaque has to yield; frequently, it fractures, sometimes deeply into the media (dissection). A stent addresses all three balloon angioplasty pitfalls. It covers and seals the dissection planes or, at least, prevents them from expanding and promoting abrupt vessel closure. It has enough radial strength to prevent early (elastic recoil) and late (restenosis) vessel constriction. Stenting improves the immediate and late PCI result, but is not without its own pitfalls: Note on technical aspects The stent is mounted on a compliant balloon and is apposed to the vessel wall through balloon inflation. Prior to advancing a stent, lesion pre-dilatation with a slightly undersized balloon breaks the fibrosis and calcium, which allows stent delivery and expansion. Sometimes, “direct stenting” not preceded by balloon angioplasty may be performed. Factors favoring successful direct stenting are: absence of calcium at the target or other coronary vessels, absence of severe proximal tortuosity, non-critical stenosis, and age <70 years. The stent is usually deployed at a pressure ≤14 atm to prevent edge overinflation by the compliant stent balloon and the risk of edge dissection. After stent deployment, stent dilatation may be performed with a shorter non-compliant balloon placed within the stent and inflated at a higher pressure (15–18 atm) to ensure proper stent expansion. (First-generation DESs: sirolimus [Cypher] and paclitaxel [Taxus, Ion]; second-generation DESs: everolimus [Xience V, Promus, Synergy], zotaralimus [Resolute]). A DES consists of a stent platform similar to a BMS, with two additional components: a drug, and a polymer that controls the local drug delivery over 3–6 months. The drug inhibits cell proliferation and thus neointimal hyperplasia, with a restenosis rate of <5–10%. On the other hand, by delaying stent strut coverage, the risk of stent thrombosis used to persist beyond the first month. With the first-generation DESs, this risk of stent thrombosis remained significant for the first 12 months and mandated DAPT for 12 months. The second-generation DESs are associated with a dramatic reduction in the risk of stent thrombosis and target vessel MI (vs. first-generation DESs); the risk of stent thrombosis is very low even after clopidogrel interruption at 1–6 months. While DES does not clearly reduce mortality or MI in comparison to BMS, it dramatically reduces restenosis and repeat revascularizations. DES is superior to BMS in all subsets of patients, but is particularly superior in the following cases: In complex lesions with several of those features, the restenosis rate remains high even with DES, ≥ 10%, with an even higher rate of target vessel revascularization related to disease progression outside the stented area. BMS is very rarely used nowadays in any lesion subset as it has no advantage over DES, not even an earlier interruption of clopidogrel. In fact, 3 trials have shown that DES is superior to BMS even in high-bleeding-risk patients who stop clopidogrel at 1 month (ZEUS, LEADERS free, and SENIOR trials). Other studies have shown the safety of 1-to- 3-month DAPT vs. longer DAPT with DES (STOP-DAPT2, MASTER DAPT trials). The atherectomy devices consist of a burr mounted over a catheter. As opposed to angioplasty, which expands the media without affecting plaque volume, atherectomy acts by removing plaque; it cuts the atheroma into small microparticles that are subsequently eliminated by the reticuloendothelial system. Those microparticles are typically very small, smaller than the red blood cells, and thus do not usually plug the microcirculation, as long as each atherectomy pass is limited to <30 seconds and vasodilators are used between passes. The Rotablator achieves better immediate results than angioplasty but is associated with a similar rate of late restenosis; the heat injury initiates both negative remodeling and neointimal hyperplasia. Atherectomy is useful in heavily calcified lesions that do not yield with high-pressure balloon angioplasty, meaning that the balloon never fully expands across the lesion. The lack of full balloon expansion is different from the more common elastic recoil, wherein the balloon expands but the vessel quickly collapses back. A stent should never be used for an unyielding lesion, i.e., a lesion where the balloon does not fully expand, as the stent will fail to appropriately expand and a catastrophic stent thrombosis may occur. After balloon angioplasty fails to expand a lesion, the patient is brought back 4–6 weeks later for rotational atherectomy. It is not performed in a patient with dissection planes soon after angioplasty, as a major vessel perforation may be created by atherectomy. As an adjunct to balloon and stenting, aspiration thrombectomy catheters (suction catheters: Penumbra, Pronto catheters) can be used to aspirate thrombi. Large STEMI trials (TASTE and TOTAL) did not show any clinical benefit from the systematic use of thrombectomy and there was a small stroke hazard. The selective use of thrombectomy in STEMI patients with a large thrombus burden may still be justified. The Angiojet rheolytic thrombectomy catheter may be used for a heavy thrombus burden, and is mostly valuable in lower extremity acute limb ischemia, often as an adjunct to local thrombolysis. It is associated with a high risk of embolization. Wires with a distal basket, called distal embolic protection devices, are mainly useful during PCI of venous grafts where they reduce no-reflow and peri-PCI MI, not mortality (SAFER trial), and during femoro-popliteal atherectomy. They have not shown a benefit in acute MI: native coronary arteries have numerous side branches, such that a distal basket will prevent embolization into the distal vessel but will favor embolization into side branches, some of which are large. Also, placement of the distal protection may create arterial injury per se (especially if the basket moves during device manipulations). Stent thrombosis usually occurs within 1 month of stent placement but can occur later with DES or BMS. It is prevented with dual antiplatelet therapy and adequate stent expansion. Stent thrombosis typically leads to STEMI, the prognosis of which is much worse than that of spontaneously occurring STEMI (high clot burden), with a 15–45% risk of mortality. Timing of stent thrombosis: acute (within 24 hours), subacute (between 1 and 30 days), late (between 1 and 12 months), and very late (>12 months). Late and very late stent thromboses are rare, but may be seen with DES or BMS, especially if both antiplatelet agents are discontinued. The risk of late stent thrombosis is probably similar with second-generation DES and BMS(0.2–0.4% per year) (DAPT trial). After the first month, stent thrombosis usually occurs ≥ 7 days after clopidogrel discontinuation, implying that brief clopidogrel interruption for non-cardiac surgery may be safe. There are three categories of risk factors for stent thrombosis (Table 38.1). Table 38.1 Risk factors for stent thrombosis. Both processes start beyond 1 month (1–6 months). As opposed to stenting, the main mechanism of restenosis after angioplasty is negative remodeling, which peaks earlier than neointimal hyperplasia. When the stent is underexpanded, even a mild degree of neointimal hyperplasia may lead to significant restenosis. Underexpansion is a common factor in restenosis, especially DES restenosis, where neointimal hyperplasia is milder than with BMS. This makes stent underexpansion a major factor in >50% of DES restenoses. Neointimal hyperplasia starts to develop 1 month after angioplasty or stent placement, peaks at 3–6 months, and does not progress beyond 6–8 months with BMS or 12 months with DES. After DES, neointimal hyperplasia is milder but also more delayed than after BMS. Either way, neointimal hyperplasia does not usually progress beyond those timelines. However, progression of atherosclerosis in the nearby segments and neoatherosclerosis inside the stented segment may occur beyond those timelines (even more so with SVG stenting). Restenosis may be focal (42% of cases of BMS restenosis), diffuse (21%), proliferative (extends beyond stent margins; 30%), or totally occlusive (7%). With DES, restenosis is often focal, sometimes purely at the edges, in relation to uncovered edge disease, progressive edge disease, or neointimal hyperplasia and negative remodeling at the edges. High-pressure inflation of the compliant stent balloon may also induce edge injury and restenosis. Restenosis usually leads to recurrence of stable angina, but it may lead to MI presentation in ~10% of patients (usually NSTEMI). Therefore, while it is less catastrophic than stent thrombosis, restenosis is not always benign. In-stent restenosis is asymptomatic in ~50% of patients. Asymptomatic restenosis is associated with favorable long-term outcomes, with no clear effect on mortality or MI (vs no restenosis). In DES trials, routine angiography and PCI of restenosis was not associated with improved outcomes (vs. clinically-driven PCI). Thus, after PCI, neither surveillance stress testing or angiography nor treatment of asymptomatic restenosis is indicated. Also, FFR may be used to safely defer treatment of restenosis>50% at >6 months after the index PCI. Restenosis is treated with balloon angioplasty if the main problem is technical (i.e., the stent is underexpanded) or if the in-stent restenosis is focal. Focal restenosis has good outcomes with balloon angioplasty and a recurrent restenosis rate of ~20%. A cutting balloon may also be used. Conversely, diffuse in-stent restenosis and edge restenosis are treated with DES placement within the previously placed BMS or DES (DES stent “sandwich”). Alternatively, a drug-coated balloon may be used (available in Europe). IVUS helps determine the mechanism and guide the treatment of in-stent restenosis. Sandwich DES stenting for DES restenosis is associated with a ~15–20% risk of recurrent restenosis. Placing a heterogeneous sandwich DES, i.e., a different type of DES within the restenosed DES, is not clearly superior to a homogeneous sandwich DES. Beyond 1 year, atherosclerosis starts to grow over the stent neointima; this is referred to as neoatherosclerosis. As opposed to neointimal hyperplasia, neoatherosclerosis is characterized by a lipid-laden plaque with foamy macrophages and a fibrous cap. It tends to appear earlier and more frequently with DES (30–50% at 1–2 years) than BMS (16% at 5 years). It explains late restenosis and partly explains the very late stent thrombosis seen with both types of stents. Table 38.2 Recurrent target vessel disease/angina after stenting. a Also, necrotic plaque protrusion through the stent (more so in ACS) may predispose to restenosis or thrombosis. Table 38.3 Peri-PCI antithrombotic therapy. GPI: glycoprotein IIb-IIIa inhibitors According to clinical trials, multivessel PCI compares favorably with CABG if the stenoses are technically amenable to PCI. The presence of a chronic total occlusion or one or more technically difficult lesions or long lesions (angiographic SYNTAX score ≥ 23) should favor CABG, especially because CABG provides a more complete revascularization. Diabetes favors CABG as well, as CABG provides lower rate of repeat revascularization and better survival compared with PCI in diabetes. Normally, the stent should be implanted from normal reference to normal reference if possible (starting 2 mm before and 2 mm after the lesion); this will avoid edge dissections and edge plaque shift. The reference should have <40% plaque burden on IVUS. In case of diffuse disease with tandem lesions in series, the most severe lesions are stented (spot stenting), while the intermediate ones may be left untreated. FFR may be used to guide PCI in diffuse disease: stent the areas where the pressure drop is largest, as assessed by FFR pullback tracing. FFR may be reassessed after stenting each lesion. If possible, the distal lesion should be stented first, followed by the proximal lesion; this obviates the need to cross the proximal stent with the distal stent. While DES length does not increase restenosis rate as strongly as BMS length, very long stenting (“full metal jacket”), even with DES, is associated with a high risk of restenosis and is preferably avoided. Furthermore, long stenting may compromise future placement of bypass grafts and is preferably avoided, especially in the LAD. Side branch (SB) narrowing may occur after main branch (MB) PCI. This is related to plaque shift (snowplow phenomenon). The likelihood of this depends on the degree of ostial SB narrowing. When the ostium of the SB has >50% stenosis (= true bifurcation lesion), there is a risk of SB occlusion with MB stenting (14–35%). Bifurcation stenoses are approached as follows: FFR studies have shown that SB narrowing that occurs after stenting is often functionally non-significant (~75% of the time). The narrowing is partly due to a benign geometric kinking of SB when MB is straightened by the stent. In addition, provisional rather than planned stenting is associated with a lower periprocedural MI and similar long-term outcomes. Stent-induced occlusion of a large branch may result in significant myocardial ischemia/necrosis, though in most patients the long-term prognosis is good and many initially occluded SBs are patent on follow-up. CTO implies a total coronary occlusion that is >3 months old. An occlusion may develop progressively over time and lead to an insidious, chronic angina presentation. It may also develop or progress acutely/subacutely leading to STEMI or NSTEMI; when this acute occlusion is not treated early on, it enters the category of CTO 3 months later. Well-developed collaterals may provide flow equivalent to a 50–90% stenosis, which helps maintain myocardial viability and prevents resting myocardial ischemia. When the coronary occlusion develops progressively rather than abruptly, the myocardial function may be normal at rest or depressed, but not infarcted (hibernating rather than necrotic myocardium). Hibernation is distinguished from infarction by the persistence of angina, lack of Q waves, lack of echocardiographic thinning, and ischemia or >50% uptake on nuclear imaging. MRI may be used when viability is questionable. CTO PCI is indicated in symptomatic patients whose angina is refractory to medical therapy. Four randomized trials have shown that CTO recanalization does not reduce the long-term risk of death or MI. It does not improve overall wall motion or EF (Explore trial), and does not clearly improve the segmental motion of dysfunctional segments (REVASC trial). It only improves angina and quality of life, as per Euro-CTO trial. In addition, opening an occlusion that was associated with a large transmural infarct (STEMI or Q-wave MI), and a myocardium that is now akinetic and minimally ischemic, is not beneficial and is not indicated (OAT trial). The success rate of CTO PCI can exceed 80% when using a hybrid algorithm, with mortality rates of 0–2% (see Question 7). Restenosis rate is markedly reduced with DES (~10%). Six main features predict failure of the antegrade approach to CTO PCI (the first 5 are J-CTO score): Polymer-coated wires may be used in a functional CTO to slide through the microchannels. Otherwise, drilling wires with progressively heavier tips are used, with the support of a catheter advanced to the occlusion site. Subintimal passage and distal reentry into the true lumen is the preferred technique when multiple adverse features are present, especially length >2 cm. Double arterial access with engagement of the contralateral coronary is usually necessary. Contralateral injections allow collateral retrograde filling of the index vessel and thus proper visualization of the wire progression. Antegrade vessel opacification usually ceases once the wire and catheter are advanced to the CTO site, making contralateral guidance critical in most cases. If heparin is used during PCI, the femoral sheath is removed when ACT <170 seconds (~2–3 hours after PCI). If bivalirudin is used, the sheath is removed 1.5 hours after the infusion is discontinued, the half-life being 25 minutes in patients with normal renal function; wait 2–3 hours in advanced renal failure (GFR <30 ml/min), where the half-life is ~1 hour. If enoxaparin is used, remove the sheath 6–8 hours after the last SQ dose of enoxaparin. General duration of manual pressure over the femoral artery: 10 minutes if a 4 Fr sheath is used and 5 more minutes for each higher French size. 4–6 Fr sheaths are usually used for diagnostic coronary angiography; ≥ 5 Fr sheaths are used for PCI. In general, the patient is maintained flat in bed 4 hours after 4 Fr sheath removal, and 6 hours after 6 Fr sheath removal. A vascular closure device may be used (polyethylene glycol [Mynx], collagen [Angio-Seal], or suture-based device [Perclose]). When a closure device is used, the sheath is pulled out immediately after PCI, even while anticoagulation with heparin or bivalirudin is therapeutic; prolonged occlusive manual compression is not needed and the patient can ambulate 2–4 hours later. However, while convenient, closure devices do not reduce local vascular and bleeding complications. They may, in fact, increase local vascular complications (small risk of arterial occlusion). It is safe to restick the same artery immediately after Mynx or Perclose closure. It is preferred to wait 3 months after Angio-Seal deployment, but, if needed, a restick may be performed 24 hours after deployment, 1 cm proximal to the initial stick. If a radial access is used, heparin is given regardless of PCI to prevent radial occlusion, which occurs in ~3-5% of cases (UFH 5000 units or 50 units/kg). If an intervention is planned, a therapeutic dose of bivalirudin may be given instead of UFH. The sheath is removed at the end of the procedure and hemostasis achieved with the use of an inflatable transradial band. Patent hemostasis should be used, meaning that the transradial band is only inflated with enough volume to stop the bleeding, not more (the transradial band is only inflated with 1 ml of air beyond the occlusive volume); pulse oximetry should continue to show a waveform even with manual ulnar occlusion, confirming antegrade radial flow. The band is kept untouched for 60–90 min then deflated progressively (~3 ml every 15 min). Patients may be hospitalized for 1 day after PCI, during which they are monitored for access complications, contrast nephropathy, and periprocedural MI. Low-risk patients without major comorbidities (HF, renal failure) undergoing a non-complex elective PCI through a radial access may be discharged on the day of the procedure; this may be extended to transfemoral procedures with no access complication (EPOS trial). Beyond 6 hours after PCI, the likelihood of complications is very low. Mortality after diagnostic angiography is ~0.1%, but this increases significantly in severe AS or left main disease (up to 0.5%), where any transient arrhythmia or hypotension, sometimes induced by sedation, may initiate an irreversible, vicious circle of myocardial ischemia and further hypotension. Also, in left main disease, the catheter abutting a left main plaque may create a left main dissection. Mortality after PCI is <1% (~0.5%). Complications and mortality after PCI are related to both the patient and the lesion characteristics: Patient characteristics are a stronger determinant of complications than lesion characteristics. A coronary dissection is seen after 30% of balloon angioplasties. It may also occur at the edges of a stent, when overinflation of the stent’s balloon damages the edges. The frequency of stent edge dissection is 5% angiographically, 10% by IVUS, and 30% by OCT (the latter frequently detects some small and clinically insignificant dissections). Coronary dissection is more common in diffuse or calcified disease. Coronary dissection may also result from wire manipulations or from a deep-seated guiding catheter. Dissections typically propagate distally (antegradely). When occurring after plain balloon angioplasty, types A and B dissections are often benign and do not affect outcomes; they may be treated with prolonged low-pressure balloon dilatation (balloon sized 1:1 to the vessel). After stenting, any angiographic edge dissection (except possibly type A) is worrisome as it increases the risk of stent thrombosis and warrants intracoronary imaging or further stenting. On IVUS or OCT, superficial dissections that do not reach the media, extend to less than one quadrant, and are <5 mm in length are benign. Edge dissections are frequently seen on OCT and do not necessarily warrant further stenting if they have benign features. Whether with angioplasty or stenting, angiographic dissections types C through F carry an increased risk of thrombosis and vessel closure and are treated with further stenting. Stent distally to stop the propagation of the dissection, then proximally to seal the source of dissection; when the dissection extends very distally in a spiral fashion, it may not be possible to stent distally and one may only stent the proximal entry point to stop the expansion of the false lumen and collapse it. When the guide dissects the ostial left main or RCA and leads to hemodynamic compromise, proximal stenting may need to be performed first to relieve the extent of ischemia. In small or severely tortuous vessels where stenting is not possible, perform prolonged low-pressure balloon inflation with a well-sized balloon (not undersized). Since type C dissection may, in fact, be a localized perforation that manifests 24–48 hours later, the patient needs to be carefully monitored and an echo needs to be obtained ± repeated 24 hours later, looking for hemopericardium. 3. Intramural hematoma is a bleeding inside the media that displaces the internal elastic membrane inward without a dissection entry or exit point. It may be iatrogenic, post-stenting, or spontaneous (SCAD). On IVUS, it appears like an echolucent crescent behind the intima/plaque, sometimes giving the impression of an echolucent plaque. Angiographically, it may simulate a dissection or may appear smooth, simulating a refractory spasm. It is usually treated with further stenting at a low pressure. Two types of periprocedural MI may be seen: No reflow is reduced with the use of IIb–IIIa inhibitors, and with the use of filter devices during venous graft interventions. Once it occurs, it is treated with intracoronary adenosine, verapamil, or nitroprusside. According to the 2018 ACC universal MI definition, periprocedural non-Q-wave MI is defined by an increase in troponin I to >5× the upper limit of normal, along with: (1) prolonged chest pain, or (2) ischemic ST changes or new Q waves, or (3) new wall motion abnormality, or (4) angiographic evidence of procedural, flow-limiting complications. However, as opposed to the more recognized prognostic value of post-PCI CK-MB elevation (>3–10× normal), the degree of troponin rise used in the definition does not clearly carry an independent negative prognostic value. Therefore, an expert consensus document has suggested the use of CK-MB elevation ≥ 10× normal or troponin I elevation ≥ 70× normal to define periprocedural MI. Lesser degrees of CK-MB elevations, as well as isolated troponin elevation post-PCI, are of questionable prognostic value. The above definition applies for patients with normal baseline biomarkers. If the biomarker values are elevated but stable or falling, periprocedural MI is diagnosed by ≥ 20% reincrease of a previously downward trending troponin or CK-MB value. In patients who present with elevated biomarkers that are on the rise and who undergo PCI within 24 hours of presentation, periprocedural MI is defined by the occurrence of procedural thrombotic/occlusive complications, prolonged ischemic symptoms ≥ 30 minutes, new ischemic ST abnormalities, or Q waves after PCI, along with an increase of the next troponin I or CK-MB by at least 50% (this is the definition used in trials, e.g., ACUITY, EARLY ACS, CHAMPION Phoenix trials). The increase in biomarkers reflects a more extensive and unstable atherosclerotic burden that predisposes to future ischemic events. The latter is likely the main reason for survival impairment, rather than the minor injury induced by PCI. This explains why the unadjusted increase in mortality with periprocedural MI is more striking than the adjusted increase in mortality, and that only marked biomarker rise post-PCI carries an independent prognostic value. Note that spontaneous NSTEMI carries a much stronger independent prognostic value than periprocedural MI, despite less biomarker elevation (3× higher mortality, according to ACUITY trial data). In fact, in NSTEMI, the adverse outcome is related not only to the minor myocardial injury but to the ruptured, active plaques that carry a high risk of a large infarction, which is not the case in controlled periprocedural MI. Large periprocedural MI (CK-MB>5-10x normal) currently occurs in ~2.5% of PCI cases and the incidence is reduced with the current antiplatelet regimens. Most periprocedural MIs are clinically silent. Cardiac markers should be checked 12–24 hours after all complicated PCIs and in patients with ischemic symptoms during or after PCI. Cardiac markers may also be checked routinely, 12–24 hours after any PCI (class IIa recommendation). A coronary perforation may be secondary to: Two procedures are to be immediately and concomitantly performed in case of a type 3 perforation, and this may require two physicians: (i) pericardiocentesis, and (ii) prolonged balloon inflation proximal to the perforation site. Afterwards, a second femoral access (6 or 7 Fr) is obtained and a second guiding catheter is used to double-engage and double-wire the perforated coronary artery. A Graftmaster or PK Papyrus covered stent is advanced through the second guiding catheter while the balloon is still inflated through the first guiding catheter, the balloon being only briefly deflated to advance the covered stent to the perforation site. Even short periods of balloon deflation may lead to hemodynamic compromise; thus, the double-guide technique obviates the need for a prolonged interval without an inflated balloon while the covered stent is advanced. Emergent surgery may be required if the perforation fails to seal with balloon inflation and a covered stent cannot be used. As an alternative to the second guide, a Guideliner guide extension may be advanced through the original guide and used to wedge and occlude the perforated artery, if it is small. The guide extension also allows delivery of the lower profile PK Papyrus stent (5 Fr), without requiring a second access. A non-sealing perforation of a branch or distal vessel, which is usually a wire-induced perforation, may be treated by distal injection of: (i) small coils or (ii) fat particles. An over-the-wire balloon catheter or microcatheter is advanced distally and wedged into the distal vessel, occluding it. Negative suction is applied to the lumen of this catheter, using a 20 ml syringe; this negative suction may seal the perforation. If not, coils or fat particles are advanced through the catheter. Fat particles are harvested from a cut made in the groin area (fat is cut into pieces <1 mm and mixed with saline). Thrombin may also be used, but is more risky as it may leak in the proximal vessel; thrombin is injected through the lumen of an over-the-wire balloon catheter, the balloon being positioned distally and inflated to prevent a proximal leak. Anticoagulation is not immediately reversed during the above maneuvers (e.g., prolonged balloon inflation), as this may lead to extensive coronary thrombosis.
38
Percutaneous Coronary Interventions and Complications, Intra-Aortic Balloon Pump, Ventricular Assist Devices, and Fractional Flow Reserve
I. Major coronary interventional devices
A. Balloon angioplasty
1. Mechanisms of action
2. Pitfalls
3. Compliant vs. non-compliant balloons
5. Cases where plain balloon angioplasty is sometimes performed, without stenting
B. Bare-metal stents (BMSs)
C. Drug-eluting stents (DESs)
D. Rotational atherectomy (Rotablator) and orbital atherectomy
E. Aspiration thrombectomy and distal embolic protection
II. Stent thrombosis, restenosis, and neoatherosclerosis
A. Thrombosis
B. Restenosis
1. Mechanisms
Stent underexpansion, stent malapposition, edge dissection, edge plaque shift (especially if the plaque is necrotic and active)
Acutely, a delayed loading of ADP receptor antagonist is a risk factor for acute thrombosis. Non-compliance with the recommended duration of DAPT is the most important risk factor for subacute and late stent thrombosis. Clopidogrel non-responsiveness increases the risk of stent thrombosis, which remains low, in absolute terms, in stable CAD PCI. The latter is rarely a standalone factor in stent thrombosis
2. Neointimal hyperplasia
3. Patterns of restenosis
4. Clinical presentation
5. Treatment
C. Neoatherosclerosis
D. Mechanisms of recurrent target vessel disease after stenting (Table 38.2)
III. Peri-PCI antithrombotic therapy (see Table 38.3)
Stable CAD PCI
Planned PCI
Bivalirudin: no clear reduction in bleeding vs UFH in modern trials, possibly more acute stent thrombosis
Dose of UFH:70–100 units/kg to a goal ACT 250–300 s if GPI is not used
50–70 units/kg to a goal ACT 200–250 s if GPI is used
Ad-hoc PCI
NSTE–ACS
Antiplatelet therapy
Anticoagulant therapy
Definitely withhold in patients with a high probability of needing CABG
or load with 600 mg of clopidogrel in the cath lab if no clopidogrel has been given
or load with prasugrel 60 mg in the cath lab (even if clopidogrel has been given)
or load with ticagrelor 180 mg in the cath lab (even if clopidogrel has been given)
UFH pre-catheterization and during PCI
or UFH pre-catheterization and switch to bivalirudin during PCI
or Fondaparinux 2.5 mg SQ QD pre-catheterization with standard-dose UFH or bivalirudin during PCI
or Enoxaparin pre-catheterization. If the patient received 1 mg/kg SQ enoxaparin within 8 h of PCI and has already received two doses of enoxaparin, no additional anticoagulation is needed during PCI. If enoxaparin was given 8–12 h ago or only one SQ dose was given, add 0.3 mg/kg IV bolus during PCI Avoid switching between UFH and enoxaparin. The switch to bivalirudin is, however, acceptable
STEMI
IV. Complex lesion subsets
A. Multivessel PCI
B. Long lesions and diffuse disease (multiple lesions in series)
C. Bifurcation lesions
D. Chronic total occlusion (CTO)
V. Sheath management
VI. Post-PCI mortality and coronary complications
A. Mortality
B. Coronary dissection
1. Angiographic types of coronary dissection
2. Treatment
C. Periprocedural MI
D. Coronary perforation
1. Causes of coronary perforation
2. Classification and management of coronary perforations
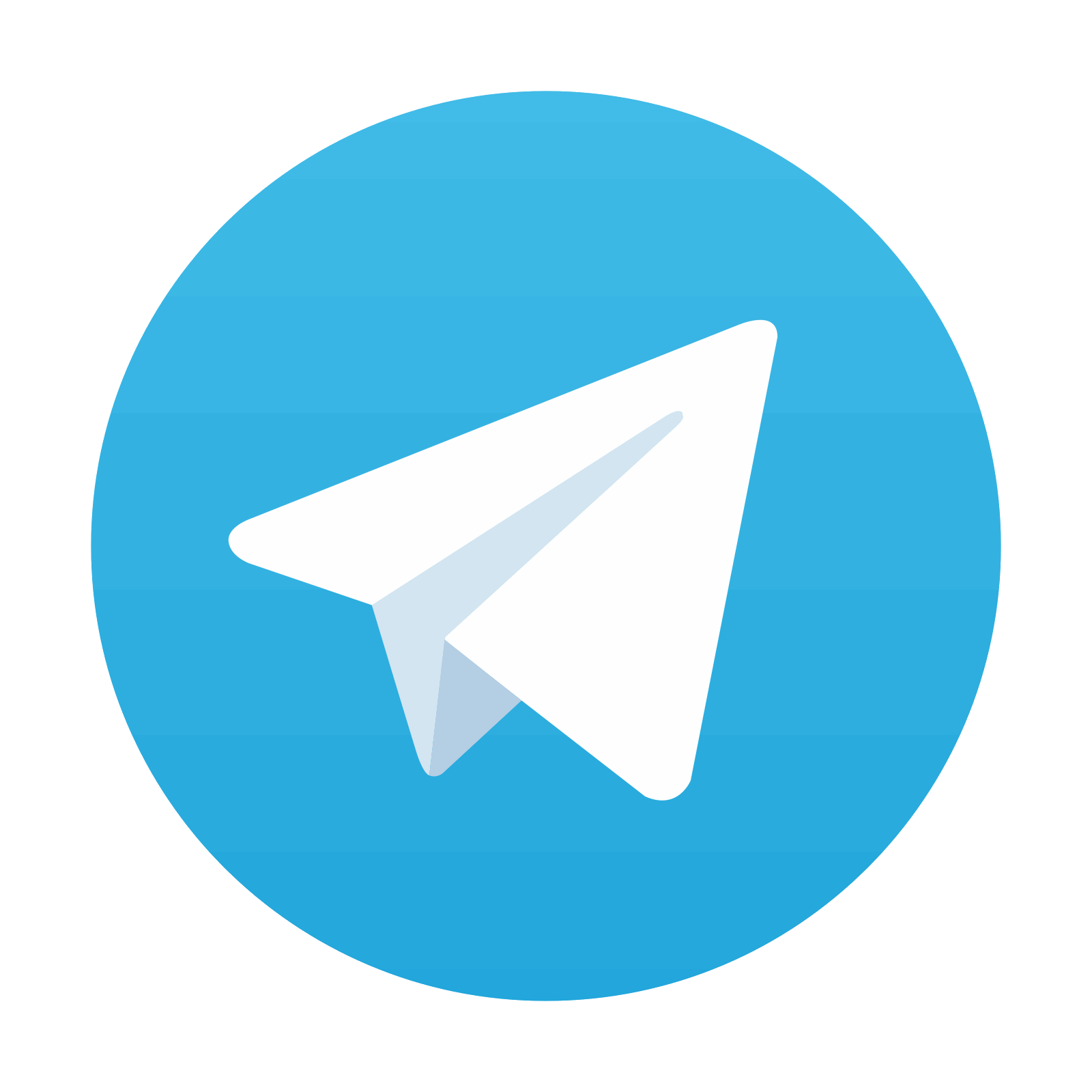
Stay updated, free articles. Join our Telegram channel

Full access? Get Clinical Tree
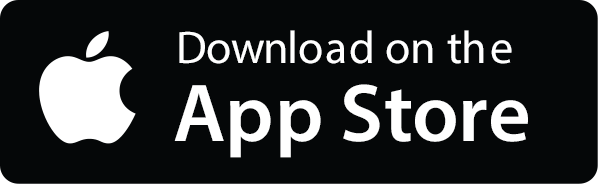
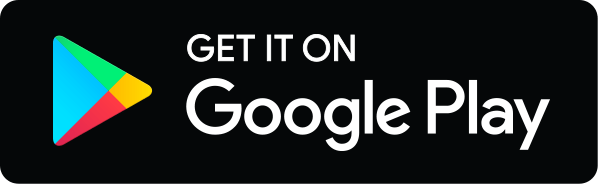