Abstract
Persistent pulmonary hypertension is the term used to describe a spectrum of pathophysiological changes that result in hypoxic respiratory failure. A number of underlying physiologies contribute to this overarching syndrome, and understanding the individual components of the disorder potentially allows the clinician to better target therapy and achieve the desired response with a greater success rate and in a shorter time frame. Understanding the underlying patient variance genetically and physiologically allows individualization and targeting of care to each patient, which is an integral part of the concept of precision medicine.
Keywords
inhaled nitric oxide, milrinone, persistent fetal circulation, pulmonary hypertension, sildenafil, systemic hypotension
- •
The primary pathophysiologic event in persistent pulmonary hypertension of the newborn (PPHN) occurs when the pulmonary vascular resistance fails to appropriately decrease at birth.
- •
PPHN is made up of a number of underlying pathophysiological subgroups with the clinical presentation dependent on the emphasis of each of these elements.
- •
There are multiple treatment modalities available for PPHN and a logical treatment strategy requires an understanding of the underlying pathophysiology.
- •
Appropriate management will include care of the underlying condition, respiratory management, treatment of myocardial impairment, use of inotrope/vasopressors, and use of pulmonary vasodilators.
- •
Cardiac ultrasound is an important assessment tool, allowing understanding of the underlying physiology, targeting of treatment, documentation of response to treatment, and identification of when to wean therapy.
The primary pathophysiologic event in persistent pulmonary hypertension of the newborn (PPHN) occurs when the pulmonary vascular resistance (PVR) fails to appropriately decrease at birth. The resultant low oxygen saturation is poorly tolerated after birth and affected neonates may develop multi-organ dysfunction/failure because of hypoxemia. The clinical manifestations of raised pulmonary pressure will depend on the severity of pulmonary vasoconstriction, the presence and magnitude of, or absence of, shunting through the patent ductus arteriosus (PDA) and foramen ovale, the relative pressure/impedance at each end of the ductus arteriosus, the contractility and efficacy of each of the ventricles, and the presence of associated morbidities.
The severity of PPHN can run the full clinical spectrum from respiratory distress with mild hypoxemia to severe hypoxemia and cardiopulmonary instability necessitating advanced intensive care support. Treatment requirements vary according to the severity of the pathophysiology and the individual components of the condition. Consequently, a good understanding of the pathophysiology is essential to allow targeting of the many different treatment modalities available.
Pathophysiology, Hemodynamics and Treatment Targets
The primary underlying pathophysiological abnormality in PPHN is raised PVR or a failure of the normally high in utero pulmonary pressures to appropriately fall in the postnatal period. Initiating factors include hypoxemia and the associated metabolic acidosis in the perinatal and/or immediate postnatal period, as both of these conditions are potent pulmonary vasoconstrictors. The clinical manifestations of raised PVR will depend on other factors including whether the fetal shunts are patent, the status of left and right ventricular function, the systemic blood pressure, the additional impact of mechanical ventilation on pulmonary blood flow, and the volume status. In many cases there will be sustained or advancing hypoxemia, ventilation perfusion (VQ) mismatch, and acidosis, which contribute to, or further enhance, the increased PVR. In addition, high PVR in association with mechanical ventilation can impair oxygenation and reduce the preload to the left ventricle, resulting in inadequate left ventricular function, and reduced systemic perfusion. Finally, sustained mild/moderate in utero hypoxia may result in remodeling of the pulmonary vasculature with smooth muscle cell migration into the more distal pulmonary vessels, making the condition, at least initially, less responsive to the administration of pulmonary vasodilators.
Understanding the contribution of the individual pathophysiological elements potentially allows a more targeted approach to care. For example, if the presentation is due to asphyxia with associated myocardial impairment, in addition to administering pulmonary vasodilators, cautious volume administration, early institution of inotropic support and optimization of ventilation to improve cardiorespiratory function are priorities. Alternatively, if the patient presents with open fetal channels with right to left shunting and adequate myocardial function, using pulmonary vasodilators and careful supportive care may be most useful.
In PPHN, there are generally two treatment targets: relaxation of the pulmonary vasculature, and addressing myocardial dysfunction and the abnormalities of peripheral vascular regulation. The hemodynamic derangements are most frequently secondary to increased pulmonary vascular pressure and, if present, to myocardial dysfunction. The use of drugs with inotropic, vasopressor, and/or lusitropic effects may alter the hemodynamic presentation during management. Therefore, it is imperative to understand the cardiovascular disturbances in PPHN in order to identify and address them in a timely manner. Following the performance of an echocardiographic study to rule out congenital heart disease, repeated bedside cardiac ultrasound examinations by the neonatologist caring for the patient play an indispensable role in recognizing the underlying elements of PPHN, and in assessing the hemodynamic response to treatment. Severe pulmonary hypertension increases right ventricular afterload, which may result in right ventricular failure unless the right ventricle can offload via a nonrestricting PDA. In addition, the elevated right ventricular pressure often causes right-to-left shunting through the patent foramen ovale (PFO) as well, and right-to-left shunting through both fetal channels worsens hypoxemia. Because of a high degree of interdependence between the right and the left ventricles, changes in right ventricle size and geometry often impacts left ventricular geometry and function. Thus, in addition to decreased left ventricular preload, compromised left ventricular geometry and function contribute to lower systemic blood pressure and low cardiac output necessitating the use of cardiovascular support.
Understanding the role of the PDA in this setting is of great importance. Increased right-to-left shunting through the PDA worsens the hypoxemia and acidosis in the organs supplied by the post-ductal circulation, which in turn results in further pulmonary vasoconstriction. On the other hand, shunting through the fetal channels may support cardiac output and maintain cerebral perfusion when the preload to the left heart is severely compromised. Finally, right ventricle failure can occur when the ductus arteriosus is closed or restricted, and re-opening of the ductus arteriosus with prostaglandins can be a life-saving immediate supportive measure in these cases.
The hemodynamic presentation in PPHN is complex and variable as it is affected by cardiovascular, pulmonary, and other factors, as well as by the treatment and the individual patient’s response. Understanding these factors, their interdependence, and their impact on treatment is of great importance and likely affects patient outcomes. Therefore, the clinician needs access to reliable, longitudinal bedside information on the cardiorespiratory status and the hemodynamic changes during the course of the disease in each patient. The use of point-of-care ultrasound (POCU) significantly improves the clinician’s ability to obtain the required information (see Chapter 10 ). By using POCU, the physician will have an understanding of the hemodynamic status of each infant and the response to treatment rather than only relying on standardized algorithms for management of these often critically ill neonates.
Principles of Management of Persistent Pulmonary Hypertension of the Newborn
The primary therapeutic goals are to lower the PVR while supporting the cardiovascular and respiratory function. Management of PPHN is accomplished by utilizing a careful, stepwise approach with progressive escalation of treatment if necessary. Some infants who present with mild clinical features of PPHN are candidates for close observation initially with careful, comprehensive cardiorespiratory monitoring, as the presentation may evolve into a more severe form of PPHN. The overall approach focuses on normalizing the cardiopulmonary adaptation while avoiding/minimizing lung injury and the potential adverse effects of ventilator support on the systemic perfusion. Variations in severity, and thus the clinical presentation of PPHN, result in a wide range of variation in management of PPHN in clinical practice.
Supportive Care
When PPHN is associated with parenchymal lung disease or systemic illness, the treatment should also target the accompanying morbidities. Exposure to environmental factors, like noxious stimuli, tracheal suctioning, heel pricks, and excessive noise may augment pulmonary vasoconstriction and increase the frequency and severity of hypoxemic episodes, the hallmarks of unstable pulmonary vasoregulation. Maintenance of normothermia and correction of metabolic, electrolyte, and hematologic abnormalities (e.g., acidosis, hypoglycemia, hypocalcemia, and polycythemia) are clearly of importance. However, pH, partial pressure of O 2 (PaO 2 ), and CO 2 (PaCO 2 ) are to be kept in the normal range as overcorrection of these parameters may be more harmful than beneficial.
Sedation, usually with opioids and benzodiazepines, is widely used to minimize fluctuation in oxygenation and to facilitate ventilation, but it is not evidence-based nor is it without side effects. In addition to the potential for the development of systemic hypotension, generalized edema, reduced cerebral blood flow, and deterioration of lung function with the prolonged use of sedation, the usefulness of sedative agents in the setting of PPHN has not been subjected to well-designed studies. Therefore, sedation should be used with appropriate caution in neonates with PPHN. Neuromuscular blockade has also been commonly used as an adjunctive therapy to limit fluctuations of oxygenation due to patient interference with mechanical ventilation. Commencement of muscle relaxation can suddenly change preload and afterload, resulting in rapid cardiovascular changes. If an infant is dependent on systemic blood pressure to limit right-to-left ductal shunting, this can cause a sudden decrease in oxygen saturation. Of note is that the use of neuromuscular blockers has been linked to an increased incidence of hearing impairment in survivors of PPHN; however, the mechanism of this association is unclear. According to studies performed before the routine use of inhaled nitric oxide (iNO), this treatment was also associated with increased mortality. Short-term administration of neuromuscular blockers may be considered in infants with severe and unstable clinical presentation of PPHN despite the appropriate use of sedation. Finally, neuromuscular blockers should never be administered without provision of appropriate sedation.
Mechanical Ventilation and Surfactant
Many infants with severe PPHN require invasive ventilatory support. The primary goal of mechanical ventilation is to ensure adequate lung inflation and thus enhance the effectiveness of inhaled vasodilators (oxygen and iNO). Indeed, in patients with significant parenchymal lung disease, administration of inhaled pulmonary vasodilators without lung recruitment results in a suboptimal vasodilatory effect. In addition, as parenchymal lung significantly contributes to hypoxia in PPHN, appropriate ventilator management is clearly warranted. However, the use of inappropriately high mean airway pressure, especially after surfactant administration, can reduce cardiac output and therefore worsen hypoxia. Consequently, a role for noninvasive ventilation in the management of PPHN in neonates with less severe presentation has been suggested and the advent of the possibility of delivering iNO noninvasively has further facilitated this strategy. The incidence of lung disease associated with PPHN has changed over the years. The overall incidence of meconium aspiration syndrome has declined, coinciding with a decrease in the number of post-term pregnancies. In contrast, respiratory distress syndrome (RDS) in late preterm neonates delivered by elective or indicated Cesarean section at 34 to 36 weeks of gestation and pneumonia/sepsis have become more frequent preludes to PPHN.
Vasodilator Therapy in Persistent Pulmonary Hypertension of the Newborn
Oxygen and Carbon Dioxide
The primary goal of PPHN treatment is to improve oxygen delivery to the tissues and decrease PVR by selective pulmonary vasodilation without compromising systemic hemodynamics. Oxygen itself can potentially help achieve both these goals. However, oxygen is a toxin at higher doses, and it may be harmful when administered in excess. Oxygen is a potent and selective pulmonary vasodilator; thus increased oxygen tension results in a reduction of PVR. Among other effects, O 2 stimulates endothelial NO synthase and NO production and contributes to pulmonary vasodilatation at birth. However exposure to hyperoxia results in the formation of oxygen free radicals, such as peroxides, superoxide, hydroxyl radical, and singlet oxygen, which all can cause lung injury.
In animal studies, an arterial PaO 2 of less than 45 to 50 mm Hg results in increased PVR in newborn calves and lambs. In contrast, maintaining PaO 2 greater than 70 to 80 mm Hg does not result in any additional decrease in PVR in both control lambs and lambs with PPHN. In lambs with PPHN, PVR increases substantially in severe hypoxemia with a PaO 2 of less than 13.9 mm Hg, whereas vascular resistance declines more gradually at PaO 2 levels greater than 59.6 mm Hg. Similarly, PVR increases below an oxygen saturation of 85%. Therefore, a pre-ductal PaO 2 of 60 to 80 mm Hg and a pre-ductal oxygen saturation of 90% to 97% would seem to be reasonable targets during the treatment of neonates with PPHN.
Carbon-dioxide-related changes to the pulmonary vascular tone differ between the normal and injured lung, and they also vary depending on pulmonary pressures and the presence of endogenous NO. Of importance, the effect of hypoxia on the pulmonary vasculature is more pronounced than any effect of pH and/or CO 2 . It is also important to consider the acid-base status in the context of O 2 administration and its effect on pulmonary vessels. Animal studies have shown that lower pH exaggerates the vasoconstrictive response of the pulmonary vessels to hypoxia. Accordingly, the acceptable PaO 2 range devoid of the hypoxic pulmonary vasoconstrictor response, is not absolute, as it is affected by the acid-base status. Therefore, avoiding acidosis offers some protection against pulmonary vasoconstriction in response to hypoxia.
In a stepwise approach to the management of PPHN, the optimization of O 2 administration and appropriate ventilation to target SpO 2 and CO 2 levels to maximize pulmonary vasodilation is the first step preceding the initiation of more lung-specific therapies, such as surfactant and iNO administration. POCU allows identification of infants who have early evidence of elevated pulmonary pressure and cardiac dysfunction, flagging those who should have blood gases and O 2 saturation carefully monitored, and in whom avoidance of sudden decreases in O 2 saturation and/or hypercapnia encourages normalization of circulatory transition.
Inhaled Nitric Oxide
NO is a potent endothelium-derived factor that has diverse effects on the pulmonary circulation, including vasodilation, pro-angiogenic, anti-inflammatory, and anti-oxidant properties. Treatment with iNO is one of the cornerstones of PPHN management. Inhaled NO reaches the alveolar space and diffuses into the vascular smooth muscle of the adjacent pulmonary arteries, where it causes a cyclic guanosine monophosphate (cGMP)-mediated vasodilation. In the pulmonary arteries, it is rapidly bound to hemoglobin, restricting its effect to the pulmonary circulation, without any clinically relevant effect on the systemic circulation. Inhaled NO is preferentially distributed to the ventilated segments of the lungs underscoring the importance of optimal lung recruitment prior to the initiation of iNO administration. Accordingly, iNO administration results in an improved ventilation/perfusion ratio, decreased intra-alveolar shunting and improved oxygenation. Infants who have evidence of raised PVR with decreased pulmonary blood flow and an open PDA with predominantly right-to-left shunting are most likely to have a dramatic response to iNO. Importantly, infants with low systemic blood pressure, often with accompanying myocardial impairment and a significant respiratory component contributing to the increased FiO 2 requirements, are less likely to respond well to iNO, and require more extensive cardiovascular and respiratory support.
The role of iNO in term and near-term infants with oxygenation failure is well known. Although iNO reduces the need for extracorporeal membrane oxygenation (ECMO), mortality without the use of ECMO is not reduced. Oxygenation improves in approximately 50% of infants receiving iNO and the response is dependent on the underlying pathophysiology of the particular infant. Targeting iNO to infants with responsive physiology will significantly increase the response rate. If a patient responds, the oxygenation index (OI) decreases within 30 to 60 minutes after commencing therapy and PaO 2 rapidly increases. The apparent lack of benefit of iNO on the survival of term neonates with PPHN is most likely due to the fact that term neonates would not be allowed to die of hypoxic respiratory failure without a trial of ECMO in developed countries where these trials were conducted. ECMO is very effective in reducing mortality in term infants with PPHN.
Early Introduction of Inhaled Nitric Oxide in Persistent Pulmonary Hypertension of the Newborn Management
The severity of PPHN is commonly assessed by calculating the OI, which reflects a combination of the ventilator support applied and oxygenation.
OI = MAP × FiO 2 × 100 / PaO 2
( MAP, Mean airway pressure; FiO 2 , fraction of Inspired O 2 ; PaO 2 , partial pressure of O 2 )
Hypoxemic respiratory failure has been classified as mild (OI ≤ 15), moderate (OI > 15 to 25), severe (OI > 25 to 40) and very severe (OI > 40). Introduction of iNO therapy at a lower OI and thus usually earlier in the course of the disease may be beneficial in the management of PPHN. Initiating therapy before alveolar atelectasis from lung disease or ventilator-induced barotrauma or O 2 toxicity occurs, could potentially lessen lung injury with a consequent reduction in the duration of respiratory support and/or length of hospital stay. However, it should be emphasized that adequate alveolar recruitment is a prerequisite for effective iNO delivery as the response to iNO in PPHN is more effective when lung recruitment is optimal. The initial notion that starting iNO for respiratory failure in the earlier stages of disease evolution (targeted to an OI of 15 to 25) does not decrease the incidence of ECMO or death or improve other patient outcomes has been changing with the addition of the findings from more recent studies. In most of the initial studies of iNO use, ECMO, in combination with mortality, served as a primary composite endpoint. As noted earlier, iNO significantly decreases the need for ECMO support in newborns with PPHN. However, data indicate that up to 40% of infants do not improve oxygenation or maintain a response to iNO. There are two schools of thought pertaining to this issue. One suggests that early iNO does not decrease the incidence of death or the need for ECMO or improve other patient outcomes. The other group hypothesizes that the relatively poor response is likely due to the “early” introduction of iNO to patients with prior lung damage secondary to iatrogenic hyperoxia and ventilation. An alternative explanation is that clinicians are not targeting therapies to the appropriate pathophysiological targets. In this case, gaining more relevant information from investigations using real-time cardiorespiratory monitoring (see Chapter 21 ) and cardiac ultrasound (see Chapter 10 ) could improve targeting. Few of the recent studies have addressed this question. Yet the field of precision or personalized medicine, targeting individual patient characteristics, is rapidly expanding (see Chapter 21 ).
On the basis of available evidence, a recent systematic review has concluded that it appears reasonable to use iNO at an initial concentration of 20 ppm for term and near-term infants with hypoxic respiratory failure who do not have a diaphragmatic hernia. Due to the rapid onset of action of iNO, a response, if present, can be seen quickly (within 1 hour). Evidence is lacking for the benefit of continuing iNO therapy in patients who do not respond with improved oxygenation. In selected cases, the use of noninvasive ventilation might be a good option to decrease ventilator-associated lung injury. Indeed, there have been case reports and case series describing iNO delivered through continuous positive airway pressure to newborn infants as part of a treatment strategy without adverse effects.
Role of Inhaled Nitric Oxide in Preterm Oxygenation Failure
The role of iNO in the management of hypoxic respiratory failure in preterm neonates is less straight forward. In preterm neonates, an increased pulmonary arterial pressure may be associated with pneumonia, RDS, bronchopulmonary dysplasia (BPD), premature prolonged rupture of membranes (PPROM), and oligohydramnios-associated pulmonary hypoplasia. Addressing the basic etiology is often the first step in selecting the most appropriate management strategy of preterm hypoxic failure. Furthermore, preterm neonates are at much higher risk of complications such as BPD, peri/intraventricular hemorrhage (P/IVH), and long-term neurodevelopment abnormalities. In addition to selective pulmonary vasodilatation, many studies have found that iNO might decrease lung inflammation, reduce oxidant stress, and enhance alveolarization and lung growth. Most of the trials of iNO undertaken in the preterm population have been focused on preterm hypoxic respiratory failure with ventilator dependence. In these trials, very few infants had documentation of cardiac ultrasound findings pretreatment, so the incidence of the potentially responsive pathophysiology of raised pulmonary pressures and right-to-left ductal shunt is unknown. There have been at least 16 randomized control trials (RCTs) evaluating the role of iNO in prevention of BPD. However, a Cochrane meta-analysis concluded that iNO as rescue therapy for the preterm infant does not appear to be effective. In addition, early routine use of iNO in preterm infants with respiratory disease does not affect serious brain injury or improve survival without BPD. Importantly in the systematic review, there is no clear effect of iNO on the frequency of all grades of P/IVH or of severe P/IVH, nor was there any effect on the incidence of neurodevelopmental impairment found. The initial concerns of P/IVH and poor neurodevelopmental outcome associated with iNO administration to preterm infants appear to be unfounded.
The focus in preterm infants has been on the role of iNO in BPD prevention. However, there is a subset of preterm infants who present with raised PVR that might benefit from iNO. This subset includes preterm neonates in whom the presentation of PPHN is associated with PPROM, oligohydramnios, and presumed pulmonary hypoplasia. As this is a relatively rare condition, only a few case series, small studies, and retrospective data analyses have been published. In many of these studies, a favorable response to iNO therapy in preterm infants born after PPROM and oligohydramnios has been reported. In the most recent case series, infants with a history of PPROM, pulmonary hypoplasia, and PPHN who were treated with iNO had a survival rate of 86%.
Specific cardiac ultrasound markers, such as pure right-to-left shunting at ductal level or a low left pulmonary artery blood flow velocity, may allow prediction of iNO response in the preterm infant and it is important to identify the target preterm population who may benefit from iNO treatment It seems that preterm infants with elevated PVR, decreased pulmonary blood flow (diagnosed by reduced left pulmonary artery velocity on cardiac ultrasound), and an open PDA with predominantly right-to-left flow are most likely to have a dramatic response to iNO.
Weaning Inhaled Nitric Oxide
Identifying the right time to wean iNO is crucial to reduce unnecessary exposure to a treatment with potential side effects in unresponsive infants. Identifying the optimal time to wean iNO in the clinical setting can be challenging as FiO 2 requirement and SpO 2 /pCO 2 lability can persist, even though the underlying pathophysiology has changed. Waiting for a clear clinical improvement may take several days. Once the PVR has reduced and, in particular, once the lability of an open PDA with changing proportions of right-to-left shunt has resolved, it is our experience that iNO may be weaned. Longitudinal cardiac ultrasound assessment (1 to 2 times daily) allows tracking of hemodynamic improvement and often helps identify the time to commence weaning iNO administration and, if the patient remains stable, hemodynamic support. This may be as early as 24 to 48 hours after commencing therapy.
However, rebound vasoconstriction following abrupt withdrawal of iNO is a real concern, and weaning iNO from 20 ppm gradually over a period of time before its discontinuation has been shown to be a safer approach that minimizes the chance of occurrence of the rebound effect. Of note is that, even in infants who show no response to iNO, sudden discontinuation of the gas can precipitate pulmonary vasoconstriction and rapid deterioration. Secondary analysis of a large blinded study reported that decreases in PaO 2 were observed only at the final step of withdrawal around iNO discontinuation. iNO reduction should be started when PaO 2 is above 60 mm Hg or when the OI is less than 10. A reduction to 1 ppm before discontinuation seems to minimize rebound hypoxemia. In one study it was reported that withdrawing iNO in nonresponders did not result in rebound hypoxemia when NO exposure was limited to 30 minutes. This finding supports the approach to discontinue iNO following a very short trial in nonresponders.
Other Pulmonary/Systemic Vasodilators
Administration of O 2 at appropriate concentrations, normalization of the acid-base status, and administering iNO are the mainstays of vasodilator therapy in infants with PPHN. However, about one third of cases fail to respond to iNO or only have a transient treatment effect. In addition, there are many centers, particularly in the developing world, who do not have access to iNO. Fortunately, there are a number of adjunct and/or alternate pulmonary vasodilator options available. However, their efficacy and safety have not been tested in large randomized trials comparable to those with iNO, nor have the long-term outcomes been carefully examined. Moreover, as some of these vasodilatory agents also have systemic effects, systemic hypotension might occur and can worsen right-to-left shunting and tissue O 2 delivery in some clinical settings.
Phosphodiesterase-5 Inhibitors
Endogenous NO stimulates cGMP generation in the smooth muscle cells of the pulmonary vessels, which leads to the relaxation of the smooth muscle. In addition, iNO-induced direct activation of K + channels and modulation of angiotensin II receptor expression and activity contribute to the pulmonary vasodilatory effects of iNO. cGMP is primarily metabolized by phosphodiesterase-5 (PDE-5), an enzyme also expressed in the vessels of the lungs, and its downregulation enhances NO-mediated vascular relaxation during normal pulmonary vascular transition after birth. Thus, inhibition of PDE-5 will increase cGMP concentrations in pulmonary smooth muscle cells and facilitate endogenous or iNO-induced vasodilatation in the pulmonary vasculature. In addition, PDE-5 inhibition may also result in a decrement in medial muscular hypertrophy in neonates with exposure to intra-uterine hypoxia and remodeled pulmonary vasculature. Sildenafil, vardenafil, and tadalafil belong to the class of PDE-5 inhibitors, with sildenafil being the most widely studied drug of this class in neonatal pulmonary hypertension.
Based on the available evidence, the use of sildenafil has several potential indications in neonatal intensive care. It can be used as an acute adjuvant to iNO, given in iNO-resistant PPHN, or to facilitate weaning of iNO. Sildenafil can also be used as a primary drug in the treatment of PPHN if iNO is not available or is contraindicated. Furthermore, it may be used for chronic treatment of pulmonary hypertension in conditions, such as BPD or congenital diaphragmatic hernia (CDH).
Sildenafil maybe given enterally or intravenously usually without clinically significant side effects. However, the development of systemic hypotension is a potential problem, especially if a loading dose is used and it is administered over less than 30 minutes. Administration of sildenafil in combination with iNO was also not associated with a significant decrease in systemic blood pressure and it improved oxygenation. In two recently published studies, there were also no significant adverse events.
Phosphodiesterase-3 Inhibitors
Another class of vasodilators are the phosphodiesterase-3 (PDE-3) inhibitors such as milrinone which act by inhibiting the breakdown of cyclic adenosine monophosphate (cAMP). Milrinone plays two important roles in the context of PPHN management. First, via its inotropic and lusitropic effects, it has a role in improving ventricular function. Second, by increasing the concentration of cAMP in both pulmonary arterial smooth muscle cells and cardiomyocytes, it improves ventricular diastolic function and induces further pulmonary vasodilation. PDE-3 expression and activity increase dramatically in spontaneously breathing lambs by 24 hours after birth. Accordingly, milrinone may not be as effective as a pulmonary vasodilator or an inotrope immediately after birth, but it may be increasingly useful after the first postnatal day. Ventilation with 21% O 2 or 100% O 2 attenuates the postnatal upregulation of PDE-3, while exposure to iNO plus 100% O 2 markedly increases PDE-3 expression. Therefore, milrinone may be a potentially useful adjunct treatment modality when iNO and high O 2 concentrations are used in neonates with PPHN.
Based on animal, and human pediatric, adult, and scarce neonatal data, there are several clinical situations where milrinone may particularly be of use in the management of neonatal PPHN. When perinatal asphyxia- or sepsis-associated PPHN presents with ventricular dysfunction and high pulmonary venous pressure, there may be a left-to-right shunt through the PFO instead of the expected bidirectional or right-to-left shunt characteristic of pulmonary arterial hypertension. In this situation, milrinone may have a definite, even preferred role, as iNO may worsen the hemodynamic presentation by dilating the pulmonary vasculature and thus aggravating the myocardial dysfunction-associated pulmonary venous congestion. As mentioned earlier, milrinone also may be used as an adjuvant to iNO especially in infants only partially responsive to iNO. Similarly, milrinone may have a role in iNO-unresponsive PPHN. Indeed, there have been several case series in term neonates with iNO-refractory PPHN demonstrating effectiveness of milrinone in reducing the pulmonary pressure. In addition, a retrospective case review reported improved myocardial performance and pulmonary hypertension after treatment with milrinone in less than 32 weeks’ gestation preterm neonates.
A loading dose of 50 mcg/kg over 30 to 60 minutes followed by a maintenance dose of 0.33 mcg/kg/min and escalated to 0.66 and then to 1 mcg/kg/min based on response, is commonly used. However, the loading dose is not recommended in the presence of systemic hypotension or prematurity; therefore, in some centers milrinone is used without a loading dose in all patients. There have been reports of systemic hypotension after milrinone use in the adult intensive care unit. In a recent retrospective data analysis, the authors identified 322 neonates who were exposed to milrinone in their study period of 13 years and reported 40% of the infants experienced clinical or laboratory adverse effects. Surprisingly PPHN (40%) was the most commonly reported diagnosis at the start of milrinone administration. Hypotension requiring vasopressors (20%) and PDA requiring treatment (13%) were the most commonly reported clinical adverse events during milrinone therapy.
Prostaglandins
Prostaglandin-E1 (PGE1) is usually used as a bridging therapy to keep the ductus arteriosus patent in neonates with duct-dependent congenital cardiac conditions before undertaking corrective surgical therapy. A study in near-term and term infants with PPHN found that the ductus arteriosus was patent in 72% of the infants compared to 34% in the control group without PPHN. The PDA functions as a pressure pop-off valve to release the high right ventricular pressure from raised PVR, thereby improving overall cardiac function and alleviating the need for PGE1 infusion in many infants. By augmenting endogenous production of cAMP, PGE1 also causes nonselective pulmonary vasodilatation. Although one group of investigators reported encouraging results with the use of PGE1 in two small pilot trials, PGE1 use is generally recommended in infants with PPHN with a closed duct and evidence of right ventricular dysfunction as an adjunctive treatment to O 2 and iNO and the other pulmonary vasodilators discussed earlier.
Prostacyclin (PGI2) is another endogenously produced arachidonic acid metabolite that also causes vasodilatation via the cAMP pathway. PGI2 production appears to increase in late gestation and early postnatal life, suggestive of its involvement in promoting neonatal pulmonary vascular dilatation at birth. In neonates and children with PPHN there is a decrease in the biosynthesis of PGI2 accompanied by increased synthesis of the vasoconstrictor thromboxane-A2. PGI2 vasodilates both the pulmonary and systemic vascular bed. However, PGI2 mostly induces vasodilation in the pulmonary venous system and thus its use may result in pulmonary congestion and edema formation. Intravenous PGI2 is one of the more commonly used therapies in adult pulmonary hypertension management. However, there have been only case series and case reports pertaining to newborn intravenous PGI2 use. Although it might be beneficial in the management of PPHN, systemic hypotension has been reported in almost all the case series and reports, significantly limiting its use in acute pulmonary hypertension. Investigators have used PGI2 by inhalation with some success both in term and preterm neonates. Because of its very short half-life (∼6 minutes), epoprostenol must be administered continuously and unplanned interruptions in drug delivery may rapidly lead to rebound pulmonary hypertension. Inhaled Iloprost, a stable PGI2 analogue, has been used successfully in preterm and term infants with both idiopathic PPHN and BPD- and CDH-associated PPHN without major side effects. However, the studies are mainly case series or case reports and thus the findings must be viewed with caution.
Endothelin Receptor Antagonists
Endothelin-1 (ET-1), synthesized by the vascular endothelial cells, is a potent vasoconstrictor and acts through two receptors (ETA and ETB). ETA primarily mediates vasoconstriction while ETB promotes vasodilation mediated by endothelium-derived NO. Elevated plasma ET-1 levels are observed in infants with CDH and PPHN, and they have been used as a marker of disease severity and poor prognosis. Endothelin receptor antagonists are categorized according to their selectivity for ETA or ETB receptors. Bosentan is a nonselective ET receptor antagonist and has been principally used to treat pulmonary hypertension in adults. There have also been case reports and case series on the use of bosentan in the management of pediatric pulmonary hypertension. Use of bosentan as a stand-alone therapy or an adjuvant to iNO and sildenafil in term and preterm neonates with pulmonary hypertension has also been reported. In an RCT in term and near-term neonates with PPHN and an OI of greater than 40, bosentan, at an enteral dose of 1 mg/kg twice a day induced significant improvement in the OI and SpO 2 in the majority (87.5%) of the neonates within 6 hours after initiation of treatment. There was no rebound hypoxemia after drug discontinuation, and the duration of mechanical ventilation was decreased. At the 6-month follow-up evaluation, major sequelae, including neurological sequelae, were significantly lower in infants treated with bosentan compared to the controls. However, in another recent, although smaller RCT of oral bosentan given at a dose of 2 mg/kg twice daily as an adjunctive therapy to iNO in neonates with PPHN and respiratory failure, there were no clinical benefits. Thus, due to the limited neonatal data, routine use of bosentan cannot be recommended, although the drug may have a role in the management of PPHN not responding to conventional vasodilators, optimized ventilation, and supportive therapy.
Magnesium Sulfate
Magnesium sulfate (MgSO 4 ) is a nonselective vasodilator and acts by antagonizing the entry of calcium ions into the smooth muscle cells. Beside its vasodilatory properties, magnesium has other effects including sedation, muscle relaxation, bronchodilation, and neuroprotection, which might make it potentially useful in certain presentations of PPHN.
The role of MgSO 4 in newborn PPHN has never been tested in an RCT trial. There have been small case studies in term and preterm neonates with some positive results In the absence of safety data and RCTs, MgSO 4 should be a last line of therapy when other effective pulmonary vasodilator-treatment and ECMO support are not available.
Utilizing Pathophysiology to Guide the Use of Vasodilators in Persistent Pulmonary Hypertension of the Newborn
As discussed, there are several vasodilators available in addition to O 2 and iNO. The key advantage of iNO is its ability to be delivered via gaseous inhalation, thus allowing optimization of VQ matching by primarily delivering the vasodilatory effect to aerated lung fields. As iNO is rapidly deactivated by hemoglobin in the blood, there is little systemic vasodilation with an associated drop in systemic blood pressure. As for the other vasodilators, the actual clinical and hemodynamic scenario can serve as guidance when deciding what medication(s) and when to use. For instance, without appreciation of the underlying pathophysiology, commencement of a nonspecific vasodilator may result in the development of systemic hypotension and worsening hypoxemia. Furthermore, in infants presenting with a wide-open ductus arteriosus and predominant right-to-left shunting, good right ventricular function, and indirect evidence of relative systemic vasoconstriction, perfusion and oxygenation may worsen when a medication with a systemic vasodilatory effect, such as milrinone, systemic PGI2, or MgSO 4 , is added. On the other hand, infants with a small and/or constricting ductus arteriosus, predominantly left-to-right ductal shunting and an “obstructed” right ventricle with reduced right ventricular function may benefit from a drug with both inotropic effect and vasodilatory effect, such as milrinone. Indeed, with this latter hemodynamic presentation, the infant is less likely to develop worsening oxygenation if systemic blood pressure decreases (up to a certain point) with the introduction of a vasodilator/lusitropic agent.
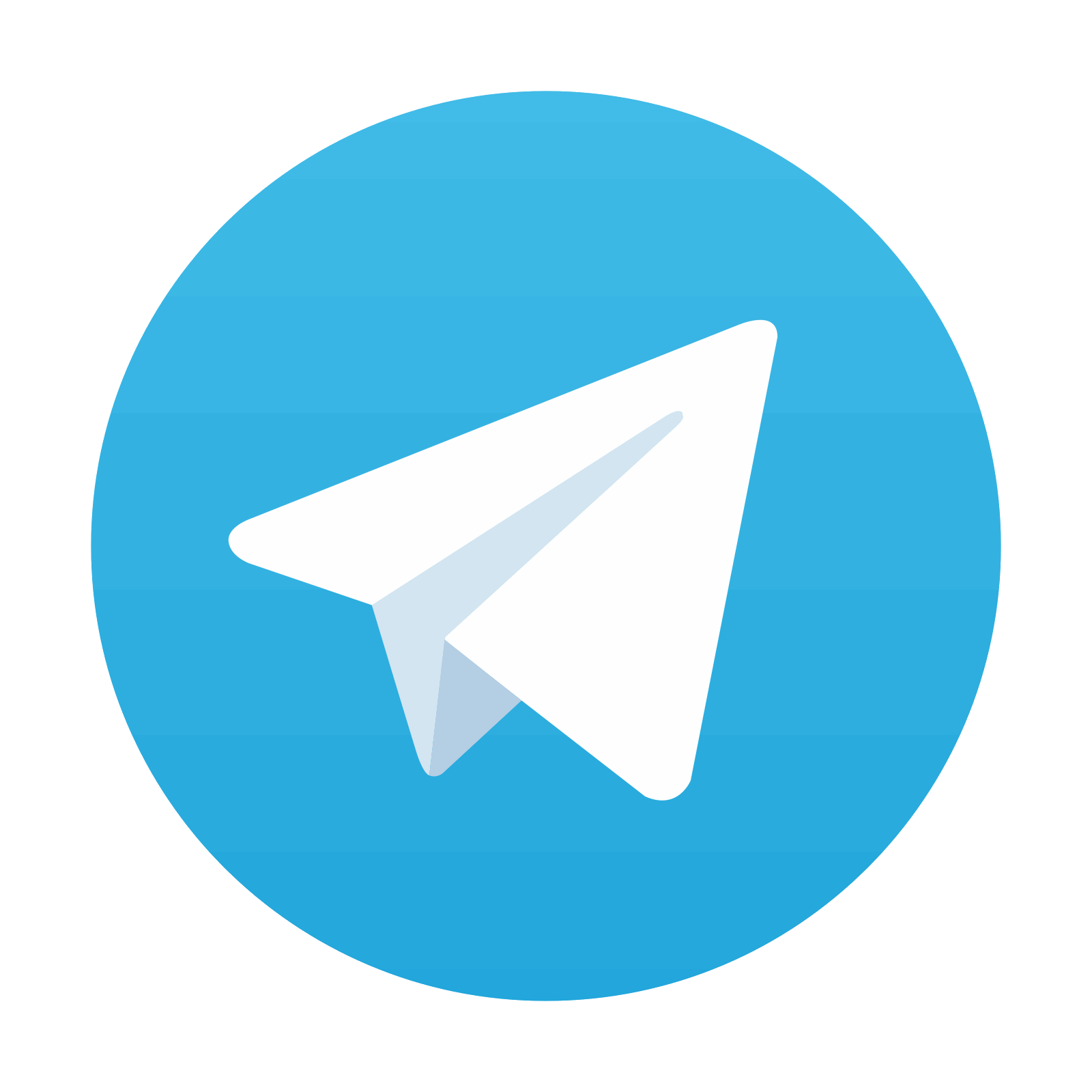
Stay updated, free articles. Join our Telegram channel

Full access? Get Clinical Tree
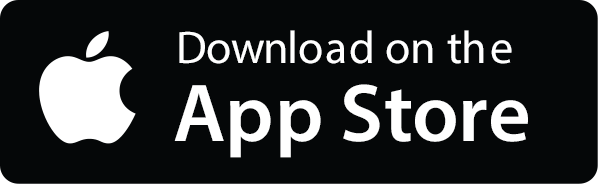
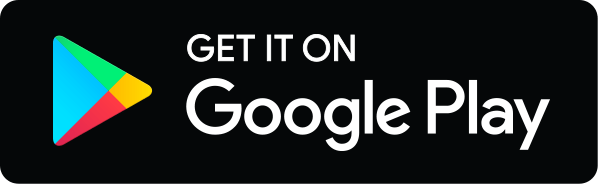