Vascular lesions that resemble atherosclerosis have been described in Egyptian and Renaissance mummies, , but death and disabling symptoms caused by atherosclerosis were probably rare before the late 18th century and remained relatively uncommon until the beginning of the 20th century. From this time on, the incidence of symptomatic atherosclerosis increased dramatically in Europe and the United States until the mid-1900s, and increases of comparable magnitude occurred later or are now occurring in many other parts of the world, making atherosclerosis a leading global cause of death and disability today.
Pathoanatomic descriptions of the disease span the last 250 years. In 1786, Edward Jenner proposed coronary atherosclerosis to be the cause of angina pectoris—the disease described few years before by Heberden—and, by the mid-1800s, the histology of atherosclerosis was already described in detail by Virchow and others. Angina pectoris was recognized to be a deadly disease, but the most common link between atherosclerosis and death (i.e., myocardial infarction [MI] caused by coronary thrombosis) was not described until the accounts of Weigert and Osler in 1880 and 1910, respectively. It would take an additional 50 years before Constantinides, Chapman, and Friedman discovered the mechanism underlying most cases of coronary thrombosis. They found that most coronary thrombi are precipitated by atherosclerotic plaque rupture, whereby highly thrombogenic plaque components are exposed to the flowing blood. It was also noted that some thrombi formed on nonruptured plaques with minimal surface irregularities, and later the term plaque erosion was introduced to describe this phenomenon. Today, we know that not only MI, but also unstable angina and most cases of sudden coronary death, are caused by thrombosis superimposed on ruptured or eroded plaques. This shared pathogenesis is reflected in the collective clinical term acute coronary syndrome (ACS).
In this chapter, we will review the pathogenesis of atherosclerosis, its early onset in life and variable progression rate, the heterogeneity of atherosclerotic plaques, and how the heterogenous nature of the disease translates into different clinical presentations.
Classification and Progression of Atherosclerosis
Atherosclerosis is a chronic, inflammatory, fibroproliferative disease of the intima of large and medium-sized arteries characterized by early retention and modification of atherogenic lipoproteins, recruitment of monocytes and T lymphocytes, and subsequent accumulation of abundant fibrous tissue. The causal agent is an elevated level of apolipoprotein B (apo B)–containing lipoproteins in the blood, but other risk factors such as male gender, hypertension, diabetes, and genetic susceptibility modify the disease by only partly understood mechanisms.
Classification
Atherosclerosis begins to develop early in life, but the speed of progression is unpredictable and varies markedly among different subjects. However, even in those most susceptible to the disease, it usually takes several decades to develop obstructive or thrombosis-prone plaques. In a series of key papers, the Committee on Vascular Lesions of the Council on Atherosclerosis, American Heart Association (AHA), reviewed how the pathogenesis of atherosclerosis can be inferred from histologic analyses of lesions at defined arterial locations in persons from childhood to old age. The AHA council also proposed a widely used classification scheme for atherosclerotic lesion types, which was updated in 2000. An alternative classification, which emphasizes the link between atherosclerotic plaque morphology and clinical presentations, was suggested by Virmani and colleagues.
Figure 6-1 displays a simplified version of the AHA classification and the proposed sequence of lesion progression. Atherosclerosis is a heterogeneous disease and a single patient with advanced disease will typically harbor all these different lesion types at different sites of the coronary tree ( Fig. 6-2 ). However, also within very short artery segments, several lesion types may be identified in close proximity by serial sectioning. The latter type of variation stems from the fact that current classifications of atherosclerosis assign lesion types based on thin cross sections (a few microns) of a three-dimensional structure that vary considerable in morphology in the longitudinal direction.


Normal Arterial Intima
The arterial wall is composed of three layers—the tunica intima (inner layer), the tunica media (middle layer), and the tunica adventitia (outer layer). The intima is defined as the region that extends from the arterial lumen, including the endothelium, to the internal elastic lamina. It is often no more than a few cell layers thick, but in discrete, reproducible regions of the normal arterial tree, the intima is thickened and consists of a subendothelial proteoglycan-rich layer and a deeper musculoelastic layer with smooth muscle cells (SMCs) and elastic fibers. These intimal thickenings presumably form as a physiologic adaptation to low and/or oscillatory wall shear stress. They are mainly located near vessel branch points or along inner vessel curvatures. , In humans and animals without atherosclerosis, these sites are further characterized by changes in the shape and replication rate of endothelial cells, expression of adhesion molecules, and recruitment of dendritic cells from circulating monocytes. ,
Intimal thickenings are affected early during atherogenesis; they are atherosclerosis-prone , and the rate of progression is higher here than at other arterial sites. With age, adjacent intima is increasingly involved, so that most of the epicardial coronary arteries are affected in persons dying from an ACS in older age.
Progression of Atherosclerosis
Foam Cell Lesions
The key initiating mechanism in atherosclerosis appears to be the retention of apo B-containing lipoproteins, primarily low-density lipoprotein (LDL), to extracellular proteoglycans in the arterial intima. , Extracellular lipid droplets of aggregated and fused LDL particles in the proteoglycan-rich layer of intimal thickenings represent the first microscopic sign of atherosclerosis in children and young adults. ,
Subendothelial modification of the retained lipoproteins (e.g., aggregation, fusion, oxidation) by enzymes and oxidative radicals yields a range of bioactive lipids. The modified lipoprotein moieties, in turn, act as proinflammatory mediators that stimulate the recruitment, differentiation, and replication of monocyte-derived macrophages through induction of endothelial adhesion molecules, chemoattractants, and growth factors. , In the intima, macrophages avidly engulf modified, but not native, LDL particles through scavenger receptors, and their cytoplasm becomes packed with droplets of cholesteryl esters, giving them the appearance of foam cells. T lymphocytes and dendritic cells are also present from this stage onward, and immune responses are an important modulator of the atherosclerotic process.
Foam cells accumulate in the luminal, proteoglycan-rich layer of the intima and, when several layers of foam cells have formed, they may be visible to the naked eye on the intimal surface of arteries as yellow fatty streaks . Foam cell lesions are harmless and are fully reversible if the local pathologic stimuli that caused their formation dissipate. For instance, they are present in the coronary arteries in 50% of infants during the first 6 months of life, but their number declines in subsequent years. At puberty, they usually reappear in atherosclerosis-prone regions of the arterial tree, but only some of these progress to more advanced stages of atherosclerosis.
Intermediate Lesions
In some foam cell lesions, retention of lipoproteins from the circulation continues and is enhanced, and isolated extracellular lipid pools begin to accumulate in the musculoelastic layer beneath the layers of foam cells. There is dispersion or local loss of the intimal SMCs where the pools form, but there is no gross disruption of the normal structure of the intima. , Recent studies have suggested that the formation of lipid pools is accentuated by failure of the normal phagocytic clearance of apoptotic foam cells.
Foam cell lesions with basal pools of extracellular lipid are termed intermediate lesions , sometimes referred to as pathologic intimal thickening, because they represent the historically controversial link between the innocuous and widespread fatty streaks, and the clinically relevant, more localized advanced lesions. Intermediate lesions are evident by 20 to 30 years of age in atherosclerosis-prone regions of the coronary arteries. ,
Fibroatheromas
The critical step that turns the reversible precursor lesions (foam cells and intermediate lesions) into irreversible advanced (fibro)atheromas is the conversion of the isolated lipid pools into one or more confluent lipid-rich cores (or, synonymously, necrotic core, lipid core, or atheromatous core). This process irreversibly disrupts the normal structure of the intima with degradation of the extracellular matrix and death of local SMCs and leaves behind a matrix-devoid, acellular gruel of lipids (cholesteryl esters, free cholesterol, phospholipids, triglycerides) and cell debris.
Foam cell apoptosis combined with defective phagocytic clearance of apoptotic cells contribute to the formation and growth of the lipid-rich core. Cell death, both apoptotic and other forms, occurs at the margin of the core, and macrophage-specific antigens and apoptotic microparticles are present within the atheromatous material. , Lipoproteins may also insudate directly from the blood into the core without passing foam cells, and this may actually be the quantitatively dominant source of the lipids. In more advanced plaques, intraplaque hemorrhage into the core from neovessels may be an important source of lipids, especially of free cholesterol. Neovascularization extending from the adventitial vasa vasorum into the base and shoulder regions of the plaque is a common feature at the fibroatheroma stage.
The piece of tissue that separates the lipid-rich core from the blood is called the fibrous cap . This structure plays an immensely important role for the clinical consequences of atherosclerosis as detailed below. The fibrous cap initially consists of the proteoglycan-rich layer of the normal intima (with isolated intimal SMCs and infiltrating foam cells), now separated from the underlying media by the developing lipid-rich core. Gradually, however, the original intimal tissue is replaced and expanded by collagen-rich fibrotic tissue, which eventually grows to become the quantitatively dominant component in most advanced plaques. The AHA classification reserves the term fibroatheroma for lesions with fibrosis and uses the term atheroma for lesions with only normal intimal connective tissues ; others use the term fibroatheroma collectively for both lesion types.
The fibrous matrix of atherosclerotic plaques is produced by synthetic-type SMCs which, compared with the normal contractile SMCs of the arterial media, are ultrastructurally dominated by organelles involved in protein synthesis (rough endoplasmic reticulum and Golgi apparatus) and show increased capacities for proliferation, migration, and matrix synthesis. A few synthetic-type SMCs are present in the normal intima, but they increase substantially in number at the fibroatheroma stage. A novel hypothesis has implicated circulating, bone marrow–derived progenitor cells in the recruitment of these cells to the atherosclerotic plaque, , but recent experimental studies in mouse models have shown that only proliferation and migration of local cells, presumably local contractile SMCs, are contributing factors. Indeed, contractile SMCs in the intima and media are able to modulate to the matrix-producing synthetic phenotype, and this phenotypic modulation appears to be key in the formation of the fibrous component of atherosclerosis. In recent years, the molecular switches that control SMC phenotype have begun to be unravelled, but the extracellular cues that direct the process are incompletely understood.
A third characteristic component of fibroatheromas is calcifications. Microscopic calcium granules are found intracellularly in some SMC organelles and extracellularly throughout the lipid-rich core. These initial microscopic foci of calcification may expand to form larger lumps and plates of calcium deposits, especially at the base of lipid-rich core. This may be a regulated process reminiscent of bone formation facilitated by phenotypically modulated, osteogenic SMCs, or by other cell types in the plaque. , Calcifications can constitute most of the plaque volume.
Fibrous Plaques
The fibroatheroma is the hallmark of atherosclerosis and what named the disease. Athére is Greek for gruel or porridge and refers to the lipid-rich core, and scleros means hard, which describes the fibrotic and often calcified encapsulating tissue. However, many advanced plaques at autopsy are not fibroatheromas but are fibrous plaques consisting of relatively homogeneous fibrotic tissue with calcifications, but no lipid-rich core. Their genesis is not fully understood. Some pathologists believe that the development of a lipid-rich core is the prerequisite of fibrosis, and serial sectioning often reveals that a lipid-rich core is present in the upstream or downstream vicinity of the section with fibrous plaque. If this is not the case, an originally formed lipid-rich core may have disappeared because of local quiescence of the atherosclerotic process or through plaque rupture with core extrusion and subsequent healing (see later). Regression studies in monkeys have indicated that lipid-lowering can turn fibroatheromas into fibrous plaques. On the other hand, recent studies have shown that some fibrous plaques may have a separate genesis, developing in the absence of a preceding lipid-rich core and fatty streak.
Complicated Plaques
When thrombus is present, with or without luminal obstruction, the lesion is called a complicated plaque and is further subdivided by the presence or absence of plaque rupture. With rupture (also known as plaque fissuring), the thrombus is in direct connection with the highly thrombogenic lipidrich core of a fibroatheroma through a disruption of the fibrous cap ( Fig. 6-3 ). When no such rupture can be identified despite a thorough search, the term plaque erosion is used. Both intermediate lesions, fibroatheromas, and fibrous plaques may be complicated by plaque erosion. Plaque disruption is sometimes used synonymously with plaque rupture and sometimes as a collective term for rupture and erosion. Some pathologists acknowledge a third, but rare, category of complicated plaques, calcified nodule.

Plaques Causing Stable Angina
Over the years, plaques can grow to become so voluminous that the arterial lumen is reduced below a critical point and ischemia sets in, leading to stable angina pectoris. Most advanced plaques in humans, however, will never cause symptoms. This is partly because stenosis is not flow-limiting until geometrically severe and partly because the artery often dilates during atherogenesis.
Determinants of Stenosis Severity
The severity of flow obstruction in atherosclerotic arteries depends on plaque size, vasoconstriction (spasm), and remodeling of the vessel wall. During atherosclerotic lesion formation, the local vessel segment may expand and thereby preserve the lumen (expansive remodeling, also known as positive or outward remodeling), or shrink to diminish it (constrictive remodeling, also known as negative or inward remodeling; Fig. 6-4 ). Expansive remodeling is more common than constrictive remodeling. ,

Autopsy studies and intravascular ultrasound (IVUS) examination of coronary arteries have shown that the extent and direction of arterial remodeling are at least as important as plaque size in determining stenosis severity. Therefore, imaging of the lumen of an artery by coronary angiography or other techniques is not useful for diagnosing the presence of atherosclerotic plaque or measuring changes in atherosclerotic plaque size with medical intervention.
The mechanisms of arterial remodeling in atherosclerosis have not been fully clarified. Expansive remodeling may partly be a homeostatic response of the nondiseased vessel wall at sites of eccentric plaque formation to maintain normal shear stress. However, increasing evidence has suggested that it is predominantly a pathophysiologic process, in which proteolytic enzymes secreted by plaque macrophages cause the underlying media to thin and yield. This is supported by the fact that plaque growth is frequently followed by a paradoxical increase in luminal area in mice and humans, , local expansion occurs beneath atherosclerotic plaques in mice, and the direction and extent of remodeling are associated with the composition of the local plaque. , Initial autopsy studies have indicated that expansive remodeling is only possible until the atherosclerotic plaque constitutes approximately 40% of the area within the internal elastic lamina, but this may have been an artifact of the variable used to measure plaque size. A recent IVUS study has shown that the capacity of the vessel wall to remodel is unrelated to plaque burden. The mechanism of constrictive remodeling have not been studied in detail, although it may be related to plaque rupture followed by healing (see later).
Superimposed on arterial remodeling, local vasoconstriction may have a profound effect on flow obstruction, and paradoxical exercise-induced vasospasm is frequent in stable angina pectoris. These dynamic changes should be distinguished from structural remodeling of the artery.
Development of Stenoses
Resting blood flow through a stenosed artery is not significantly affected until the luminal area is reduced by approximately 80% (or the diameter by 50%), although the flow reserve is decreased with less reduction. Stenoses of this magnitude are caused by a fibroatheroma or fibrous plaque. The plaque may be substantially calcified and the local vessel segment is often negatively remodeled, but the relationship among plaque morphology, arterial remodeling, and stenosis formation is not consistent.
A small proportion of culprit plaques in stable angina have residual thrombus and at postmortem examination there may be evidence of previous occlusive thrombosis in the form of a multichannel lumen, suggesting recanalization, or myocardial scarring in the region supplied by the artery. Furthermore, a special nonuniform pattern of dense type I (older) and loosely arranged type III (younger) collagen, considered to indicate healed plaque rupture, has been identified in many coronary plaques, particularly in those that cause chronic high-grade stenoses. Often, several healed rupture sites are present in plaques and the number of healed ruptures correlates with the severity of stenosis.
The combined findings indicate that subclinical plaque ruptures with thrombus formation may be important in chronic stenosis formation. Indeed, the vast majority of plaque ruptures are not lethal and most are clinically silent. In these cases, SMCs heal the rupture site and organize residual thrombus by secreting an extracellular matrix rich in glycosaminoglycans and type III collagen. This restores the integrity of the plaque surface but may cause rapid plaque growth, , and scar-like contraction of the healing SMCs pulling on fibrin fibrils may lead to concomitant constrictive remodeling. ,
The central role of plaque healing in stenosis formation is consistent with clinical observations. Serial angiography has shown that many coronary artery stenoses develop in a phasic rather than linear manner, with chronic high-grade stenoses forming at sites that were only insignificantly narrowed the year before.
Plaques Causing Acute Coronary Syndromes
Unstable angina and acute MI are almost always caused by a luminal thrombus superimposed on an atherosclerotic plaque with or without concomitant vasospasm. In ST-segment elevation myocardial infarction (STEMI), the thrombus is usually occlusive and sustained, whereas in unstable angina and non-STEMI (NSTEMI), the thrombus is more often nonocclusive and dynamic. Acute or organized thrombus is found in most victims of sudden coronary death; the rest die with severe coronary disease and myocardial scarring (old MI) in the absence of thrombosis. , Rare causes of ACS include emboli, artery dissection, vasculitis, cocaine abuse, and trauma.
The most frequent cause of coronary thrombi is plaque rupture. In plaque rupture, a structural defect in the fibrous cap exposes the highly thrombogenic core to the blood (see Fig. 6-3 ). By definition, when no plaque rupture is identified, despite a thorough search, the term plaque erosion is used. Table 6-1 presents an overview of autopsy studies in which fatal coronary thrombi were identified and the underlying plaques studied carefully to detect plaque rupture. This worldwide survey shows that plaque rupture is the major cause of coronary thrombosis, responsible for approximately 75% of cases. Plaque rupture is a less frequent cause of thrombosis in women (60%) than in men (78%), and is rare in a very small subgroup of patients—namely, premenopausal women, who constitute less than 1% of heart attack victims. A few studies have reported that diabetes, smoking, and the level of hyperlipidemia are associated with the mechanism of thrombosis in ACS but, except for gender and menopause, no consistent relationships have been demonstrated.
Study (Year) | Cause of Death | Gender | Thrombi, No. of Cases | Rupture, No. of Cases (% of Total) |
---|---|---|---|---|
Chapman (1965) | — | — | 19 | 19 (100) |
Constantinides (1966) | — | — | 17 | 17 (100) |
Friedman et al (1966) | AMI + SCD | — | 40 | 39 (98) |
Bouch & Montgomery (1970) | AMI | Female | 32 | 26 (81) |
Male | 56 | 45 (80) | ||
Sinapius (1972) | AMI | — | 91 | 68 (75) |
Horie et al (1978) | AMI | — | 76 | 69 (91) |
Falk et al (1983) | AMI | Female | 12 | 8 (67) |
Male | 37 | 32 (86) | ||
Tracy et al (1985) | SCD | — | 32 | 26 (81) |
El Fawal et al (1987) | SCD | — | 61 | 39 (64) |
Yutani et al (1987) | AMI | Male | 83 | 52 (63) |
Richardson et al (1989) | — | — | 85 | 71 (84) |
van der Wal et al (1994) | AMI | — | 20 | 12 (60) |
Shi et al (1999) | AMI | — | 61 | 56 (92) |
Arbustini et al (1999) | AMI | Female | 107 | 67 (63) |
Male | 184 | 150 (82) | ||
Kojima et al (2000) | AMI | Female | 26 | 18 (69) |
Male | 74 | 63 (85) | ||
Farb et al (1996) | SCD | Female | 16 | 5 (31) |
Male | 34 | 23 (68) | ||
Davies (1997) | SCD | Female | 27 | 16 (59) |
Male | 134 | 113 (84) | ||
— | 41 | 14 (34) | ||
Burke et al (1999) | SCD | Male | 70 | 44 (63) |
Burke et al (1998) | SCD | Female, <50 yr | 16 | 1 (6) |
Female, ≥50 yr | 10 | 7 (70) | ||
All | AMI + SCD | Both | 1461 | 1100 (75) |
Males | 672 | 522 (78) | ||
Females | 246 | 148 (60) |
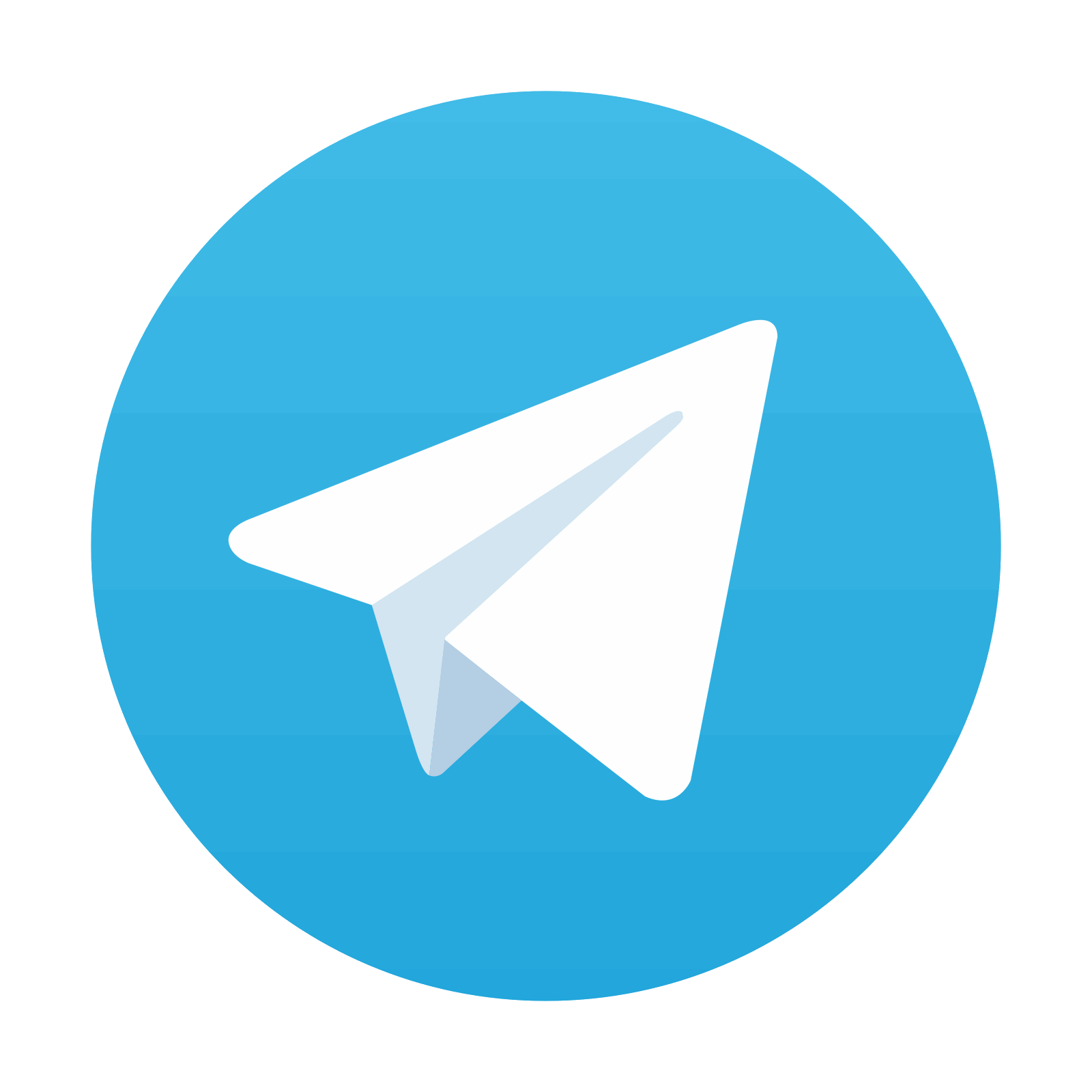
Stay updated, free articles. Join our Telegram channel

Full access? Get Clinical Tree
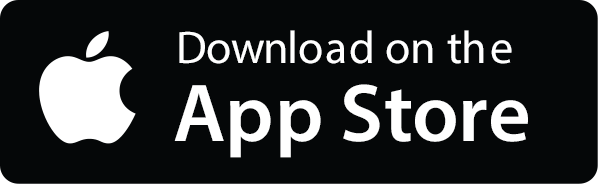
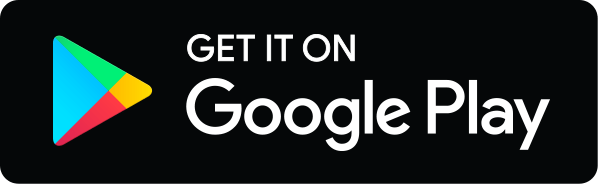
