Acknowledgments
The authors’ research was supported by grants from the National Heart, Lung and Blood Institute (PO1 HL51971), National Institute of General Medical Sciences (P20 GM104357), National Institute of Diabetes and Digestive and Kidney Diseases (1K08DK099415-01A1), and the American Heart Association. We thank Stephanie Lucas for expert assistance in preparing this chapter.
Primary (essential) hypertension accounts for the vast majority (>90%) of human hypertension and involves complex interactions of multiple organ systems and neurohormonal controllers of blood pressure (BP) as well as local tissue control systems. Although primary hypertension is a heterogeneous disorder, overweight and obesity account for as much as 65% to 75% of the risk for increased BP in these patients. Genetics and other factors such as increased dietary sodium intake, sedentary lifestyle, and excess alcohol consumption may also contribute to primary hypertension. Activation of neurohormonal systems such as the sympathetic nervous system (SNS) and renin-angiotensin-aldosterone system (RAAS) also play an important role in the pathogenesis of hypertension. Many of these contributors to primary hypertension ultimately cause kidney dysfunction which initiates or sustains increased BP.
In this chapter we discuss short-term and long-term BP control systems and how multiple organ systems interact to maintain tissue blood flow, salt and water balance, and overall homeostasis. We also briefly review various factors that influence activities of the SNS and RAAS, endothelial function, and oxidative stress that ultimately influence BP through effects on cardiac output (CO), vascular resistance, and renal salt and water excretion.
Control of Blood Pressure, Blood Flow, and Cardiac Output
Effective circulatory regulation involves complex interactions of neurohormonal and local control systems that regulate BP and tissue blood flow. According to the well-known formula, BP is the product of CO and total peripheral vascular resistance (TPR): mean arterial BP = CO × TPR. This equation directs attention to factors that affect cardiac and vascular function and is adequate for describing short-term BP control. However, chronic BP regulation is more complex and involves additional systems that regulate circulatory volume in relation to vascular capacitance (sometimes called “effective blood volume”); these systems may adjust BP to satisfy other critical homeostatic needs, such as the requirement to maintain balance between intake and output of salt and water.
CO represents the total blood flow of the circulation and is often described as the product of stroke volume and heart rate. Stroke volume, in turn, is determined by cardiac pumping ability and by peripheral circulatory factors that influence venous return to the heart. The heart normally pumps the amount of blood returned to it (ie, venous return) which is the sum of all blood flows returning from the tissues. Venous return and CO are therefore equal, except for momentary differences, and are determined by multiple factors that influence tissue blood flow, especially the metabolic demands of the tissues. For example, normal growth of the tissues is associated with increases in CO whereas loss of tissue mass (eg, loss of muscle mass that may occur with aging or with amputation of a limb) leads to decreases in CO.
Even without changes in tissue mass, metabolic rate greatly influences tissue blood flow and therefore CO. When metabolic rate increases during exercise or hyperthyroidism, for example, tissue blood flow and CO also increase to meet the higher metabolic needs of the tissues. In most cases, average daily CO remains relatively constant unless tissue mass or metabolism is altered. Even with conditions that cause marked sodium retention and increased blood volume, such as primary aldosteronism and sodium loading in subjects with impaired kidney function, CO remains relatively constant after initial transient changes that generally last only a few days. This is because most tissues autoregulate their blood flow over a wide range of BP according to their specific needs. Thus, although many patients with chronic hypertension have increased TPR, blood flows in most tissues are maintained at relatively normal levels as a result of local autoregulatory mechanisms. For example, increased BP and vascular stretch activates myogenic vasoconstriction, a response that can be observed even in isolated blood vessels. Also, when blood flow increases above that required to meet metabolic requirements, local vasoconstrictor mechanisms are activated in most tissues. With chronic increases in BP, there are structural changes in the blood vessels, such as thickening of vessel walls and decreased numbers of capillaries (rarefaction), which ensure relatively normal tissue blood flow despite increased perfusion pressure. Therefore, TPR often changes in parallel with BP, helping to maintain normal tissue blood flow and attenuating changes in vascular stretch.
In some instances, increased TPR and elevated BP are associated with high levels of vasoconstrictors, such as angiotensin II (Ang II) or endothelin, although it has been challenging to identify abnormally elevated levels of specific vasoconstrictors in most patients with primary hypertension. Even in cases where high levels of vasoconstrictors are found, tissue perfusion is usually maintained at a level that is appropriate for the metabolic requirements. However, blood flow “reserve” and the ability to increase tissue blood flow in response to increased metabolic requirements (eg, during exercise) may be impaired in some hypertensive subjects.
Although adequate BP is obviously required to maintain blood flow and nutrient supply to the tissues, blood flow and CO can be regulated independently of perfusion pressure when BP is elevated above normal. Moreover, BP is regulated by factors that may not be directly related to blood flow to most peripheral tissues, except for the kidneys which require a BP that maintains urinary excretion of water and electrolytes at a level equal to intake. As discussed later, chronic hypertension may represent a “trade off” that permits the kidneys to excrete normal amounts of salt and water, equal to intake, in the face of disturbances that impair renal function. For example, constriction of the renal arteries or aortic coarctation above the renal arteries initiates compensatory increases in systemic arterial pressure that eventually restore renal perfusion and excretion of salt and water to normal levels. In contrast, reducing perfusion pressure to nonrenal tissues such as skeletal muscle (eg, by aortic coarctation below the renal arteries) does not lead to chronic hypertension. Although insufficient blood flow to the brain may initiate emergency mechanisms (eg, sympathetic activation) that acutely raise BP, the importance of cerebral perfusion in long-term BP regulation is still unclear.
Long-Term Blood Pressure Control: Role of Renal Pressure Natriuresis
In contrast to the mechanisms that rapidly adjust vascular and cardiac function for moment-to-moment BP regulation, long-term regulation of average daily BP is closely linked with salt and water homeostasis. Fig. 5.1 shows a conceptual framework for integrating chronic control of BP and body fluid volumes. A key element of this feedback system is the effect of increased BP to raise renal sodium/water excretion, often called renal-pressure natriuresis/diuresis. Even temporary imbalances between intake and output will change extracellular fluid volume (ECFV) and potentially BP if cardiac and vascular functions are adequate. In some cases, increased BP serves to maintain salt and water balance, via pressure natriuresis, in the face of abnormalities that tend to cause salt/water retention. Although temporary imbalances between intake and output of salt and water occur routinely in daily life and excess sodium can be stored in tissues such as skin, independent of volume retention, balance between average intake and output must eventually be achieved; otherwise, continued expansion or contraction of body fluids would occur and ultimately lead to circulatory failure.

The kidneys have powerful intrarenal and neurohormonal systems that help maintain salt and water balance, often with minimal changes in extracellular fluid volume or BP over a wide range of salt and water intakes. For example, when salt intake is increased in persons who have normal kidney and neurohormonal functions, minimal changes in BP occur and these individuals are called “salt-resistant.” However, in “salt-sensitive” individuals with impaired kidney function, because of abnormal neurohormonal control or because of intrinsic kidney abnormalities (eg, kidney injury), increased BP and subsequent pressure natriuresis/diuresis provide another means of maintaining salt/water balance. In some circumstances, renal-pressure natriuresis may play a critical role in maintaining balance between intake and output of salt and water and in preventing excessive fluid retention.
Some investigators have argued that pressure natriuresis has minimal role in long-term BP regulation because the kidneys can adapt to elevated BP. However, considerable evidence indicates that renal perfusion pressure has a sustained effect on salt and water excretion and plays a critical role in chronic BP regulation. For example, using a split-bladder preparation to collect urine separately from each kidney and servo-controlling renal perfusion pressure in each of the two kidneys independently, we found that small changes in BP cause large alterations in NaCl/water excretion that persisted as long as pressure was altered (12 days) ( Fig. 5.2 ). We also tested the importance of pressure natriuresis in maintaining NaCl/volume balance in several forms of experimental hypertension, including Ang II, aldosterone, deoxycorticosterone acetate (DOC)-salt, norepinephrine, adrenocorticotropic hormone plus norepinephrine, and vasopressin hypertension. In each case, increases in renal perfusion pressure played a key role in maintaining salt and water balance; when renal artery pressure was servo-controlled at the normal level during development of hypertension, there was progressive sodium/water retention as well as continued increases in ECFV and systemic arterial pressure. In some cases, extreme salt/volume retention occurred when pressure natriuresis was prevented during development of hypertension, leading to circulatory congestion and pulmonary edema in a few days.

An important implication of the fact that renal perfusion pressure has a long-term effect on salt and water excretion is that chronic hypertension cannot be sustained unless pressure natriuresis is shifted to a higher BP. If pressure natriuresis was not reset, the elevated BP would increase sodium excretion and decrease ECFV and CO until BP returned to normal levels ( Fig. 5.3 ). Thus, chronic hypertension cannot be sustained by nonrenal vasoconstriction or increased CO unless there is also resetting of renal-pressure natriuresis.

Renal-pressure natriuresis is affected by many neurohormonal systems that can augment or blunt the effects of BP on salt and water balance. As discussed previously, high salt intake normally causes reductions in antinatriuretic hormones (Ang II and aldosterone) and increased formation of natriuretic hormones which together enhance the effects of pressure natriuresis, allowing the kidneys to maintain sodium balance with minimal changes in BP. However, excessive activation of antinatriuretic systems (eg, RAAS or SNS) reduces the effectiveness of pressure natriuresis, requiring a higher BP to maintain sodium balance.
In all forms of experimental or human hypertension studied thus far, there is a shift of pressure natriuresis to higher BP. This shift may be because of neurohormonal or intrarenal disorders that either reduce glomerular filtration rate (GFR) or increase renal tubular salt or water reabsorption. The increased BP, in turn, helps to return salt and water excretion to normal, via pressure natriuresis, despite kidney dysfunction.
Sodium Retention Does Not Always Cause Hypertension
Although impaired pressure natriuresis is required for chronic hypertension to be sustained, sodium retention does not always elevate BP. In pathophysiologic conditions such as chronic heart failure and cirrhosis, salt and water retention occur without hypertension. In these cases, salt and water retention occurs as a compensation for inadequate cardiac or vascular functions which tend to reduce BP. In heart failure, salt and water retention tend to increase ECFV which may raise cardiac filling pressures sufficiently to return CO and BP toward normal if cardiac dysfunction is not too severe. In cirrhosis there is loss of fluid from the circulation into the interstitial spaces and/or increased vascular capacitance and pooling of blood in the portal circulation because of liver fibrosis. This also leads to activation of various antinatriuretic systems which cause salt and water retention, helping to sustain normal BP.
Impaired Renal-Pressure Natriuresis Does Not Always Cause Sodium Retention or Increases in Blood Volume and Cardiac Output
Although ECFV and blood volume are key components of long-term BP regulation, via the renal-body fluid feedback mechanism, BP is not a function of blood volume per se but of blood volume in relation to vascular capacity. This concept is sometimes referred to as “effective blood volume.” When vascular capacity increases (eg, large varicose veins), greater blood volume is needed to maintain normal BP. Conversely, less volume is required to maintain normal BP with vasoconstriction. When high concentrations of strong vasoconstrictors such a norepinephrine and Ang II are present, the kidneys may actually undergo pressure-induced natriuresis and ECFV may decrease even though these vasoconstrictors also have important antinatriuretic effects which maintain the hypertension. Thus, in some forms of hypertension associated with marked peripheral vasoconstriction (eg, pheochromocytoma or renin secreting tumor) there may be reduced blood volume even though impaired renal-pressure natriuresis is necessary for the hypertension to be sustained.
Salt Sensitivity and Hypertension
Because excess salt intake increases the risk for hypertension, moderation of salt intake is an important strategy for prevention of cardiovascular and kidney disease, especially in salt-sensitive subjects. Although there is significant heterogeneity of BP responses to high salt intake over several days, BP salt sensitivity may worsen with chronic exposure to excessive salt intake. Salt sensitivity may also increase with aging or with various pathophysiologic conditions that cause kidney dysfunction, such as diabetes, hypertension, and various types of kidney disease. Gene mutations or neurohormonal changes that increase renal tubule sodium reabsorption may also increase BP salt sensitivity. Salt sensitivity also occurs more frequently in blacks than whites. Despite the seemingly disparate causes of salt sensitivity, all individuals with salt-induced chronic increases in BP have the common characteristic of impaired renal-pressure natriuresis and maintenance of salt balance at the expense of increased BP.
Experimental and clinical studies indicate that several types of kidney-specific disorders increase BP salt sensitivity ( Table 5.1 ): (1) kidney injuries that cause loss of functional nephrons or decreased glomerular capillary filtration coefficient; (2) patchy (nonhomogeneous) increases in preglomerular resistance; (3) inability to modulate the RAAS appropriately; and (4) acquired or genetic disorders that directly or indirectly increase renal NaCl reabsorption, especially in the distal and collecting tubules. The various types of kidney dysfunction that induce salt-sensitive hypertension are distinct from those that cause salt-resistant hypertension.
Experimental Kidney-Specific Disorders |
|
Monogenic Human Disorders |
|
Loss of Nephrons and Kidney Injury Cause Salt Sensitivity
Although surgical removal of up to 70% of kidney mass does not generally cause marked hypertension, it does greatly enhance BP salt sensitivity. Partial kidney infarction, tubulointerstitial inflammation, immune cell infiltration of the kidneys, immunoglobulin A (IgA) nephropathy, hydronephrosis, and many other types of renal insults also increase BP salt sensitivity. In patients with chronic kidney disease, BP salt sensitivity increases exponentially as creatinine clearance decreases. Thus, acquired renal injuries attributed to aging, diabetes, hypertension, and various types of acute and chronic kidney injury, even when they are subtle, usually increase BP salt sensitivity.
Loss of functional nephrons or renal injury also makes the kidneys more susceptible to additional insults that impair their function or to additional challenges of sodium homeostasis. Thus, hypertension associated with excess mineralocorticoids is more severe after reducing kidney mass. Moreover, loss of substantial numbers of nephrons may initiate compensatory vasodilation and hyperfiltration of the surviving nephrons that eventually cause further nephron loss, greater salt sensitivity, higher BPs, and ultimately kidney failure in some patients.
Inability to Effectively Modulate the Renin-Angiotensin-Aldosterone System Causes Salt Sensitivity
When the RAAS is fully functional, sodium balance can be achieved over a wide range of intakes with minimal BP changes ( Fig. 5.4 ). However, excessive activity of the RAAS or fixed low activity of the RAAS increases BP salt sensitivity. As discussed later, one of the major functions of the RAAS is to permit wide variations in intake and excretion of sodium without large fluctuations in BP that would otherwise be needed to maintain the sodium balance.

Focal nephrosclerosis or patchy constriction of preglomerular blood vessels, as occurs with renal infarction, leads to increased renin secretion in ischemic nephrons and low levels of renin release by overperfused nephrons. Thus, the ischemic as well as overperfused nephrons are unable to adequately suppress renin secretion during high salt intake and BP becomes salt sensitive.
Another cause of decreased responsiveness of the RAAS is increased distal and collecting tubular sodium reabsorption, as occurs with mineralocorticoid excess or gene mutations that increase distal and collecting tubule reabsorption (eg, Liddle syndrome, apparent mineralocorticoid excess, Gordon syndrome, and glucocorticoid-remediable aldosteronism). In these conditions, excess sodium retention causes almost complete suppression of renin secretion, resulting in an inability to further decrease renin release and Ang II formation during high sodium intake. Consequently, BP becomes highly salt-sensitive.
Blockade of the RAAS, with angiotensin-converting enzyme (ACE) inhibitors, Ang II receptor blockers (ARBs), or mineralocorticoid receptor (MR) antagonists also makes BP more sensitive to changes in salt intake despite reducing BP in many hypertensive subjects. Thus, these antihypertensive drugs are generally much more effective when salt intake is normal or reduced than when salt intake in elevated.
Endothelin and Salt Sensitivity
Endothelin-1 (ET-1) is a potent vasoconstrictor but its renal actions, especially in the collecting ducts (CDs), are of special importance in long-term BP regulation and salt sensitivity. The CDs produce ET-1 which binds in an autocrine manner to endothelin A/B (ETA/B) receptors, causing inhibition of NaCl reabsorption. Salt/volume loading stimulates CD ET-1 production through local mechanisms that sense salt delivery and shear stress when tubular flow rate increases. Locally released ET-1 then activates ETB receptors and inhibits sodium reabsorption. Moreover, CD-specific deletion of ETB receptors increases BP salt sensitivity. CD-specific deletion of ET-1 production or deletion of ETA/B receptors in CDs produces even greater salt-dependent BP elevation than deficiency of ETB receptors alone. Blockade of ET-1 receptors also attenuates or abolishes hypertension in Dahl-salt sensitive rats and DOCA-salt hypertension.
Although ET-1 is a potent vasoconstrictor in many tissues, including the kidneys, and may stimulate SNS activity and regulate extravascular sodium storage, whether these extrarenal actions ultimately influence renal-pressure natriuresis, salt sensitivity and chronic BP regulation is uncertain. It is clear, however, that the renal actions of ET-1, especially in the CD, play a major role in protecting against salt-sensitive hypertension.
Genetic Causes of Salt-Sensitive Hypertension
Nearly all monogenic forms of hypertension discovered thus far share the common phenotypes of increased renal NaCl reabsorption and salt-sensitive hypertension ( Fig. 5.5 ). In contrast, those monogenic disorders associated with decreased NaCl reabsorption tend to have salt-sensitive reductions in BP. Although these disorders account for less than 1% of human hypertension, they provide additional examples of salt-sensitive hypertension associated with excessive reabsorption of NaCl in the kidney distal and collecting tubules.

Pseudohypoaldosteronism type 2 (Gordon syndrome) is caused by mutations of genes that encode WNK1 and WNK4, two members of the WNK family of serine-threonine kinases expressed in the distal nephron. Mutations of WNK1 are large intronic deletions that increase WNK1 expression whereas WNK4 mutations are missense and cause loss of function. Both mutations increase activity of thiazide-sensitive NaCl transporters in the distal nephron, and patients with these mutations are effectively treated with thiazide diuretics which lower BP chronically by inhibiting NaCl renal reabsorption.
Liddle syndrome is caused by gain of function mutations of the β or γ subunits of the epithelial sodium channel (ENaC) which cause increased sodium reabsorption, hypoaldosteronism and low plasma renin activity. This disorder is effectively treated with amiloride or triamterene, which block ENaC and inhibit collecting tubule reabsorption. Hypertension also resolved after transplantation of a normal kidney in a patient with Liddle syndrome. The finding that BP remained normal for at least 5 years after kidney transplantation, even though potential extrarenal effects associated with increased ENaC activity were still present, indicates that the kidney dysfunction plays a critical role in the pathogenesis of hypertension.
Apparent mineralocorticoid excess (AME) is a monogenic form of salt-sensitive hypertension caused by deficiency of 11β-HSD2 which causes glucocorticoid activation of MR. Although cortisol binds MR with high affinity, renal epithelial cells are normally “protected” by 11β-HSD2 which locally converts cortisol to cortisone, a steroid that does not avidly bind MR. Therefore, 11β-HSD2 deficiency causes excessive MR stimulation which activates ENaC in aldosterone-sensitive distal nephrons, leading to increased NaCl reabsorption and hypertension with characteristics similar to that caused by primary aldosteronism. After hypertension is established, most indices of kidney function appear normal, except for hypokalemia and impaired renal-pressure natriuresis, and multiple vascular abnormalities begin to appear as a consequence of increased BP. Evidence that kidney dysfunction rather than some extrarenal effect of 11β-HSD2 deficiency mediates this form of salt-sensitive hypertension comes from the finding that transplantation of normal kidneys into patients with AME caused complete remission of hypertension and electrolyte abnormalities.
Glucocorticoid-remediable aldosteronism (GRA), congenital adrenal hyperplasia, familial hyperaldosteronism not remedial by glucocorticoids, and hypertension exacerbated by pregnancy are also monogenic forms of salt-sensitive hypertension associated with excessive activation of the MR. All are effectively treated by drugs that block renal tubular ENaC, MR, or aldosterone secretion. In each case, the primary driving force for salt-sensitive increases in BP is increased distal and collecting tubule NaCl reabsorption.
Kidney Disorders That Cause Salt-Resistant Hypertension
Not all kidney disorders increase salt sensitivity of BP. Generalized increases in preglomerular resistance caused by suprarenal aortic coarctation or constriction of one renal artery and removal of the contralateral kidney (1-kidney, 1-clip Goldblatt hypertension) cause salt-resistant hypertension. After constriction of the renal artery or aorta, GFR and sodium excretion initially decrease and renin secretion increases. As BP rises and pressure distal to the stenosis returns toward normal, most measurements of kidney function, including sodium excretion and renin secretion, return to nearly normal if the constriction is not too severe. If renal artery constriction of a sole remaining kidney is severe, malignant hypertension may develop.
A major reason that high salt intake does not usually exacerbate hypertension caused by increased preglomerular resistance is that after BP increases sufficiently to restore renal perfusion pressure and renin secretion to normal, the RAAS is fully capable of appropriate suppression during high salt intake. As discussed previously, the ability to effectively modulate RAAS activity is a key mechanism for preventing salt sensitivity of BP.
Gain-of-function phosphodiesterase 3A (PDE3A) mutations, in contrast to all other forms of monogenic hypertension that have been discovered, cause increased vascular resistance and salt-resistant hypertension. This is an autosomal-dominant form of hypertension associated with brachydactyly and is caused by increased activity of PDE3A which catalyzes hydrolysis of intracellular second messengers, cAMP and cGMP. The mutated PDE3A causes vascular smooth muscle cell proliferation and vasoconstriction, leading to increased TPR and presumably increased renal vascular resistance. Sympathetic blockade and hydrochlorothiazide treatment are ineffective in reducing BP whereas nitroprusside caused acute decreases in BP, consistent with an intrinsic vascular abnormality. Despite marked vasoconstriction and increased TPR, these patients are not salt-sensitive and have normal renin, aldosterone and norepinephrine.
Thus, generalized vasoconstriction does not appear to increase BP salt sensitivity in the absence of kidney abnormalities that increase tubular reabsorption, decrease glomerular filtration coefficient, and/or reduce responsiveness of the RAAS. Renal preglomerular vasoconstriction can increase BP but the hypertension is usually not salt-sensitive.
Clinical Assessment and Significance of Salt Sensitivity
Although various experimental methods have been used to assess salt sensitivity, none are widely used in clinical practice. Most salt sensitivity protocols involve relatively short-term changes in sodium intake, usually over a few days. For example, salt sensitivity has also been defined as a 10 mm Hg or greater increase in mean BP from the level measured after a 4-hour infusion of 2 L of normal saline compared with the level measured the morning after 1 day of a low-sodium (10 mmol) diet and administration of three doses of furosemide. Using this protocol, Weinberger reported that 51% of hypertensive and 26% of normotensive subjects were salt-sensitive. However, it is unclear whether these short-term protocols reliably predict the long-term effects of changes in salt intake.
In most cases, salt sensitivity is determined empirically by encouraging patients to reduce their salt intake and measuring their BP responses. Some studies suggest that those patients who are salt sensitive may have greatest risk for hypertensive target-organ injury and early death compared with those who are salt-resistant. Weinberger et al followed subjects for more than 20 years and found that normotensive individuals with increased salt sensitivity died almost at the same rate as hypertensive individuals and much faster than salt-resistant individuals who were normotensive ( Fig. 5.6 ). Whether this increased mortality was related to BP effects of salt or to other effects is still unclear. It is also not known whether chronic high salt intake, lasting over many years, may cause a person who is initially “salt-insensitive” to become “salt-sensitive” as a consequence of gradual renal injury.

There is evidence that salt-sensitive forms of hypertension are often associated with glomerular hyperfiltration and increased glomerular hydrostatic pressure that is further amplified by the hypertension ; together the hypertension and renal hyperfiltration may promote glomerular injury and may eventually cause loss of nephron function. Clinical studies support this concept and demonstrate that salt-sensitive individuals typically have substantially higher glomerular hydrostatic pressure and albumin excretion when given a salt load compared with salt-resistant individuals. Further studies are needed to assess the overall impact of BP salt sensitivity in normotensive and hypertensive subjects and the mechanisms that may link salt sensitivity to target organ injury and premature death.
Renin-Angiotensin Aldosterone System
The RAAS is one of the body’s most powerful systems for regulating sodium balance and BP as evidenced by the effectiveness of RAAS blockers in reducing renal tubular sodium reabsorption and lowering BP in normotensive as well as hypertensive subjects. Although the RAAS has many components its most important effects on sodium excretion and chronic BP regulation are exerted by Ang II and aldosterone. Both Ang II and aldosterone potently increase sodium reabsorption whereas Ang II also has important renal hemodynamic effects that contribute its antinatriuretic actions and long-term BP regulation.
Ang II and Long-Term Blood Pressure Regulation
Ang II has powerful vasoconstrictor effects that help maintain BP during hemorrhage, dehydration, heart failure and other disturbance that cause circulatory depression and/or volume depletion. However, Ang II also plays a key role in chronic BP regulation via its sodium-retaining effects on the kidneys. As discussed previously, activation of the RAAS during low salt intake and appropriate suppression during high salt intake permits maintenance of balance with minimal changes in BP over a wide range of sodium intakes.
RAAS antagonists (ACE inhibitors, ARBs, renin inhibitors, MR antagonists) improve the kidneys’ ability to excrete sodium and permit sodium balance at lower BP. However, RAAS blockade also makes BP more salt-sensitive because the actions of Ang II and/or aldosterone are already blocked and therefore cannot be effectively suppressed during high salt intake. Conversely, RAAS antagonists are more effective in lowering BP with concomitant diuretic treatment or after reducing salt intake.
Ang II causes salt and water retention by direct actions on the kidneys that increase NaCl reabsorption and by stimulating the adrenal glands to release aldosterone. Ang II also constricts renal efferent arterioles which reduces renal blood flow and peritubular capillary hydrostatic pressure while increasing filtration fraction and peritubular colloid osmotic pressure; together these renal hemodynamics effects enhance peritubular capillary reabsorption and consequently tubular reabsorption of salt and water. In addition, reductions in renal medullary blood flow or direct effects of Ang II on the vasa recta may enhance sodium reabsorption in the loop of Henle and CDs.
Ang II directly stimulates sodium reabsorption through its actions on luminal and basolateral membranes of the renal tubules. Ang II stimulates proximal tubular sodium reabsorption by increasing activities of the Na + /H + exchanger and Na + /K + ATPase. Furthermore, Ang II stimulates Na + /K + /2Cl − transport in the loop of Henle as well as multiple ion transporters in the distal nephron and collecting tubules to increase NaCl reabsorption.
Ang II acts primarily on two receptors. Activation of the AT1 receptor leads to vasoconstriction, increased renal NaCl transport, and release of aldosterone which all ultimately lead to salt and water retention. The AT2 receptor opposes the function of the AT1 receptors and inhibits cell proliferation, promotes cell differentiation, and causes vasodilation and natriuresis. Compared with the AT1 receptor, AT2 receptor expression is relatively low in adult animals, although in some circumstances (eg, wound healing) AT2 receptor expression may increase significantly.
Although the chronic BP effects of Ang II have often been attributed to its effects on the brain, adrenal gland, and nonrenal blood vessels, activation of kidney AT1 receptors is required for Ang II to cause chronic hypertension ( Fig. 5.7 ). Crowley and colleagues found that Ang II infusion in wild-type (WT) mice increased BP and caused cardiac hypertrophy and fibrosis. In contrast, WT mice that received transplanted kidneys from AT1 receptor knock-out mice (ie, AT1 receptors were present in the peripheral vasculature, brain, heart and other organs, but not in the kidneys), Ang II infusion did not raise BP chronically or cause cardiac hypertrophy/fibrosis. In AT1 receptor knock-out mice that received transplanted kidneys from WT mice (ie, AT1 receptors were present only in the kidneys and not in peripheral blood vessels, brain, heart or other organs), Ang II infusion caused chronic increases in BP, albeit more slowly, as well as cardiac hypertrophy/fibrosis that were similar to that found in WT mice. These observations indicate that kidney AT1 receptor activation by Ang II, instead of peripheral vascular or other nonrenal effects, mediate chronic increases in BP and cardiac hypertrophy.

Mechanisms of Ang II-Mediated Target Organ Injury
Ang II has been suggested to cause damage to the kidneys, heart and other organs by both hemodynamic and direct tissue effects. Much of the evidence for nonhemodynamic effects of Ang II in promoting target organ injury comes from in vitro studies, often using supraphysiologic doses of Ang II, but also from in vivo studies suggesting that RAS blockers (ACE inhibitors and ARBs) reduce target organ damage (ie, chronic kidney disease [CKD] and left ventricular hypertrophy) more than other antihypertensive drugs. However, high Ang II levels in the absence of elevated BP do not appear to cause target organ damage. One example is the two-kidney, one-clip Goldblatt hypertension model where the clipped kidney is exposed to very high Ang II levels but is protected from high BP by the clip. The clipped kidney (as long as the stenosis is not too severe) is protected from renal injury whereas the nonclipped kidney which is exposed to lower Ang II levels but higher BP demonstrates substantial injury. Convincing evidence that the hemodynamic effects of Ang II are essential for target organ injury comes from the experiments by Crowley et al discussed previously. In these studies, cardiac fibrosis and hypertrophy did not occur during chronic Ang II infusion unless BP also increased, and BP did not increase unless AT1 receptors were present in the kidneys.
Aldosterone, Mineralocorticoid Receptor Activation, and Long-Term Blood Pressure Regulation
Aldosterone, a mineralocorticoid synthesized in the zona glomerulosa of the adrenal cortex, is secreted mainly in response to increases in extracellular concentrations of Ang II and potassium, but several other factors associated with changes in body fluid volumes and stress can also influence aldosterone secretion. In humans about 90% of mineralocorticoid activity is normally from aldosterone. Aldosterone stimulates MR in the principal cells of the distal tubules, cortical collecting tubules and the CDs to increase sodium reabsorption and potassium secretion.
Aldosterone exhibits both genomic and nongenomic effects on the cardiovascular and renal systems. Genomic effects are those mediated by gene transcription and require 60 to 90 minutes to occur after the MR is activated. Genomic effects of aldosterone include synthesis and insertion of Na + /K + -ATPase pump proteins into the basolateral membrane as well as amiloride-sensitive sodium channels in the luminal membrane of the principal cells, leading to increases in renal sodium reabsorption and potassium secretion. Although the membrane receptor and the cell-signaling mechanisms responsible for nongenomic actions of aldosterone are not well-understood, the effects are rapid. For example, aldosterone may activate the Na + -H + exchanger in vascular smooth muscle in less than 4 minutes. Currently, the functional significance of aldosterone’s nongenomic effects on BP regulation has not been elucidated.
The effects of aldosterone on renal-pressure natriuresis are similar to those of Ang II. When salt intake is reduced, aldosterone is released to increase renal sodium reabsorption, thereby attenuating sodium loss and preventing large reductions in BP. With high salt intake, aldosterone is suppressed, attenuating sodium retention and increased BP. Excessive aldosterone, by stimulating renal sodium reabsorption, impairs pressure natriuresis and makes BP more salt-sensitive. However, even when aldosterone levels are elevated up to ten-fold, BP may not be elevated if sodium intake is low. Normal or high salt intake also appears to be a requirement for target organ damage associated with increased aldosterone. Thus, high concentrations of aldosterone during low sodium diet are not associated with increased BP or target organ injury.
When occurring concomitantly with normal or high sodium intakes, high levels of aldosterone and MR activation cause excess sodium retention, hypertension and target organ injury. Hyperaldosteronism or failure to adequately suppress aldosterone in response to sodium retention may be more common than previously believed, especially in patients with hypertension resistant to treatment with the usual antihypertensive medications. Some investigators have suggested that the prevalence of primary aldosteronism is as high as 20% of patients referred to specialty clinics for resistant hypertension. Moreover, blockade of MR or amiloride-sensitive sodium channels lowers BP and attenuates cardiovascular and renal injury in many treatment resistant hypertensive subjects even when aldosterone levels are not substantially elevated above normal. Convincing evidence for the effectiveness of MR blockade in treatment resistant hypertension not associated with high levels of aldosterone comes from the PATHWAY-2 (The Prevention And Treatment of Hypertension With Algorithm based therapY) study which also provided evidence that sodium retention plays a key role in the hypertension of the patients.
Many treatment-resistant hypertensive patients who respond to MR blockers or amiloride are overweight or obese. As discussed later, obesity may activate MR and increase renal ENaC activity independent of aldosterone. Furthermore, administration of MR antagonists to obese hypertensive patients reduced BP despite concomitant treatment with ACE inhibitors, ARBs, calcium channel blockers or thiazide diuretics suggesting that MR activation in obese humans may occur independently of Ang II-mediated aldosterone secretion.
Sympathetic Nervous System
The SNS plays a major role in short-term and long-term control of BP. Almost all components of the vasculature and the heart are innervated by sympathetic fibers. SNS activation can increase BP within seconds through its vasoconstrictor effects and by increasing cardiac output (increased chronotropic and inotropic effects). There are multiple levels of the nervous system that can modulate SNS activation including the central nervous system (CNS), ganglionic transmission, release, clearance and reuptake of neurotransmitters, and sensitivity or density of adrenergic receptors.
The renal sympathetic nerves appear to play a major role in long-term control of BP and in the pathogenesis of hypertension. This is evidenced by the effect of renal denervation (RDN) to lower BP in several animal models of experimental hypertension. The renal blood vessels, juxtaglomerular apparatus, and renal tubules are extensively innervated and excessive activation of these nerves promotes sodium retention, increased renin secretion, and impaired renal-pressure natriuresis. Although SNS activation in most forms of hypertension is generally not great enough to reduce renal blood flow and GFR, even mild SNS activation can increase renin release and renal sodium reabsorption in various parts of the nephron including the proximal tubules, loop of Henle, distal tubule, and collecting tubules. The renal nerves therefore provide a mechanism to connect the SNS with control of body fluid volumes and long-term BP regulation.
Multiple studies (eg, SYMPLICITY HTN-1 and HTN-2) suggest that the SNS may contribute to human hypertension via activation of the renal sympathetic nerves. As discussed later in the section on obesity-induced hypertension, renal sympathetic activity is often increased in obese individuals, especially those with increased visceral adiposity. Clinical trials that have ablated the renal nerves using percutaneous procedures to treat resistant hypertensive patients have reported substantial reductions in BP that persisted for as long as 3 years. However, the SYMPLICITY HTN-3 trial, which included a sham surgery arm, failed to demonstrate significant reductions in 24-hour ambulatory BP after RDN beyond those observed in the sham control group. The reasons for these differences in BP responses in the SYMPLICITY HTN-1, HTN-2 and HTN-3 trials of RDN in treatment-resistant hypertension are still unclear and have been the subject of much speculation.
One obvious explanation for failure of RDN to lower BP in these trials is that the patients were already on at least three antihypertensive medications, including blockers of the RAAS which may mediate at least part of the effect of the renal nerves on BP. Another possible explanation is that the effectiveness of the procedures may have differed in various trials because the extent of RDN was not verified. The usual radiofrequency method causes only 40% to 50% RDN even under optimal procedures. Experimental studies indicate that up to 75% ablation of the renal nerves can be achieved if the radiofrequency ablation includes all branches of the main renal artery close to the hilum of kidneys. However, this extensive denervation was not achieved in any of the SYMPLICITY trials. Further studies will be required to determine whether RDN is an effective therapy for patients with resistant hypertension and improved methods are needed to predict which patients will benefit most from RDN. Also, longer follow-up periods will be needed to determine if the renal nerves eventually regrow and reinitiate increases in BP as observed in experimental animal models of RDN.
Excessive sympathetic activation clearly plays a major role in contributing to hypertension in many patients, especially those who have visceral obesity. As discussed previously, obesity accounts for much of the risk for human essential hypertension. In the section on primary hypertension, we discuss some of the mechanisms that may contribute to SNS activation and hypertension in obese subjects. There are, however, many additional factors besides obesity that have been proposed to cause SNS activation in hypertension, as discussed in several excellent reviews. Two factors that have recently received considerable attention include baroreceptor dysfunction and chemoreceptor activation of the SNS.
Arterial Baroreceptors and Long-Term Blood Pressure Regulation
The role of the arterial baroreflex system in moment-to-moment regulation of BP is well known but its importance in long-term BP control remains controversial. Although unloading the baroreceptors by carotid sinus denervation markedly increases BP variability, the average 24-hour mean arterial pressure is not substantially altered after a few days. Also, in chronic hypertension there may be resetting of the arterial baroreceptors to higher BPs, leading to the suggestion that the baroreflex may have little role in long-term BP regulation. To the extent that resetting of baroreceptors occurs, their potency as a long-term controller of BP would be diminished. This suggestion is bolstered by the observation that arterial baroreceptor denervation in dogs may increase the rapidity of the rise in BP but not the eventual severity of several forms of chronic hypertension. In contrast, studies in rodents suggest that baroreceptor denervation exacerbates increased BP induced by a chronic high salt diet. Other studies also suggest that baroreceptors may not completely reset in hypertension and therefore buffer increases in BP. Thus, it is still unclear whether baroreceptor dysfunction merely alters the time course for onset of hypertension or plays an important role in long-term BP regulation.
Chronic electric stimulation of the afferent nerves of the carotid sinus baroreceptors produces sustained reductions in SNS activity and BP in normotensive dogs and hypertensive obese dogs. Although baroreflex activation by electric stimulation of carotid sinus nerves also caused transient reductions in BP in dogs infused with Ang II or aldosterone, minimal long-term effects on BP were observed in these models of hypertension. In humans with treatment-resistant hypertension, however, electrical stimulation of baroreceptors caused significant and sustained reductions in BP. These observations indicate that strong chronic activation of the carotid sinus nerves can lower BP in humans with treatment-resistant hypertension and in some, but not all, forms of experimental hypertension.
Electric stimulation of the carotid sinus afferent nerves bypasses the mechanosensors that may contribute to baroreceptor resetting in chronic hypertension. Therefore, the observation that chronic stimulation of baroreceptor afferents lowers BP does not necessarily reveal the physiologic importance of the arterial baroreceptors in long-term BP regulation.
Although the physiologic role of baroreceptor dysfunction in contributing to chronic hypertension is still controversial, there is little doubt that impaired baroreflexes lead to increased BP lability. There is also evidence that large swings in BP associated with impaired baroreflexes eventually cause renal injury that could exacerbate the impact of other hypertensive stimuli. For example, baroreceptor-denervated animals have significant glomerular injury as well as cardiac hypertrophy. Therefore, it seems likely that the arterial baroreceptors play a significant role in protecting the heart, blood vessels and kidneys against injury that would otherwise occur with heightened lability of BP.
Peripheral Chemoreceptors and Blood Pressure Regulation
The carotid bodies are chemosensors that initiate reflex increases in ventilation and SNS activity in response to hypoxemia. These chemoreceptors may interact with arterial baroreceptors such that chemoreceptor activation impairs baroreceptor sensitivity whereas carotid body inhibition and/or resection improve baroreflex function. Studies in spontaneous hypertensive rats (SHR) and in patients with primary hypertension suggest that tonic increases in peripheral chemoreceptor activity may contribute to sustained increases in SNS activity, including renal sympathetic nerve activity (RSNA), and hypertension. For example, denervation of the carotid body reduced RSNA and attenuated hypertension in SHR. Deactivation of carotid body chemoreceptors by respiration with 100% oxygen reduced muscle SNS activity in hypertensive men but not in control subjects.
Surgical removal of the carotid body for treatment of bronchial asthma or chronic obstructive pulmonary disease has been reported to cause a significant decrease in BP that was sustained for 6 months in hypertensive patients, whereas no reduction in BP was seen in normotensive patients and a rise in BP was found in hypotensive patients. However, there have been no clinical trials to examine the effect of unilateral carotid body resection for hypertension in humans.
Repeated activation of peripheral chemoreceptor in sleep apnea has been suggested to contribute to increased BP as well as metabolic derangements in metabolism in obese subjects. However, establishing cause and effect relationships between chemoreceptor activation and hypertension in obese patients with sleep apnea have been challenging. Even in the absence of obstructive sleep apnea, obesity may activate or sensitize the carotid chemoreceptors. Hypoxemia has been reported in some subjects with obesity, although its overall prevalence in obesity is unclear. Lohmeier and colleagues reported that obese dogs fed a high fat diet for only 5 weeks had increases in BP and respiratory rate along with hypoxemia. Moreover, denervation of the carotid sinus region attenuated increases in BP in obese dogs and transiently reduced respiratory rate while exacerbating hypoxemia. These findings suggest that hypoxemia may account for stimulation of peripheral chemoreceptors in obese dogs and that this activation may cause compensatory increases in ventilation and central sympathetic outflow that contributes to neurogenically-mediated increases in BP. However, the role of hypoxemia and peripheral chemoreceptor stimulation in contributing to hypertension in humans is still unclear.
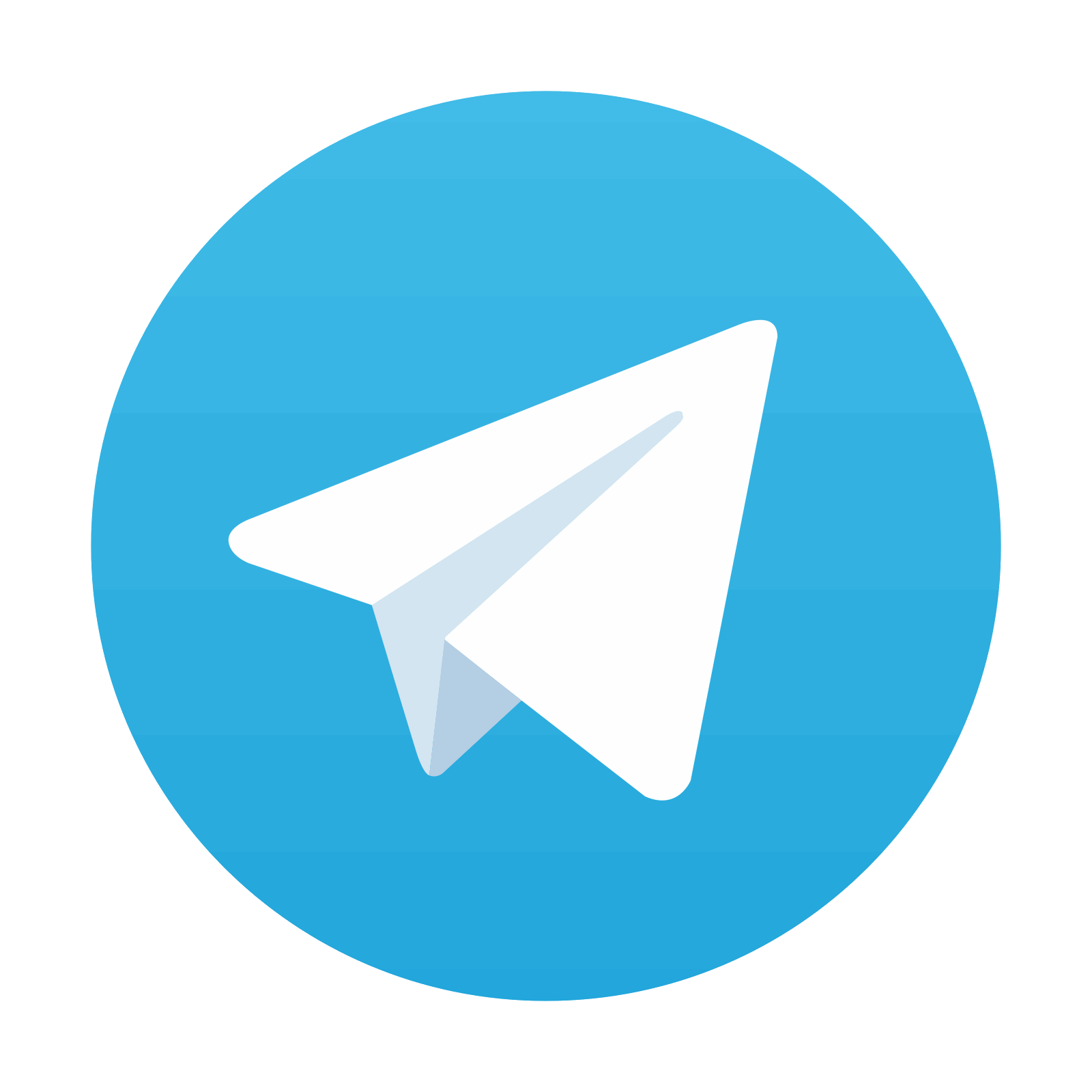
Stay updated, free articles. Join our Telegram channel

Full access? Get Clinical Tree
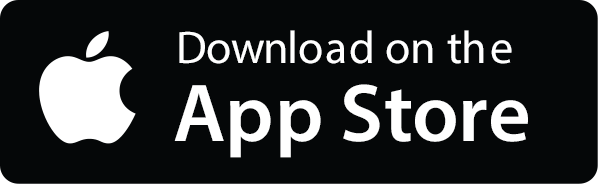
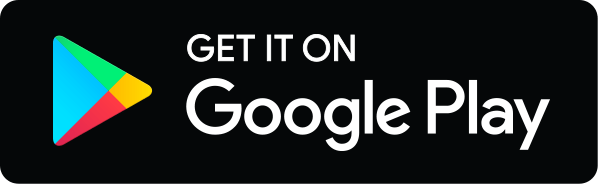