Patent Ductus Arteriosus and Aortopulmonary Window
Nathaniel W. Taggart
Mohammed Yasir Qureshi
Patent Ductus Arteriosus
Introduction
The ductus arteriosus is a normal fetal structure that develops from the sixth branchial arch by the sixth week of gestation and allows communication between the aorta and pulmonary arteries (PAs). The ductus arteriosus is an obligatory part of normal fetal circulation that allows blood returning to the heart to bypass the lungs, and instead flow directly into the descending aorta. After birth, in the absence of severe cyanotic heart disease or persistently elevated pulmonary vascular resistance, a patent ductus arteriosus (PDA) may be pathologic, presenting a detrimental pressure load to the pulmonary circulation, a volume load to the left heart, or an increased risk of endocarditis over time.
Epidemiology
Because the ductus arteriosus is a necessary part of normal fetal circulation, the presence of a patent ductus arteriosus immediately after birth can be assumed to be nearly universal. In most cases, the ductus constricts in response to increased blood oxygen content and other factors described below. While ductal constriction usually leads to functional and anatomic closure, in some cases the ductus may close only partially or not at all. Historical estimates have placed the incidence of isolated PDA at approximately 0.05% of all live births. Isolated PDA accounts for 5% to 10% of congenital heart defects (1,2). This number most likely represents the prevalence of a “symptomatic” PDA—that is, one that results in evidence of increased pulmonary blood flow, left heart volume overload, elevated PA pressure, murmur, etc. With the advent of color Doppler echocardiography, the incidental recognition of asymptomatic “silent” ductus has become more common. Some have estimated the prevalence of silent PDA among children and adults to be up to 0.5%, far more common than the “symptomatic” PDA (3). For reasons that are not clear, females are more commonly affected by PDA than males, by a ratio of around 2:1 (4).
Etiology
The precise cause of persistent postnatal ductal patency is not known. Our understanding of the etiology and development of a PDA is shaped primarily by our understanding of ductal physiology during fetal life and the process of normal ductal closure during the transition to postnatal circulation.
The Ductus Arteriosus in the Fetus
In the normal fetus, the ductus arteriosus is a vascular structure connecting the PAs and the descending aorta. The PA end of the ductus usually arises from the proximal left pulmonary artery (LPA) just after the bifurcation of the main pulmonary artery (MPA). High fetal pulmonary arteriolar resistance combined with relatively low placental and fetal systemic vascular resistance results in right to left (PA to aorta) flow across the ductus. Patency of the ductus in this scenario is influenced to a varying degree by a number of factors, including blood oxygen content and circulating prostaglandins.
Oxygen
One significant factor contributing to ductal patency in the fetus is the low dissolved oxygen concentration (pO2) supplied to the ductus. The pO2 of blood in the lumen of the PDA is approximately 18 mm Hg, while the pO2 of the vasa vasorum of the ductus is approximately 26 mm Hg (5). By contrast, the aortic dissolved oxygen concentration shortly after birth is near 100 mm Hg. Higher pO2 levels promote smooth muscle constriction within the wall of the ductus, probably by triggering calcium influx into the cell (6,7,8). Conversely, the low pO2 in fetal blood prevents such calcium influx and smooth muscle contraction, and helps to maintain ductal patency.
Prostaglandins
Prostaglandins are hormone-like compounds synthesized from arachidonic acid by the enzyme cyclooxygenase (COX) within the walls of the ductus arteriosus (9). Prostaglandins act to relax ductal smooth muscle resulting in vasodilation and patency of the ductus. The two prostaglandins produced in the ductus are prostaglandin E2 (PGE2) and prostacyclin (PGI2). Circulating PGE2 in fetal blood probably plays the larger role in maintaining patency of the ductus arteriosus in normal fetal circulation. Following birth, there is a rapid decrease in the concentration of PGE2, which, along with an increase in blood oxygen concentration, contributes to functional closure of the ductus arteriosus (10,11). Not surprisingly, intravenous administration of exogenous PGE1 (a synthetic variant of PGE2) can prevent ductal closure in the context of ductal-dependent cyanotic and obstructive congenital heart lesions (12,13,14).
Conversely, inhibition of PGE2 synthesis using aspirin-like COX inhibitors (such as nonsteroidal anti-inflammatory drugs, indomethacin, or ibuprofen) facilitates ductal closure in small or premature neonates (15,16).
Conversely, inhibition of PGE2 synthesis using aspirin-like COX inhibitors (such as nonsteroidal anti-inflammatory drugs, indomethacin, or ibuprofen) facilitates ductal closure in small or premature neonates (15,16).
Other Factors
Other factors have been shown to affect vasodilation and vasoconstriction of the ductus arteriosus. Nitric oxide (NO), a potent pulmonary vasodilator, has been shown to prevent ductal constriction in animal models (17,18). Nitric oxide synthase, the enzyme responsible for producing NO is expressed on ductal endothelial cells.
Adenosine has also been shown to cause vasodilation of the ductus arteriosus. Studies in lambs have shown that the concentration of adenosine is much higher in the fetus than in the neonate (19). These findings have led some to speculate about a potential role of adenosine in regulating patency of the ductus arteriosus (20).
Normal Ductal Closure
With a baby’s first breath, alveolar oxygen concentration increases, resulting in pulmonary vasodilation. In addition, removal of the low resistance placenta from systemic circulation decreases the ratio of pulmonary vascular resistance (Rp) to systemic vascular resistance (Rs). This drop in Rp/Rs produces a change in direction of blood flow across the ductus arteriosus, which then flows from aorta to PA. At this point in the transition to postnatal life, the ductus arteriosus is exposed to systemic arterial pO2, which in room air at sea level is near 100 mm Hg. This high systemic arterial pO2 causes vasoconstriction of the ductus arteriosus through mechanisms that have not been completely elucidated. Smooth muscle contraction may be promoted by oxygen-sensitive potassium channels that in turn activate voltage-sensitive calcium channels that allow calcium influx into smooth muscle cells, resulting in smooth muscle contraction (6,21). Other mechanisms may also be involved (7).
Initial, “functional” closure of the ductus arteriosus is caused by smooth muscle contraction resulting from increased blood pO2 and decreased concentration of circulating prostaglandins. Functional closure occurs within the first 24 hours of life in about half of all healthy, term neonates and is nearly universal by 72 hours of life (22). Even after functional closure the ductus can still be “re-opened” within the first few days of life by the administration of PGE1.
In most cases, the constriction of the ductus begins at the PA end and progresses along its course toward the aortic end. Because most PDAs result from incomplete ductal closure rather than a complete lack of constriction, the narrowest diameter of the PDA is usually closer to the PA. The aortic end of the ductus is usually wider in diameter and is often referred to as the ductal ampulla.
Following functional closure of the ductus arteriosus, lower pO2 and thickening of the ductal walls due to smooth muscle contraction produce hypoxia of the inner layers of the ductus. This hypoxia leads to cell destruction and fibrosis, and ultimately, anatomic (permanent) obliteration of the ductal lumen (23,24,25). The fibrous strand that remains in place of the ductus arteriosus is known as the ligamentum arteriosum.
PDA in the Premature Neonate
Persistent patency of the ductus arteriosus after birth is much more common among preterm neonates than among otherwise healthy, term neonates. One proposed factor that may contribute to this increased risk of PDA is persistence of relatively low arterial pO2 in the preterm neonate (5). This low pO2 may result from poor alveolar-capillary oxygen diffusion due to immature lungs. It may also be compounded by persistently elevated pulmonary vascular resistance leading to right-to-left shunting across the ductus. Low pO2 by itself, however, is insufficient to explain the increased incidence of PDA in premature infants. Animal studies have suggested that the premature ductus may be less responsive to the vasoconstrictive effects of O2 (8). Furthermore, because PGE2 is primarily metabolized in the lungs, infants with premature lungs may manifest impaired metabolism and elevated circulating levels of PGE2. Others have suggested that ductal tissue in the premature neonate may contain increased levels of NO synthetase, and that higher levels of circulating NO may be primarily responsible for patency of the ductus in that population (26).
PDA at High Elevations
PDA Associated with Other Congenital Cardiovascular Defects
PDA is a common associated finding in the presence of other more severe congenital cardiovascular defects. A PDA may be necessary to support oxygenation and oxygen delivery in “ductal-dependent” cyanotic and obstructive defects. While it is not exactly known why PDA is more common in these situations, this increased incidence is likely due to decreased ductal blood pO2. In ductal-dependent lesions, ductal constriction often occurs even in the setting of severe cyanosis and should be managed with exogenous PGE1 administration.
PDA Associated with Congenital Rubella Syndrome
Congenital rubella syndrome (CRS) is a constellation of congenital abnormalities that includes growth retardation due to placental insufficiency, deafness, cataracts, congenital heart defects, and neonatal purpura with thrombocytopenia. CRS results from rubella infection usually within the first 16 weeks of gestation (29,30). The most common congenital heart defects associated with CRS are PDA and branch PA stenosis. PDA occurs in 60% to 70% of patients with CRS. The histology of PDA in CRS has been described as “immature” and may result from an arrest in ductal development due to the rubella virus (31).
PDA Associated with Genetic Disorders
The relative frequency of PDA in the general population argues against a single genetic or environmental cause. However, epidemiologic data support an increased risk of PDA (approximately 3%) among siblings of affected individuals, suggesting that there is a genetic predisposition in some families (32). One group identified a recessive gene locus (12q24) that may be accountable for up to one-third of PDAs in their study population (33). However, this population had a higher rate of PDA (approximately 15% of congenital heart defects) and a high rate of parental consanguinity, suggesting that this recessive gene may contribute less to the incidence of PDA in other cultures.
Char syndrome is an autosomal dominant “heart-hand” syndrome associated with PDA. Typical facial features include short philtrum, prominent lips (sometimes referred to as “duck-billed”), flattened nasal bridge with upturned nose, and abnormalities of the fifth finger (often absence or hypoplasia of the phalanges). A missense mutation in the TFAP2B gene on chromosome 6p has been shown to cause Char syndrome (34,35,36). Subsequent to this
discovery, mutations in TFAP2B have been identified in cases of familial PDA without the clinical phenotype of Char syndrome (37,38).
discovery, mutations in TFAP2B have been identified in cases of familial PDA without the clinical phenotype of Char syndrome (37,38).
Morphology
Normal Anatomy of the Ductus Arteriosus
The normal ductus arteriosus travels from the anterior surface of the aortic isthmus to the origin of the LPA. Passing to the left of the trachea, it is designated a “left-sided” ductus. The origin of the ductus from the aorta is inferior to its connection with the LPA such that the ductus runs anterior and superior in its course from aorta to PA. The left recurrent laryngeal nerve courses along the left side of the ductus before hooking under the ductus and heading cephalad. The anatomic arrangement of the recurrent laryngeal nerve relative to the ductus arteriosus is an important consideration during surgical ligation.
Morphologic Classification
Morphologic classification of PDAs was largely established in the late 1980s by the group from Hospital for Sick Children in Toronto (39). They identified five reasonably consistent ductal morphologies based upon the location of the narrowest diameter of the vessel (Fig. 31.1):
Type A refers to a “funnel”- or “conical”-shaped PDA consisting of a prominent ductal ampulla on the aortic end. The ampulla tapers quickly and the narrowest diameter of the ductus is at the PA end. Type A morphology is by far the most common, comprising about 65% of all PDAs in the study from Toronto.
Type B, sometimes referred to as a “window-type” ductus, has a much shorter course and the narrowing is at the aortic end without a significant ductal ampulla and was found in 18% of patients. Both Type A and Type B were further subclassified in the original article based upon the anterior–posterior relationship of the ductal narrowing to the trachea.
Type C PDA is long and tubular without significant narrowing along its course.
Type D PDA has multiple areas of constriction.
Type E PDA is long with a narrowing that is well anterior to the trachea.
In the Toronto series, Types C, D, and E each comprised less than 10% of PDAs.
Anatomic Variations in Ductal Anatomy
While the typical ductus is left sided and usually falls into one of the morphologic types described above, there can be tremendous variation, particularly in the presence of other congenital cardiovascular abnormalities. A right-sided ductus that travels to the right of the trachea and enters the base of the right pulmonary artery (RPA) is an unusual finding. A right-sided ductus can be seen with a left or right aortic arch and may be a component of a vascular ring. In some cases, the ductus arteriosus can originate from the brachiocephalic branches of the aorta rather than from the aorta itself.
Physiology
The clinical significance of a PDA is primarily determined by the volume of blood shunting across it and the effect over time of high-pressure systemic-to-pulmonary blood flow. The blood flow across a PDA depends upon the total resistance to flow relative to systemic vascular resistance. The resistance to flow across the PDA includes both the intrinsic resistance of the ductus itself and the resistance of the distal pulmonary vascular bed. If a PDA is large, such that there is no drop in pressure across it (i.e., PA and aortic pressures are equal), the resistance within the PDA would be negligible, and therefore the volume of shunt across the PDA would be solely determined by the relationship between the pulmonary vascular resistance (Rp) and systemic vascular resistance (Rs). This relationship between the resistance and flow is described quantitatively in the following modification of Ohm’s law (P = pressure; Q = blood flow):

Assuming Pp = Ps:

or

With this relationship, any increase in the resistance of one vascular bed will result in a proportional decrease in blood flow through that bed. Because normal Rp is usually approximately 25% of Rs after the first months of life, a large, nonrestrictive PDA in the setting of normal resistance would result in a calculated Qp/Qs of 4:1. A shunt with such high pulmonary blood flow (which is rarely seen because most PDAs exhibit some degree of ductal constriction) produces a volume load with consequent dilation of the chambers and vessels, through which the flow passes: the pulmonary vasculature, left atrium, and left ventricle. The cardinal features that define a hemodynamically important ductus arteriosus beyond the neonatal period are left atrial and left ventricular enlargement. This chamber enlargement is a function not only of shunt volume but also of time, and therefore is progressive. Symptoms of congestive heart failure and pulmonary edema may also develop. In addition, such a high-volume, high-pressure shunt over time can lead to irreversible pulmonary vascular disease (Eisenmenger syndrome).
In most cases of PDA, there is some degree of constriction of the ductus arteriosus that limits flow to the PAs independent of Rp/Rs. Likewise, the pressure effect of ductal flow is restricted and the risk of pulmonary vascular disease is significantly lower. These patients typically present with a cardiac murmur, the character of which depends upon the size of the PDA and Rs/Rp. Depending upon the overall shunt volume, they may also have some degree of left heart enlargement.
Clinical Features
The presentation of PDA depends upon patient age and the size of the PDA. A preterm neonate with a large ductus will not have the typical “machinery” murmur of a PDA. Hypotension with widened pulse pressure, excessive pulmonary blood flow, and pulmonary edema is the more likely presentation. A neonate with elevated but subsystemic pulmonary vascular resistance and a restrictive ductus may manifest only a systolic murmur because of low diastolic flow velocity across the ductus. A neonate with suprasystemic pulmonary vascular resistance may be found to have a ductus arteriosus when an echocardiogram is performed for systemic arterial desaturation; further investigation may reveal normal saturation in the upper extremities and cyanosis in the lower due to right-to-left shunting across the PDA (i.e., differential cyanosis).
In term infants and children, the most common presentation of PDA in the absence of other congenital heart defects is a murmur. The classic murmur of a restrictive PDA is continuous throughout systole and diastole with systolic accentuation. This makes sense when one considers the relative pressure differences between the aorta and PA throughout the cardiac cycle. Assuming a child with a PDA has a systemic blood pressure of 90/50 mm Hg and a PA pressure of 25/15 mm Hg, the peak systolic pressure gradient is 65 mm Hg, much higher than the peak diastolic pressure gradient of 35 mm Hg. Blood will flow at a faster rate down the higher systolic pressure gradient, producing accentuation of the murmur. Extrapolating from the modified Bernoulli equation (P = 4v2, where P is pressure, v is velocity of flow), the peak systolic velocity would be approximately 4 m/s and the peak diastolic velocity would be close to 3 m/s in the above scenario. This simplified application of the Bernoulli principle is limited, however, because aortic and PA maximum systolic and minimum diastolic pressures are not simultaneous, and because the modified Bernoulli equation does not take into account the length of the PDA.
With the accessibility of high-quality echocardiography, the diagnosis of small, incidental PDAs without audible murmur has become more common. These so-called “silent” PDAs result in a negligible shunt and do not cause left atrial or left ventricular enlargement. The silent ductus presents an interesting management conundrum, which is discussed below.
Rarely, a large PDA may present in older children and adult patients with signs and symptoms of Eisenmenger syndrome—including exercise limitation and cyanosis. These patients may have had limited access to medical care earlier in life. With Eisenmenger syndrome, a typical PDA murmur is absent. If appropriately scrutinized on examination, the pulmonary component of S2 will be accentuated due to elevated PA diastolic pressure. Often, the PDA is incidentally found by echocardiography, CT, MRI, or angiography.
Investigation
Chest Radiograph
The chest x-ray of a patient with a restrictive PDA is often normal or nonspecific. With a larger ductus, there may be pulmonary vascular congestion. Left atrial enlargement may result in inferior
compression of the left mainstem bronchus. For very large PDAs, the cardiac silhouette may be enlarged due to left atrial and left ventricular enlargement.
compression of the left mainstem bronchus. For very large PDAs, the cardiac silhouette may be enlarged due to left atrial and left ventricular enlargement.
Electrocardiogram
Electrocardiographic (ECG) findings are neither sensitive nor specific for PDA. A hemodynamically insignificant PDA will not cause any notable ECG changes. With larger PDAs, the ECG may show widened P waves, reflecting left atrial enlargement. Changes suggestive of left ventricular hypertrophy may be seen in the presence of a significant, chronic PDA shunt, including tall R waves in I, II, II, aVL, V5, or V6 and tall S waves in V1 or V2. Rarely, ischemic ST segment changes have been reported in the presence of significant left-to-right diastolic runoff, resulting in coronary steal. Oxygen supplementation during anesthesia for PDA closure may increase the Qp/Qs and exacerbate coronary hypoperfusion in such cases (40).
Echocardiogram
Echocardiography is the principal imaging modality needed to assess the PDA and its hemodynamic effects. On 2-D echocardiography, the usual left-sided PDA is most consistently seen between the origin of LPA and proximal descending aorta, distal to origin of left subclavian artery (41,42,43,44,45,46). Typical views to assess PDA are parasternal short-axis, high-left parasternal short-axis, and suprasternal sagittal views. From parasternal short-axis view (Fig. 31.2, Video 31.1), the PDA is seen originating from the junction of LPA and MPA, and connecting to the proximal descending aorta. A high-left parasternal view (Fig. 31.3, Video 31.2) is directed in the plane of LPA and angulated to visualize the “trifurcation,” where the MPA appears to divide into three vessels—the RPA, LPA, and PDA. This view is optimal for displaying the complete length of PDA and also provides a good angle for spectral Doppler interrogation.
Color Doppler imaging in these planes can show the direction and relative amount of shunt across a PDA (22,47,48,49). Left-to-right shunt is easily seen as the direction of blood flow from aorta to PA is in opposite direction of the forward flow in the branch PAs and descending aorta. However, a PDA with right-to-left shunt or low-velocity left-to-right shunt can be difficult to visualize by color Doppler. Lowering the Nyquist limit to detect low-velocity shunt is helpful for diagnosis. A PDA that is difficult to visualize, such as small PDA with low-velocity shunt, can be more reliably seen in a sagittal plane in the high-left parasternal position, on a sweep from LPA to descending aorta. Vertical or tortuous PDAs commonly are associated with other congenital heart disease (50).
A “reverse” or vertical PDA is usually seen in cases of pulmonary atresia. This type is best visualized from a suprasternal sagittal view in the plane of aortic arch. The ductus is seen arising from the undersurface of the distal aortic arch. Sometimes the direction of shunt in a tortuous PDA is difficult to assess with color Doppler alone. Pulse wave interrogation can help in clarifying the direction of shunt, especially in case of low-velocity or bidirectional shunting (51,52). The pulse wave Doppler cursor usually is positioned at the PA end of the PDA.
A “reverse” or vertical PDA is usually seen in cases of pulmonary atresia. This type is best visualized from a suprasternal sagittal view in the plane of aortic arch. The ductus is seen arising from the undersurface of the distal aortic arch. Sometimes the direction of shunt in a tortuous PDA is difficult to assess with color Doppler alone. Pulse wave interrogation can help in clarifying the direction of shunt, especially in case of low-velocity or bidirectional shunting (51,52). The pulse wave Doppler cursor usually is positioned at the PA end of the PDA.
Continuous wave Doppler interrogation usually is used to assess the pressure gradient between the aorta and PA, but may be contaminated by blood flow in LPA. Pressure gradient estimation by Doppler echocardiography is not reliable in a tortuous PDA. Pulse wave Doppler assessment is used to assess the hemodynamic significance of a PDA, which is more important than its actual size. The clinical decision of whether to close a PDA is usually made on the basis of its hemodynamic effect. A PDA in a patient with low PA pressure will have continuous left-to-right shunt on color and spectral Doppler assessment (Fig. 31.4). Whereas, in case of elevated PA pressure, the shunt is bidirectional (Fig. 31.5). A hemodynamically significant PDA has continuous diastolic antegrade flow into the LPA. Diastolic run-off from descending aorta can be seen as pan-diastolic flow reversal on pulse wave Doppler interrogation of descending aorta from a suprasternal notch or subcostal view (Fig. 31.6). A large left-to-right shunt also manifests volume overload of left heart structures. Left atrial and left ventricular volumes are increased with increased flow velocities on pulmonary venous Doppler profile.
MRI/CT
Echocardiography alone is almost always adequate for assessing the anatomy and hemodynamic effects of PDA. Computed tomography angiography (CTA) or cardiac magnetic resonance (CMR) imaging can be used in rare situations when echocardiography is limited due to poor acoustic windows or when other anomalies are suspected, such as coarctation of aorta or vascular rings (53). More often, a small PDA is incidentally discovered on a CTA or CMR study performed for a different indication. Visualization of a PDA on CTA or CMR is dependent on the accurate timing of contrast injection to opacify the duct. For optimal opacification of the PDA, the contrast is timed to
the aorta in a patient with low PA pressure (Fig. 31.7). If the contrast is timed to the PA, left-to-right shunting from aorta through PDA creates a filling defect in the proximal LPA. With elevated PA pressure, such as Eisenmenger syndrome, the PDA is best seen when contrast is timed to the PA (Fig. 31.8). Flow quantification by phase contrast imaging in CMR examination is useful in assessing the ratios of pulmonary to systemic blood flow (Qp/Qs).
the aorta in a patient with low PA pressure (Fig. 31.7). If the contrast is timed to the PA, left-to-right shunting from aorta through PDA creates a filling defect in the proximal LPA. With elevated PA pressure, such as Eisenmenger syndrome, the PDA is best seen when contrast is timed to the PA (Fig. 31.8). Flow quantification by phase contrast imaging in CMR examination is useful in assessing the ratios of pulmonary to systemic blood flow (Qp/Qs).
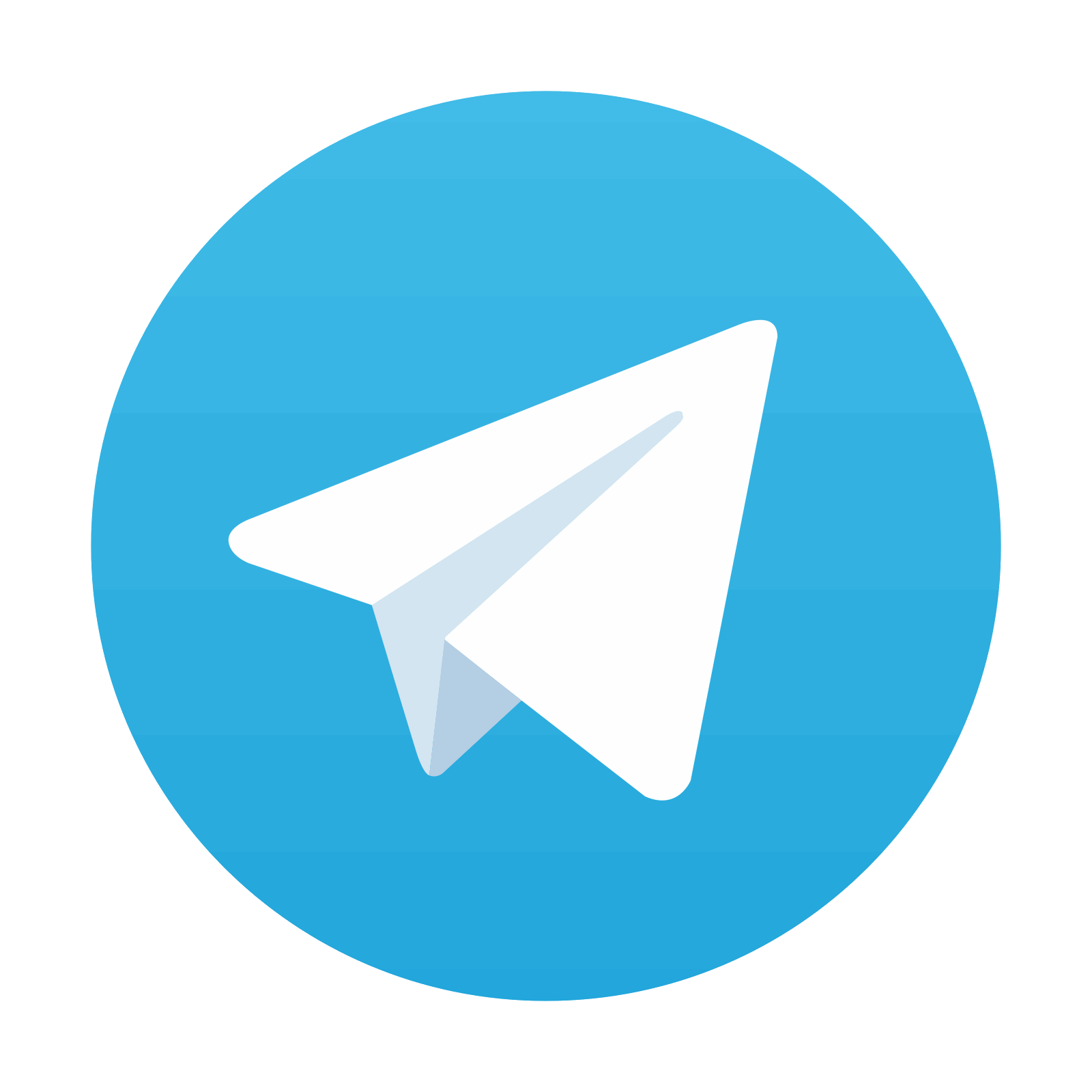
Stay updated, free articles. Join our Telegram channel

Full access? Get Clinical Tree
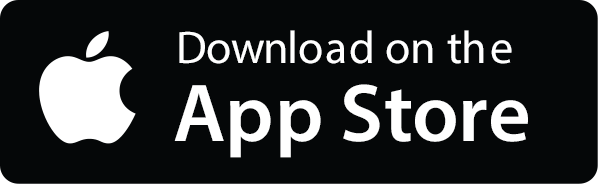
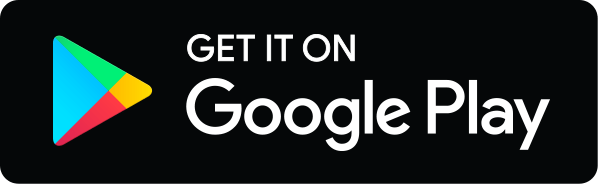
