Flow/volume curves. (a) Patient with mild to moderate expiratory muscular compromise. The initial slope of a slow ascent is observed. The maximum expiratory flow (MEF) does not show a defined peak. An abrupt end of the expiration is apparent. (b) Patient with moderate to severe muscular compromise. There is a flattening of the initial phase of the expiratory phase and of the inspiratory phase of the flow/volume loop. (c) Transient fluxes during the expiratory phase of the flow/volume curve, determined by coughing in a healthy subject. Its absence indicates expiratory muscle compromise
An effective cough that efficiently removes secretions from the airway requires sufficient intrathoracic pressure to produce dynamic compression of the airway and respiratory flow at a high velocity. The expiratory flow/volume loop also serves to show the effect of coughing. In normal subjects, coughing appears as transitory flows that exceed the maximum flow achieved by a forced expiration, with spikes that rise above the loop. The absence of these spikes indicates respiratory muscular weakness and correlates very well with a maximum respiratory pressure of <40 cm H2O (Fig. 10.1c).
Pulmonary Volume and Capacity
Spirometry Measurements: Volumes and Flows of Forced Expirations
The basic examination assesses respiratory mechanics and is used in patients with NMDs to establish the degree of respiratory muscular compromise through analysis of the shape of the inspiratory and expiratory flow/volume loops (described above) and their variables, the most useful being the forced vital capacity (FVC) test, which measures inspiratory and expiratory musculature at the time. A normal score indicates the absence of significant muscular compromise. However, it is not a sensitive indicator as it does not decrease until muscular force is highly compromised. It is mainly used to follow the evolution of patients as a marker of the risk of respiratory insufficiency and mortality. Thus, FVC of <1 liter predicts an 8% probability of 5-year survival in patients with Duchenne muscular dystrophy.
Another useful spirometry technique to identify diaphragmatic compromise is performance of the test with the patient first sitting or standing and then in the prone position. A 25% or greater decrease in FVC in the supine position indicates altered diaphragm functioning that is not able to overcome the gravitational force of the abdominal content. On the other hand, if the patient has an obstructive disease of the small intrathoracic airway (asthma, postviral damage, or other disease), measurements of forced expiratory flow (FEF) (e.g., FEF at 25–75% of FVC (FEF25–75) and FEF at 50% of FVC (FEF50)) help to determine the presence of this associated compromise.
Measurement of Static Pulmonary Volume
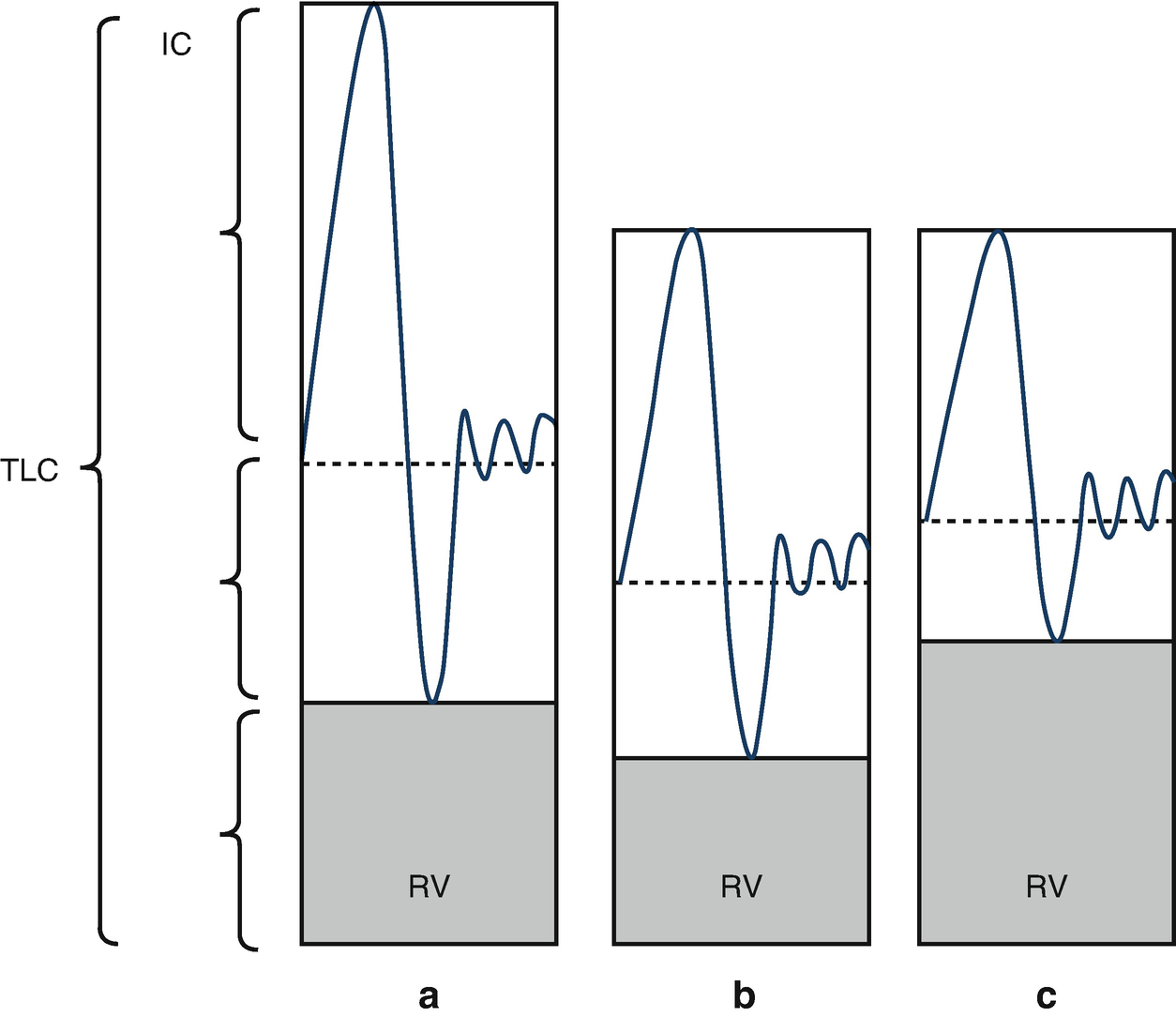
Lung volumes. (a) Normal. (b) Restrictive lung disease, showing proportional decreases in all volumes. (c) Restrictive lung disease with air trapping, showing a proportional increase in the residual volume, due to the lower expiratory reserve volume and the decrease in inspiratory capacity
Maximum Voluntary Ventilation
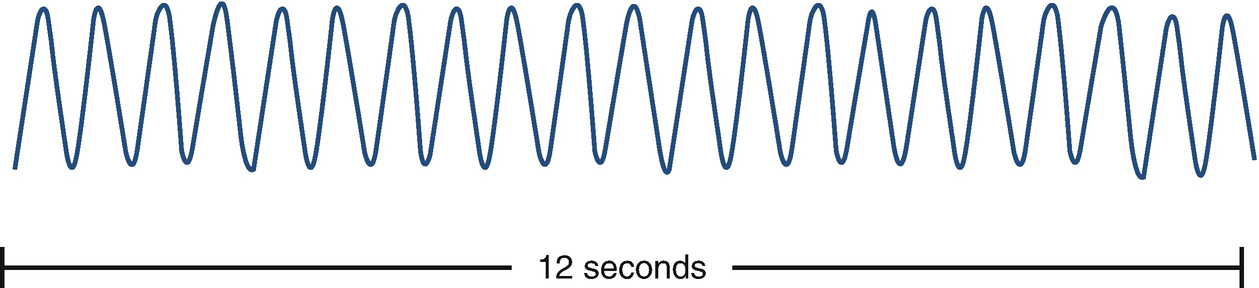
Maximum voluntary ventilation measurement. The outline is homogeneous, with the end of expiration at a constant level
Arterial Gases and Saturometry
The main purpose of arterial gas measurement is detection of hypercapnia secondary to hypoventilation. This appears initially in patients with slow and progressive compromise during sleep; consequently, the sample should be taken upon awakening. Samples taken when patents are fully awake can underestimate the severity of the alteration of gaseous exchange.
In patients who have moderate to severe muscular compromise, continuous measurement of peripheral oxygen saturation (SpO2) during sleep shows frequent falls due to apnea and/or central and/or obstructive hypopnea. This measurement correlates very well with the vital capacity. More details can be found in the chapter on pulse oximetry in this book. Polysomnography is the test of choice for diagnosing sleep disorders, with the capacity to register SpO2 and transcutaneous CO2 tension.
Ventilation Control: Measurement of Occlusion Pressure
Respiratory control depends on the partial pressures of carbon dioxide (pCO2), and of oxygen (pO2), the pH chemoreceptors in the neural centers and paths, and the motor action of the respiratory muscles. A progressive increase in pCO2 is observed in patients with NMDs, which may be caused by alveolar hypoventilation and/or failure to control respiration. The latter has been described in myotonic dystrophy and other congenital myopathies. Measurement of occlusion pressure in the mouth in the first 100 milliseconds of inspiration (P0.1) allows estimation of the response to CO2 retention. This is the pressure generated in the airway by contraction of the inspiratory muscles when the airway is occluded at the end of an expiration at rest (the functional residual capacity (FRC)). FRC is the pressure generated in the first 100 milliseconds of inspiration, during which the conscious response does not interfere with the occlusion or the mechanical properties of the lung. However, it depends on the contractile state of the respiratory musculature and on the FRC variation in the subject. FRC can be measured in infants while they are sleeping and in older children when they are capable of breathing peacefully with a nasal clamp and a mouthpiece for at least 5 minutes, with occlusion occurring every minute until five acceptable measurements have been obtained.
The main purpose is assessment of respiratory control in stable patients with an increased respiratory load due to bronchopulmonary dysplasia, cystic fibrosis, bronchiolitis obliterans, or another cause. The normal value in adults is 1 cm H2O, and under normal conditions of stability, P0.1 increases by approximately 3 cm H2O.
Functional Capacity: 6-Minute Walk Test
There are several ways to objectively study functional exercise capacity. The 6-minute walk test is considered the easiest to undertake, the easiest for the patient to tolerate, and the test that most reflects tolerance of regular activities. The test measures the distance the patient can cover when walking as rapidly as possible for 6 minutes. It assesses, in an integral manner, all of the systems involved in the exercise: pulmonary, cardiovascular, circulatory, blood, neuromuscular, muscular, and metabolic.
Because of their base condition, patients with NMDs have difficulty doing the test. The main purpose of the test in patients with chronic pulmonary disease is a checkup for respiratory rehabilitation.
The test requires a 30 m walkway with a smooth hard surface. A marker should be placed every 3 m, and turning points should be marked with a cone. The heart rate and SpO2 are assessed before and after the exercise. When continuous monitoring is required, the patient should carry the equipment, which needs to be light enough not to hinder the patient’s performance. The degree of basal and post-test dyspnea is measured on the modified Borg scale. If the patient requires continuous oxygen support, the system should not interfere with the walking circuit. Ongoing encouragement by the technician is important, drawing on the chronology and standard phrases. It has been observed that longer walk distances are achieved with encouragement.
There are several reasons for obtaining different results in the test performance. Factors that reduce the distance walked are smaller body size, obesity, failure to understand the technique, and a walkway of <30 m, given that this implies more turning. The most important factor in increasing the walk distance is the motivation of the patient.
The most reliable way to determine the clinical improvement of a patient on the basis of this test after some form of intervention is to conduct two or three pre- and post-treatment tests (thus ensuring the reproducibility of the test), using the same technician to ensure the same methodology.
In relation to Chilean reference values, we use those described by Gatica et al. (2002), which are described in greater detail in the chapter on respiratory rehabilitation in this book.
Muscular Strength
Muscular strength in the respiratory system is estimated as the pressure generated by muscular contraction. However, this relationship is complex and depends on the mechanical characteristics of the rib cage and the abdominal wall. Consequently, it is preferable to interpret the results of these measurements as a global index of respiratory musculature performance.
Static Pressures: Maximum Inspiratory and Expiratory Pressures
Tests that measure the maximum inspiratory pressure (MIP) and maximum expiratory pressure (MEP) are most commonly used to assess muscular strength, given that the MIP test is more sensitive than vital capacity measurements at the initial stage of the disease. The MIP is the maximum pressure generated during inhalation with the airway occluded and is based on RV. MEP is the maximum pressure generated during exhalation with the airway occluded and is based on TLC. Measurement of MIP assesses the functioning of the inspiratory musculature: the diaphragm and the external intercostal and accessory muscles (the scalenes and sternocleidomastoids). The abdominal and internal intercostal expiratory muscles are assessed with MEP.
These techniques measure the pressure generated by the respiratory muscles plus the pressure of the elastic retraction of the rib cage and the lungs. Consequently, it should be keep in mind that measurement of MIP on the basis of RV can increase the latter by up to −30 cm H2O. Similarly, to measure MEP on the basis of TLC, the contribution can be up to +40 cm H2O. Some specialists argue that, realistically, in MIP and MEP measurements in chronic respiratory failure (CRF)—that is, with elastic retraction at zero—the pressures measured in the mouth reflect only the pressure that is generated by muscular contraction.
For the measurement, a nasal clamp should be placed on the patient, and a cylindrical mouth piece is recommended. Although some authors have used scuba diving–type masks to obtain reference values, it is recommended that this be based on theoretical values for the same method used on the patient. The mouthpiece should have an escape 1 mm in diameter to avoid glottic closure and an artificially elevated MIP, and also to reduce the use of the mouth musculature during the maneuver for MEP.
The force should be maintained for a minimum of 1.5 seconds; at least five maneuvers should be conducted until at least two repeatable results are obtained (with a maximum variation of 10% between the two best values), and the better one should be chosen. A MIP value under −80 cm H2O is of great value for ruling out diaphragmatic compromise.
The disadvantages of these measurements are their high degree of variability and the fact that they reflect the effect of learning. Consequently, it is advisable to do more than five maneuvers in patients who do not achieve an adequate technique, until two repeatable results are obtained. The analysis of the values obtained will be aided by inclusion of the FVC value, given that the pressures the patient can generate depend significantly on this. A MIP value of less than −25 cm H2O suggests a risk of respiratory failure. This indicates the importance of serial measurements of pulmonary volumes and static pressure.
It is recommended to use the reference values provided by Szeinberg et al. with schoolchildren and adolescents. MIP and MEP values can be measured in infants when they cry, with the mask placed over the infant’s mouth. The mouthpiece should have an escape to avoid glottic closure. The airway should be occluded at the end and at the beginning of the crying effort to measure MEP and MIP, respectively.
Nasal Sniff Test
Sniffing is a natural action, which many patients find easier than performing a maneuver for measuring static pressure. It is a reproducible method, validated in children, and used to assess global inspiratory muscular function. Measurement of MIP has the advantage of being an easily applied technique that avoids the problem of air loss through a mouthpiece, as often occurs in patients with neuromuscular illnesses. It consists of a short, rapid, voluntary inhalation through the nose and with the mouth closed, beginning after an exhalation at rest (FRC). One of the patient’s nostrils is sealed with a rubber or sponge mold, into which a catheter is inserted. The patient breathes through the nostril that is not blocked. The catheter measures the nasopharyngeal pressure, which is a reasonable indicator of the intra-alveolar pressure. The values are generally lower than the MIP value because there is less shortening of the inspiratory musculature.
As with measurement of static pressure, a limitation is the dependence on effort. Most children over 4 years of age are capable of performing the maneuver adequately. There should be a 30-second lapse between maneuvers. If it is difficult to obtain reliable data in child subjects, it is recommended to make more than ten attempts, given the important effect of learning. The values tend to be underestimated in patients with intra- or extrathoracic airway obstruction.
Pressure values greater than −70 cm H2O and −60 cm H2O are considered acceptable for males and females, respectively.
Peak Cough Flow
Coughing is a basic defense mechanism to eliminate secretions from the airway. One or more coughing phases are affected in patients with NMDs: maximum inspiration, opening or closing of the glottis, keeping the glottis open or closed while increasing intrathoracic pressure, and opening the glottis together with forced expiration. Coughing can be assessed objectively by measuring the peak cough flow (PCF) or MEF generated while the subject is coughing. A nasal clamp is placed on the patient, who is asked to take a deep breath and then cough into the flow meter. The maneuver should be done at least five times, taking care to ensure that the mouthpiece remains well sealed. The normal MEF is above 300 l/min. A value of less than 160 l/min is associated with difficulty in eliminating secretions and a consequent risk of respiratory complications such as pneumonia, atelectasis, or respiratory insufficiency. If the reading is between 160 and 270 l/min, it is likely it will fall below 160 l/min in the context of a respiratory infection.
Invasive Methods: Transdiaphragmatic Pressure
