Dariush Mozaffarian
Nutrition and Cardiovascular and Metabolic Diseases
Together with smoking and physical activity, dietary habits constitute the foundation for causation, prevention, and treatment of most cardiometabolic diseases, including coronary heart disease (CHD), stroke, type 2 diabetes mellitus (DM), sudden cardiac death, atrial fibrillation, heart failure, and vascular cognitive decline. In 2010, 8 of the top 25 modifiable causes of all global morbidity and mortality, expressed as disability-adjusted life-years, were dietary, including insufficient intake of fruits, nuts, whole grains, vegetables, seafood, omega-3 fatty acids, and dietary fiber and excess intake of salt and processed meats.1 The global burden from suboptimal dietary habits has increased substantially in recent decades because of rapid social, cultural, and environmental transitions transmitted primarily through changes in diet and other lifestyle-related behavior.1 Familiarity with evidence of the beneficial and harmful effects of specific dietary factors is essential to prioritize interventions for individual patients and for populations and to reduce the tremendous disease burden caused by suboptimal diets.
The science of nutrition and chronic diseases has progressed rapidly in recent years. Whereas previous dietary guidance was derived largely from ecologic studies, short-term experiments, and animal studies, nutritional science has been transformed by more robust evidence from prospective cohorts and randomized trials of cardiovascular disease (CVD) endpoints and well-conducted metabolic trials of multiple risk markers and pathways. One key lesson learned is that dietary habits influence virtually all established and emerging risk factors, including blood pressure (BP), glucose-insulin homeostasis, lipoprotein concentration and composition, weight gain, inflammation, endothelial function, and cardiac function and arrhythmia. Consequently, the effects of a dietary factor on disease risk should never be inferred only from its effects on any single risk factor, such as blood cholesterol concentrations.2 For several diet-disease relationships, robust concordant evidence is now available across many different research paradigms. A second key lesson is the importance of foods and overall dietary patterns, rather than single isolated nutrients, for treating and preventing cardiometabolic diseases. This chapter reviews dietary factors with the strongest evidence of cardiometabolic effects and highlights key knowledge gaps. Because translation of knowledge into action is essential, this chapter also reviews effective individual- and population-based strategies for changes in behavior.
Foods
In the early and mid-20th century, nutritional science and dietary guidelines concentrated mainly on diseases caused by deficiency of nutrients, which resulted in corresponding emphasis being placed on isolated single nutrients to prevent disease.3 When nutritional focus subsequently shifted to chronic diseases, this emphasis on single nutrients nonetheless lingered. With few exceptions, however, single nutrients in isolation have little effect on cardiometabolic diseases. More relevant is the influence of foods and dietary patterns, a complex matrix of fatty acids, proteins, carbohydrate quality, micronutrients, and phytochemicals that together modify cardiometabolic risk.3,4 A focus on foods rather than single nutrients also facilitates dietary guidance and change in behavior.
Fruits and Vegetables
Higher fruit and vegetable intake is consistently associated with a lower incidence of CHD and stroke (Fig. 46-1).5 Risk for DM involves similar, though not statistically significant trends, perhaps because of stronger associations of certain subtypes such as green leafy vegetables.6 In controlled trials lasting up to 2 years, diets with an emphasis on consuming fruits and vegetables substantially improve multiple cardiometabolic risk factors, including BP, lipid levels, insulin resistance, inflammation, adiposity, and endothelial function.7 In trials, the benefits of fruit and vegetable intake cannot be reproduced with equivalent amounts of potassium, magnesium, and fiber supplements8 and are largely independent of the macronutrient (fat, protein, or carbohydrate) content of the diet.9 This observation suggests that the benefits derive from a more complex set of micronutrients, phytochemicals, and fiber in fruits and vegetables, as well as from the replacement of less healthful foods. Together, these studies provide convincing evidence that fruit and vegetable consumption each lowers risk for CVD; notably, fruits and vegetables have similar overall effect sizes. The benefits of specific subtypes of fruits and vegetables, as well as 100% juice, require further study.
Whole Grains, Refined Grains, Starches, and Sweets
Improved understanding of how different carbohydrate-rich foods, which comprise about half of all calories in most diets, influence cardiometabolic risk represents a major advance in nutritional science. Although carbohydrates have traditionally been classified as simple (e.g., sugars such as glucose, fructose, galactose, sucrose, lactose, and lactulose) or complex (e.g., starches in grains and potatoes such as glycogen, cellulose, and hemicellulose), this distinction has little cardiometabolic relevance; simple sugars and refined carbohydrates or starches are each rapidly digested and absorbed after ingestion. Other characteristics more accurately define carbohydrate quality, including dietary fiber content, whole grain content, glycemic index (GI) and glycemic load (GL), and food structure (liquid versus solid).7 Even though total carbohydrate intake is not strongly associated with CVD, these interrelated characteristics of carbohydrate-rich foods have important effects on cardiometabolic risk (Fig. 46-2). The effects of dietary fiber and GI/GL, each determined by overall dietary patterns, including intake of whole grains, refined grains, starches, sweets, fruits, vegetables, nuts, seeds, and legumes, are reviewed later (see Carbohydrates).
The content of whole grain also appears to be relevant. Whole grains include endosperm, bran, and germ; the latter are stripped away during processing to produce refined grains, in which only the starch-rich endosperm remains. Bran provides fiber, B vitamins, minerals, flavonoids, and tocopherols, whereas germ provides fatty acids, antioxidants, and phytochemicals. Whole grain intake is consistently associated with lower risk for CVD, DM, and weight gain (see Fig. 46-1).10,11 In trials, whole grain intake improves glucose-insulin homeostasis, low-density lipoprotein cholesterol (LDL-C), and possibly endothelial vasodilator function and inflammation.11 As with fruits and vegetables, no single nutrient appears to account for these benefits, which may arise from multiple synergistic effects.4 Food structure is also relevant: carbohydrate-rich foods in liquid form, such as sugar-sweetened beverages (SSBs), are associated with even greater weight gain than equivalent solid foods are,10 probably related to less satiation when consuming liquid carbohydrates.
In contrast to whole grains, refined grains (e.g., white bread, rice, most breakfast cereals), starches (e.g., potatoes), and sweets are associated with greater weight gain,10 are a major determinant of dietary GI and GL, and are associated with greater risk for CHD and DM (Fig. 46-3). These effects are probably due to both direct metabolic harm (e.g., on postprandial glucose-insulin, endothelial, and inflammatory responses) and displacement of healthier foods (e.g., whole grains, fruits, vegetables). Additionally, harm may be greatest in those predisposed to insulin resistance, such as persons with DM, lower physical activity, or greater adiposity. Based on their prevalence in most diets, reducing the intake of refined grains, starches, and sugars, with replacement by whole grains, fruits, vegetables, and other healthier foods, is a major dietary priority. Because several interrelated factors influence their effects on health (see Fig. 46-2), no single accepted criterion exists for identifying healthful carbohydrate-rich foods. Choosing grain foods with a ratio of total carbohydrate to dietary fiber (grams per serving) of less than 10:1 appears to identify the most healthful grain choices better than other recommended criteria do.12
Nuts, Seeds, and Beans
Nut consumption has been associated with a lower incidence of CHD and DM in prospective cohorts (see Fig. 46-1)5,13,14; with lower LDL-C and some evidence of improved oxidative, inflammatory, and endothelial responses in observational studies and controlled trials15–17; and with less long-term weight gain and lower body mass index in observational and some interventional studies.10,18 Potentially bioactive constituents include unsaturated fats, vegetable protein, fiber, folate, minerals, tocopherols, and phenolic compounds. The effects of different types of nuts require further study, but the benefits in short-term trials, the magnitude and consistency of lower risk in observational studies, and the large predicted disease burden because of low population intake1 support an emphasis on modest nut consumption for lowering cardiometabolic risk.
The cardiovascular effects of seeds and beans (legumes) are less well established. In a limited number of cohorts, legume intake was inversely associated with CHD, but with not diabetes or stroke.14 Meta-analyses of small trials of soy foods suggest modest improvement in blood cholesterol levels, especially in diabetic patients, and small to no effects on multiple other risk factors, including glycemic control, BP, inflammation, and body weight, though with heterogeneity and occasional positive findings in some post hoc patient subgroups.19–23 Both seeds and legumes provide an overall package of micronutrients, phytochemicals, and fiber that could reduce cardiometabolic risk; this hypothesis requires further evaluation in controlled interventions and long-term cohorts.
Fish
Numerous prospective cohorts have reported on fish intake and CVD (see Fig. 46-1). Modest fish consumption is consistently associated with less fatal CHD, but only small or no association with total CHD or nonfatal myocardial infarction (MI).24,25 Meta-analyses of randomized trials of fish oil supplementation are consistent with these findings and demonstrate reductions in CHD mortality but not in other subtypes of CVD.26 The evidence suggests a nonlinear benefit: modest consumption (~2 servings per week) significantly reduces risk versus no consumption, whereas higher consumption does not have appreciable further effects.25
In observational studies, fish consumption has been associated with less ischemic stroke, but fish oil supplements have not been found to influence stroke in post hoc analyses of CHD trials.26,27 A few observational studies have evaluated other CVD outcomes, such as atrial fibrillation and heart failure, with mixed findings.24 Many studies have evaluated incident DM, which is not associated with either overall fish consumption or circulating omega-3 biomarkers, although inverse associations are seen in Asian populations.28,29
The types of fish consumed and the preparation methods may influence omega-3 blood levels and effects on CVD, with the greatest benefit being derived from nonfried oily (dark meat) fish, which contain up to 10-fold more omega-3 fatty acids than other species do.24 Fish contains omega-3 and other unsaturated fats, selenium, and vitamin D. Prevention of death in patients with CHD appears to be related mainly to the omega-3 content; the health effects of omega-3 fatty acids are reviewed later (see Macronutrients). Methylmercury in fish has no detectable influence on CVD events or incident hypertension.30,31
Meat
Although ingesting lean meat lowers saturated fat and cholesterol, the effects of meat consumption on cardiometabolic risk appear to be more complex, with other factors (e.g., sodium, heme iron) potentially being more relevant. The evidence available suggests that processed meat (i.e., meat preserved with sodium or other preservatives, such as deli meats, sausage, and hot dogs) increases the risk for both CHD and DM whereas unprocessed red meat has small or no effects on CHD but increases the risk for DM, though to a lesser extent than processed meat does.32 These divergent associations are seen despite relatively similar average amounts of saturated fat and cholesterol in processed and unprocessed red meat.33 Conversely, differences in sodium content (≈400% higher in processed meat) appear to account for about two thirds of this difference in the observed incidence of CHD.32 These findings, together with other evidence of little to no overall effect of saturated fat consumption on CHD or diabetes (see Macronutrients), suggest that the constituents most relevant to cardiometabolic effects may include dietary cholesterol, heme iron, and nitrates with respect to risk for DM, and sodium and nitrates with respect to risk for CHD.32 This evidence implies that clinical and public health guidance for achieving cardiometabolic health should especially prioritize reduced ingestion of processed meats, as well as lowering the content of sodium and other preservatives. Thus, promotion of processed deli meat sandwiches (e.g., low-fat processed chicken, turkey, or bologna) as “healthy” alternatives on the basis of lower total fat and saturated fat is unjustified. The few studies reporting on meat intake and incident stroke observed relatively small positive associations,34 which limits strong conclusions on this endpoint.
Dairy
Dairy foods constitute a major portion of many diets, yet their cardiometabolic effects remain remarkably understudied. In randomized trials, milk or dairy intake has been found to reduce body fat and increase lean mass in the setting of energy-restricted diets and to have little effect on body weight or composition in ad libitum diets.35,36 Long-term prospective cohort studies support the latter findings and have not observed any relationship of whole-fat or reduced-fat/nonfat dairy with long-term weight gain, except for an inverse association of yogurt, which requires further study.10 Multicomponent dietary trials, including low-fat dairy products, have shown improvements in BP, lipid levels, insulin resistance, and endothelial function,7 but such designs cannot extricate the specific effects of dairy products. Long-term cohort studies have found dairy consumption to be associated with a modestly lower risk for CHD, stroke, and DM (see Fig. 46-1), as well as a lower risk for metabolic syndrome or its components.37–40
The relevant active constituents in dairy foods remain unclear. In short-term interventions, calcium and linoleic acid (LA) have produced small or no effects on risk factors for CVD. Some observational studies suggest that dairy fat or potentially bioactive dairy fatty acids may have benefit.40–42 Fermentation (i.e., the presence of active bacterial cultures) may also be relevant given growing recognition of the importance of the gut microbiome for health.43 Overall, the data support guidelines for modest dairy consumption (two to three servings per day), but the active ingredients have not been defined sufficiently by current evidence to allow strong recommendations on choosing between whole-fat or low-fat dairy or between subtypes (e.g., milk, cheese, yogurt) for reducing CVD, DM, or adiposity.
Beverages
Sugar-Sweetened Beverages
Ecologic data, prospective cohorts, and randomized trials together provide convincing evidence that SSB intake increases adiposity. In the United States, calories from beverages increased from 11.8% to 21.0% of all calories consumed between 1965 and 2002—an increase of 222 kcal per person per day, largely because of SSBs (sodas, energy drinks, sweetened ice teas, fruit drinks).44 The average American teenage boy and girl consume 18 and 14 8-oz servings, respectively, of SSBs weekly45; most consumption by youth occurs at home.46 Per serving, SSBs are more strongly associated with long-term weight gain than nearly any other dietary factor.10 Randomized trials have confirmed that reducing SSB intake decreases weight gain and fat accumulation.47–49 Calories in liquid form, versus solid foods, appear to be less satiating and increase the total calories consumed.50 SSB intake is also associated with a significantly higher risk for DM and metabolic syndrome,51 probably related to both weight gain and the independent harm of a high GL.
Milk
See Dairy.
Coffee and Tea
Although coffee and tea are often considered in terms of caffeine, they each represent liquid extracts of plants (coffee beans, tea leaves) containing many other compounds. Frequent coffee intake (three to four or more cups per day) in particular is associated with lower insulin resistance and risk for DM (see Fig. 46-1), unrelated to its caffeine content.52,53 Most such data are observational, however. Acutely, caffeinated coffee worsens BP, insulin resistance, and glucose intolerance.54,55 Yet long-term coffee intake does not raise BP or insulin resistance, thus suggesting tachyphylaxis and/or other partly offsetting factors.52,56,57 In prospective observational studies, coffee intake was not associated with CHD and was weakly associated with stroke.58,59 In a limited number of studies, coffee intake has been associated with less incident heart failure.60 Drinking unfiltered coffee raises LDL-C; intake of instant or paper-filtered coffee has little effect.52
Intake of black tea is not significantly associated with CHD, whereas intake of green tea is associated with lower risk, but this is based on few studies (Fig. 46-1).61 Frequent tea drinking (three or more cups per day) is associated with a modestly lower risk for stroke and DM.53,62 In short-term trials, green tea was found to lower LDL-C63 and minimally affect weight loss or maintenance64; fewer data are available on BP or endothelial function.65 In one 6-month trial, three cups per day of black tea modestly reduced mean ambulatory systolic (−2 mm Hg) and diastolic (−2.1 mm Hg) BP at 6 months in comparison to controls, who abstained from regular tea drinking.66 Overall, observational evidence supports the benefits of frequent coffee intake on incident DM, but little effect on CHD or stroke, whereas more mixed observations suggest possible benefits of frequent tea intake on DM and stroke. Strong conclusions on the cardiometabolic effects of coffee or tea will require further investigation, including both physiologic and clinical endpoint trials.
Alcohol
Habitual heavy alcohol consumption causes up to a third of cases of nonischemic dilated cardiomyopathy in many nations.67 The ventricular dysfunction is often irreversible, even when alcohol use is stopped; continued drinking is associated with high mortality (see also Chapter 68). Habitual alcohol intake, as well as acute binges, is associated with a higher risk for atrial fibrillation.68–70 Like other liquid calories (except milk), alcohol intake is also associated with higher long-term weight gain.10 Conversely, when compared with nondrinkers, regular moderate consumption—up to about 2 drinks per day for men and around 1 to 1.5 drinks per day for women—is associated with a lower incidence of CHD and DM, but not stroke.71,72 Such observational analyses, however, could overestimate the benefits because of nondrinkers comprising individuals who had quit or never used alcohol as a result of poor health.73 Yet the magnitude and consistency of the lower risk observed across diverse populations, together with favorable effects on high-density lipoprotein cholesterol (HDL-C), insulin resistance, and fibrinogen in controlled trials,74 provide strong evidence for at least some cardiometabolic benefit of moderate alcohol intake. The health effects are due to the alcohol content; different drinks (e.g., red or white wine, beer, spirits) have similar cardiometabolic effects. Conversely, the drinking pattern is important: greater benefit is seen with regular moderate drinking than with irregular or binge drinking.75 Because of alcohol-related cancers, liver disease, cardiomyopathy, accidents, homicides, and suicides, alcohol use produces major net adverse population health effects.1,76 Thus, alcohol use should not be advised as a means of reducing risk for CVD; adults who already use alcohol should drink moderately.
Macronutrients
Carbohydrates
Although total carbohydrate intake is not strongly associated with risk for CHD (see Fig. 46-3), the quality of carbohydrate-rich foods consumed does influence metabolic health. The effects of whole grains versus refined grains, starches, and sweets are discussed earlier in this chapter.
Dietary Fiber
Dietary fiber consists of nondigestible polysaccharides, naturally occurring resistant starches and oligosaccharides, and lignins in plants, including whole grains, fruits, vegetables, nuts, seeds, and legumes. Higher fiber intake reduces multiple risk factors, including serum triglycerides (TGs), LDL-C, blood glucose, and BP.77 Few long-term trials have been performed; in the Diet and Reinfarction Trial among men with previous MI, advice to consume foods higher in cereal fiber had no significant effect on CHD endpoints, but follow-up was limited to 2 years. Dietary fiber intake is inversely associated with CHD13; however, several studies have observed heterogeneity in this relationship, depending on the fiber type (soluble, insoluble), source (e.g., fruits, vegetables, cereals), or participant characteristics (e.g., sex, smoking status), thus raising questions about the consistency and magnitude of the effect of dietary fiber itself.77 The lower risk observed with fiber-rich foods is probably at least partly due to other beneficial compounds in these foods and/or substitution effects (i.e., replacement of more processed, less healthful foods). Thus, consumption of fiber-rich foods in place of refined grains and processed foods, rather than focusing on dietary fiber per se, is a sensible strategy for lowering cardiometabolic risk.
Glycemic Response
The GI quantifies the total postprandial rise in blood glucose (area under the curve) following ingestion of a carbohydrate-rich food.78 The postprandial rise is standardized to that of a standard carbohydrate (e.g., white bread), calculated as a proportion (percentage) of the standard response times 100. Thus, foods with a GI of 100 induce the same postprandial glycemia as white bread does; foods with a GI of 50 induce half. A principal determinant is the extent of processing: disruption of the natural grain structure exposes the endosperm to digestive enzymes and accelerates absorption. Even high-fiber, whole grain foods (e.g., instant oats, most whole grain breads) can have a relatively high GI because of extensive milling and processing in comparison to less processed counterparts (e.g., steel-cut oats, stone-ground bread) (see Fig. 46-1). Thus, the GI provides a measure of carbohydrate quality independent of fiber or whole grain content. More processed and starchy foods have a higher GI (e.g., white bagel, 99; white rice, 103; whole wheat bread, 106; Gatorade, 111; corn flakes, 115; instant oats, 114; baked potato, 123), whereas less processed foods have a lower GI (e.g., stone-ground bread, 85; steel-cut oats, 74; apples, 56; milk, 45; lentils, 41; mixed nuts, 34).79
The GI compares a fixed amount of carbohydrate (50 g) in each food, but certain foods contain very different amounts of carbohydrate. To account for this, the GL is derived by multiplying the GI by the usual amount of carbohydrate in each food (GL = GI × g per serving of carbohydrate). This distinction is crucial for fruits, which have a low absolute carbohydrate content. For example, the GI of watermelon and white rice is similar (103 per 50 g carbohydrate), but the GL of watermelon is much lower (4 versus 29, respectively).79 Postprandial glycemia correlates with insulin and related counterregulatory responses,78 thus making the GL a reasonable measure of the postprandial metabolic effects of carbohydrate-rich foods.
When compared with foods and diets with a higher GI/GL, those with a lower GI/GL improve blood glucose control, TGs, and LDL-C, as well as perhaps inflammation, endothelial function, and fibrinolysis.78,80–83 Acutely, meals with a lower GI increase satiety84; in some but not all short-term trials, lower GI/GL diets improve weight loss,85,86 and in long-term observational studies, foods with a high GL are strongly linked to weight gain.10 Remarkably, the GL may influence not only caloric intake but also energy expenditure. In a controlled feeding trial, diets with a higher GL led to greater declines in resting and total metabolic expenditure than did diets with a lower GL after weight loss.87 Consistent with these adverse effects, diets with a higher GL have been associated with incident CHD, stroke, and DM in prospective studies (see Fig. 46-3).88–90
Fats
Total Dietary Fat Versus Fat Quality
Early ecologic (cross-national) studies suggested that higher fat intake might increase cardiometabolic risk, but more robust evidence from prospective cohorts and randomized trials has convincingly established that the proportion of energy consumed from fat has negligible effects on CHD or DM (see Fig. 46-3).13,91,92 Similarly, the percentage of total fat in foods or diets has little influence on weight loss, weight gain, or overweight/obesity (see Energy Balance). Lowering total fat intake variably alters blood lipid levels, depending on the specific subtypes of fat in the diet.93 When total fat is reduced, carbohydrate intake typically increases, which can induce adverse effects if the carbohydrates are more refined and of lower quality (see earlier). Some prospective studies suggest that low fat intake might increase the risk for stroke,93 potentially related to low dietary cholesterol and vascular fragility.
In contrast to the limited relevance of total fat, the quality of dietary fat—that is, the specific types of fat and fatty acids consumed—has major effects on health. Dietary fats are traditionally classified into broad categories based on the numbers (e.g., saturated, monounsaturated, polyunsaturated) and positions (e.g., omega-3 [n-3], omega-6 [n-6]) of double bonds (see also Chapter 45). These broad groupings can obscure differences in dietary sources and the biologic effects of individual fatty acids within each class, which may exert specific influences on gene transcription, cell membrane fluidity and receptor function, and lipid metabolites. This chapter follows the conventional categories but highlights the effects of individual lipids when sufficient data exist.
Saturated Fatty Acids
Meats, dairy products, and tropical oils (e.g., palm, coconut) are major sources of saturated fatty acids (SFAs). Based on ecologic comparisons, effects on LDL-C, and animal experiments, SFA intake increases risk for CHD. Yet the SFA story is not so simple.93 For instance, lauric (12:0), myristic (14:0), and palmitic (16:0) acids raise LDL-C relative to carbohydrate, but stearic acid (18:0) does not. Furthermore, even though the 12-, 14-, and 16-carbon SFAs raise LDL-C, they also each lower TGs, raise HDL-C, and increase apolipoprotein A-I (apo A-I) levels. When consumed in place of monounsaturated fatty acids (MUFAs) or carbohydrates, SFAs also lower lipoprotein(a).94 Given these complex, divergent lipoprotein changes, a more global lipid risk marker, such as the total/HDL-C ratio, may be most informative and also correlates best with findings from prospective cohorts and clinical trials of disease endpoints.95 When compared with carbohydrate, the total/HDL-C ratio is not altered by 14 : 0 or 16 : 0 SFA, is nonsignificantly decreased by 18 : 0 SFA, and is significantly decreased by 12 : 0 SFA.93 These changes would predict minimal overall effects of higher SFA intake on CHD.
Prospective cohorts and clinical trials support these findings. In one large trial targeting fat reduction, SFA intake was reduced from approximately 12.5% to 9% energy (E), largely replaced with carbohydrates, without effects on incident CHD (relative risk [RR], 0.98), stroke (RR, 1.02), or diabetes (RR, 0.96).91,92 Similarly, in prospective cohorts, people consuming the highest amounts of SFA have a similar incidence of CVD as those consuming the lowest amounts (see Fig. 46-3).13,96,97
Together, the results of lipid biomarker trials, prospective cohorts of CVD endpoints, and large clinical trials suggest that overall SFA intake minimally affects risk. Yet this absence of overall effect obscures the heterogeneity of individual SFAs, depending on the comparison nutrient and possibly the food source and subtype of SFA. Most notably, multiple research approaches have demonstrated that replacing SFAs with polyunsaturated fatty acids (PUFAs) lowers the risk for CHD (see Fig. 46-3) (see Polyunsaturated Fatty Acids, later).95 Conversely, isocaloric replacement of SFAs with carbohydrates—the common dietary practice—has little effect95; indeed, replacing SFAs with carbohydrates that have a high GI is linked to higher risk for CHD.98 These findings indicate that increasing PUFA intake (i.e., vegetable oils) in place of either SFAs or carbohydrates is an effective strategy for reducing CHD (see Polyunsaturated Fatty Acids, later). Replacing SFAs with MUFAs has uncertain effects on CVD (see Monounsaturated Fatty Acids, below). The effects of SFAs may also depend on the food source. SFAs from meat sources are associated with higher risk for CVD, whereas SFAs from dairy are associated with lower risk.42 The effects of vegetable sources of SFAs on CVD remain understudied. In addition to other ingredients, different foods contain varying proportions of individual SFAs (e.g., 16:0, 18:0); how these different SFAs influence clinical outcomes remains unclear.93
In sum, the effects of SFAs are heterogeneous and depend on the comparison nutrient, and also perhaps the food source and subtype of SFA—thus highlighting the limitations of SFA content as a major metric for defining healthful foods or diets. Better evidence-based targets for dietary change are the foods themselves (Table 46-1
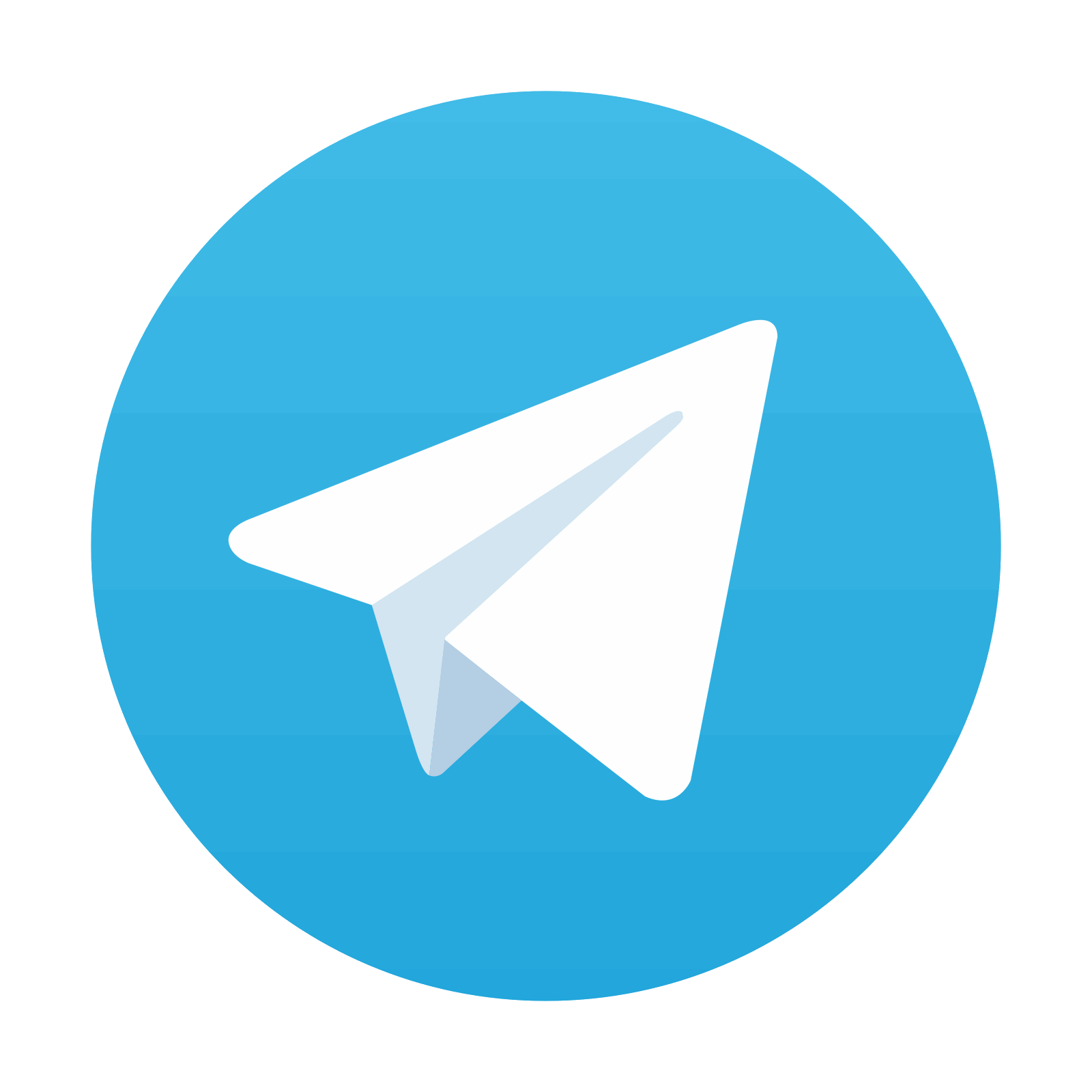
Stay updated, free articles. Join our Telegram channel

Full access? Get Clinical Tree
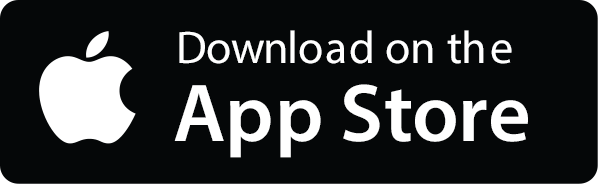
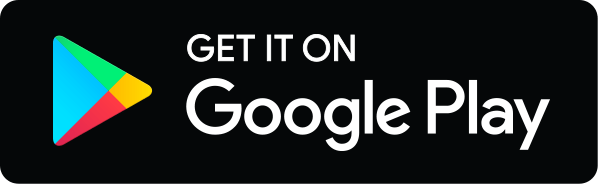