, Jagat Narula2, Yuliya Vengrenyuk3 and Samin Sharma4
(1)
Director, Cardiac Catheterization Laboratory, Director, Structural Heart Intervention Program, Director, Interventional Cardiology Fellowship Program, Zena and Michael A. Wiener Professor of Medicine, Icahn School of Medicine at Mount Sinai, Mount Sinai Hospital, New York, New York, USA
(2)
Director, Intravascular Imaging Core Laboratory, Instructor, Department of Medicine, Icahn School of Medicine at Mount Sinai, Mount Sinai Hospital, New York, New York, USA
(3)
Philip J. and Harriet L. Goodhart Chair in Cardiology, Chief of Cardiology, Mount Sinai St. Luke’s Hospital, Professor of Medicine and Radiology, Associate Dean, Arnhold Institute for Global Health, Icahn School of Medicine at Mount Sinai, Mount Sinai Hospital, New York, New York, USA
(4)
Director, Clinical and Interventional Cardiology, President, Mount Sinai Heart Network, Dean, International Clinical Affiliations, Anandi Lal Sharma Professor of Medicine, Icahn School of Medicine at Mount Sinai, Mount Sinai Hospital, New York, New York, USA
Electronic Supplementary Material
The online version of this chapter (doi:10.1007/978-3-319-62666-6_5) contains supplementary material, which is available to authorized users.
Keywords
Drug-eluting stentsBare metal stentsStent thrombosisBioresorbable vascular scaffoldsPeri-stent contrast stainingIntraluminal filling defectsThrombosisCalcified lesionDissectionIn-stent restenosisLipid-rich plaqueThin fibrous capStent edge dissectionHealed plaque rupture5.1 Introduction
The current generation of drug eluting stents (DESs) has several advantages over the previous generations of DESs and bare metal stents (BMSs) and offers good efficacy and safety. However, the risk of stent thrombosis remains, and the stented vessel remains permanently associated with a rigid metal cage. Bioresorbable vascular scaffolds (BRSs) have recently reenergized the field of coronary intervention; they support transient drug delivery and vessel patency and then gradually tend to complete resorption [1, 2]. Therefore, BRSs might allow restoration of vasomotion and distensibility [3] and may exclude stent thrombosis, “jailing” of the side branches, and struts overhanging over ostial lesions. They may also obviate the inability to surgically graft stented segments [4]. Optical coherence tomography (OCT) has played a major role in the development of BRS technology by providing more precise and detailed morphologic information (compared to IVUS) as a result of its higher resolution [5–8]. While the clinical outcome data from the landmark BRS trials are promising [9–11], the studies had numerous limitations regarding patient and lesion selection. There are a paucity of data on BRS implantation in unselected patient population in “real world” clinical practice, including in-stent restenosis, bifurcation, and severely calcified lesions [12–14]. Similar to metallic stents, suboptimal implantation with incomplete lesion coverage, scaffold underexpansion, and strut malapposition have been the main pathomechanisms for both early and late BRS thrombosis [15]. OCT imaging can help optimize BRS sizing and guide postdilation in order to avoid scaffold underexpansion or fracture.
For metallic stents, OCT has demonstrated exquisite capability to clarify abnormal angiographic findings, including peristrut contrast staining and intraluminal filling defects. Abnormal coronary dilatation at the site of DES implantation demonstrating contrast staining outside the stent struts, peri-stent contrast staining (PSS) , has been shown to be associated with target-lesion revascularization and very late stent thrombosis [16]. OCT can differentiate between two main underlying causes of PSS, incomplete stent apposition and multiple interstrut hollows or cavities between well apposed stent struts [17]. Intraluminal filling defects are occasionally observed on coronary angiography, and thrombosis has often been used as a default diagnosis; however, there are many different causes responsible for the phenomenon, including calcification, dissection, plaque rupture, and artifacts [18]. OCT allows accurate characterization angiographic filling defects and provides important information for treatment optimization.
5.2 Case 1. Preparing a Heavily Calcified Lesion for Accepting a Bioresorbable Vascular Scaffold (Figs. 5.1 and 5.2, Videos 5.1 and 5.2)

Fig. 5.1
A 78-year-old male, an ex-smoker with hyperlipidemia, controlled hypertension, and positive stress cardiovascular magnetic resonance imaging (CMR) presented with stable angina. Coronary angiography showed severely calcified stenoses in the proximal and mid LAD (a1, arrows). To ablate calcified plaque, rotational atherectomy (RA) was performed with a 1.75-mm burr at 160,000 rpm for 88 s resulting in significant lumen gain by angiography (a2). OCT pullback after atherectomy verified effective calcium debulking by visualizing significant modifications of fibrocalcific plaques (b1–b4, asterisks) with minimal lumen area (LA) of 6.0 (b4) and 5.2 mm2 (b1) in the proximal and distal segments respectively

Fig. 5.2
Coronary angiography and OCT imaging after BRS implantation. Two BRS, 3.5/28- and 3.5/18-mm, were deployed in the proximal and mid LAD with a satisfactory angiographic result (a). OCT pullback confirmed good scaffold expansion and apposition (b2, b4, b5) with the minimal scaffold area (SA) of 7.8 and 8.5 mm2 and a short (1.6 mm) scaffold overlap segment (b3, arrow). At the distal edge of the distal BRS, OCT detected a 2.4-mm intima-media dissection (b1, arrows), which was successfully treated with an additional 3.25/12-mm DES. In this case, OCT helped assess the effectiveness of calcium ablation and an immediate post-PCI complication not detected by angiography
5.3 Case 2. Preparing a Totally Occluded Vessel for a Bioresorbable Vascular Scaffold (Figs. 5.3 and 5.4, Videos 5.3 and 5.4)

Fig. 5.3
A 67-year-old male with controlled hyperlipidemia , hypertension , and NIDDM presented with worsening chest pain. Single photon emission computed tomography myocardial perfusion imaging (SPECT-MPI) demonstrated moderate ischemia in the mid LAD area. Coronary angiography showed severely calcified proximal LAD chronic total occlusion (CTO) with collateral flow from the RCA (a1, arrow). CTO was successfully crossed using the antegrade approach with a Fielder wire. Orbital atherectomy was performed in the proximal and mid LAD with 1.25-mm burr at 80,000 rpm for 60 s. OCT pullback performed after lesion predilatation by a noncompliant balloon revealed almost circumferential calcification in the proximal (b4–b7, asterisks) and middle (b1, asterisks) portions of the LAD and visualized significant modifications of fibrocalcific plaques (arrows)

Fig. 5.4
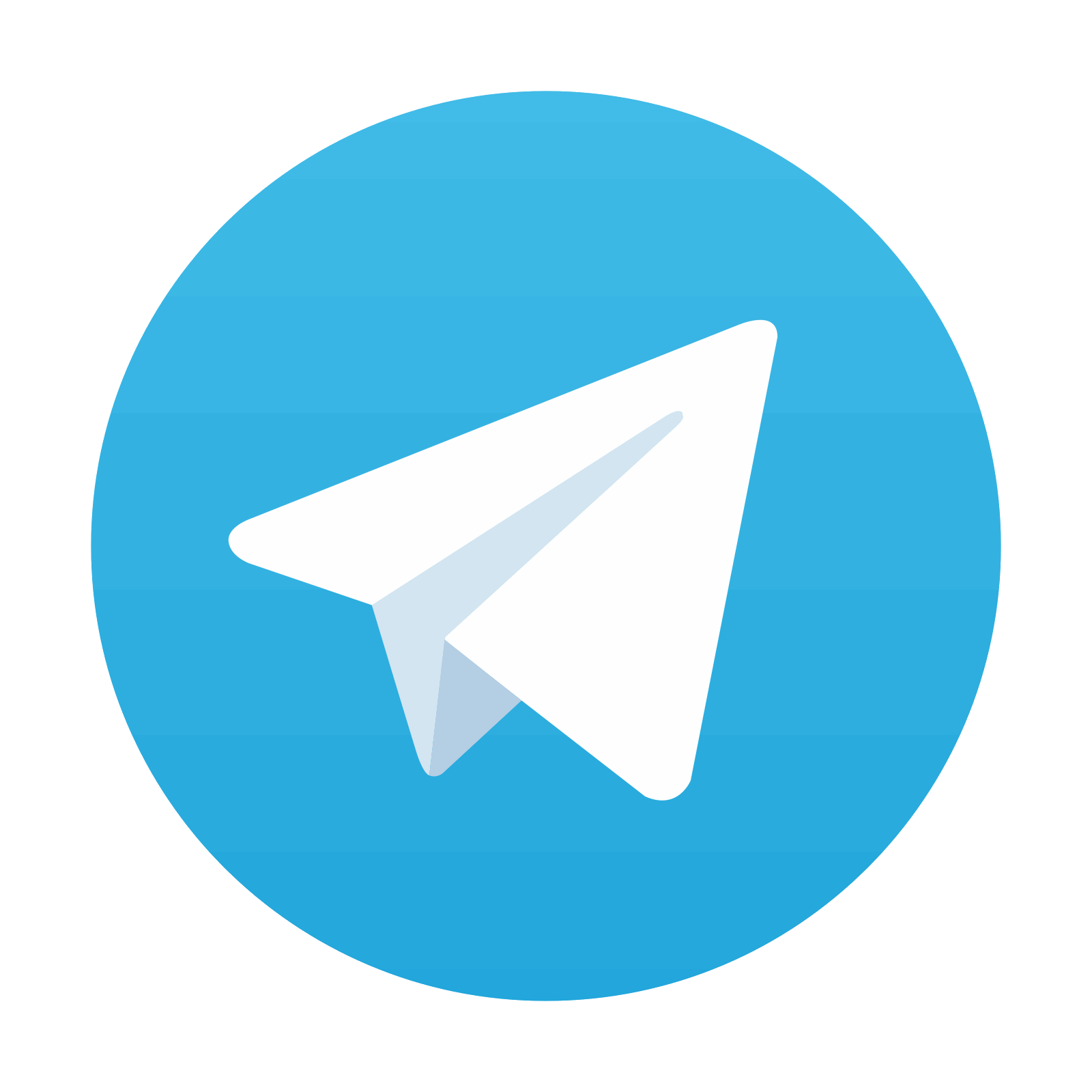
Post-stent imaging . To cover the entire diseased segment, two BRSs were implanted in the proximal (3.5/28 mm) and mid (3.0/28 mm) LAD followed by postdilation with a 3.5-mm noncompliant balloon at 20 atm with satisfactory result by angiography (a). Postprocedural OCT showed good expansion and apposition for both scaffolds without any evidence for dissection or strut malapposition in the whole stented segment with a 1.6-mm long overlap segment (b3). The minimal area of the proximal and distal scaffold was 8.3 and 5.3 mm2 respectively. In the present case, OCT demonstrated BRS implantation in a calcified CTO lesion with good results for scaffold expansion and apposition after adequate lesion preparation and postdilatation
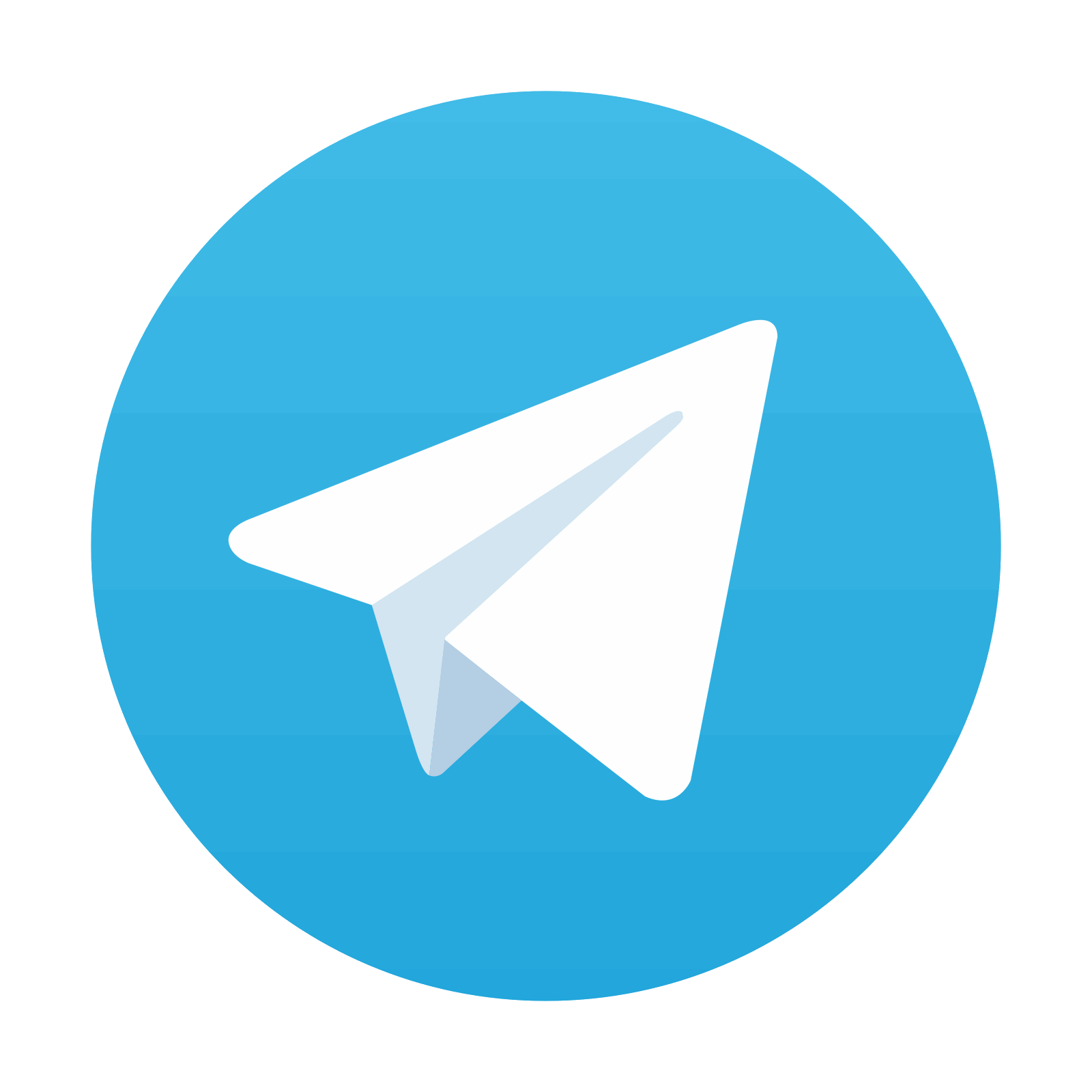
Stay updated, free articles. Join our Telegram channel

Full access? Get Clinical Tree
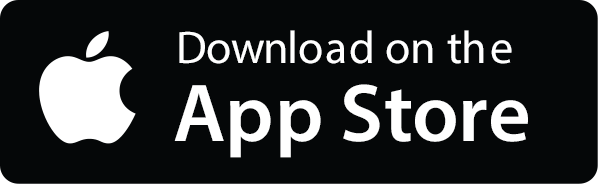
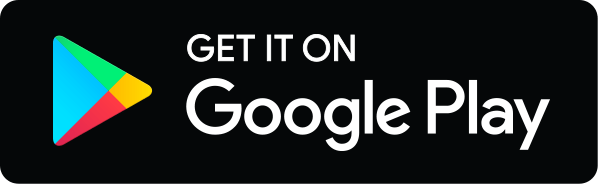
