Background
New echocardiographic parameters (apical rocking [AR], septal flash [SF]) are intended to detect patterns specific to responders to cardiac resynchronization therapy (CRT). The patterns are visually recognized and qualitatively described, requiring experience and training. Speckle-tracking echocardiography can reflect SF and AR by using newly developed, dedicated parameters, such as start systolic index (SSI) and peak longitudinal displacement (PLD). The aim of this study was to investigate whether SSI and PLD can identify potential CRT responders.
Methods
In 125 patients, echocardiograms from before and 9 ± 3 months after CRT were retrospectively analyzed with dedicated EchoPAC prerelease software. From speckle-tracking baseline images, color-coded bull’s-eye displays of SSI and PLD were generated. Cutoff values for both parameters were derived from 25 randomly selected patients and applied to the remaining 100 patients to identify CRT response, defined as a decrease in end-systolic volume of ≥15% during follow-up. The performance of SSI and PLD was compared with the visual assessment of AR and SF by expert and novice readers.
Results
Expert readers detected 77 patients with AR, identifying CRT responders with sensitivity and specificity of 85 ± 2% and 82 ± 2%, respectively. Novice readers reached 74 ± 7% sensitivity and 55 ± 11% specificity, while the sensitivity and specificity of the quantitative analysis were 72 ± 3% and 84 ± 4% for SSI and 80 ± 1% and 75 ± 2% for PLD, respectively.
Conclusions
New speckle-tracking-based quantitative assessment of mechanical dyssynchrony by SSI and PLD performs comparably in identifying CRT responders as visual analysis by expert readers and performs significantly better than novice readers.
Cardiac resynchronization therapy (CRT) with biventricular pacemakers has become a standard therapeutic option in patients with refractory heart failure with reduced left ventricular (LV) ejection fraction and conduction delay. However, about one third of the patients selected by current guideline criteria fail to respond to this expensive and invasive therapy.
Different echocardiographic parameters proposed in the past, using either Tissue Doppler or M-mode imaging, have failed to demonstrate added value in multicenter trials. Myocardial deformation patterns, such as apical rocking (AR) and septal flash (SF), however, are specific for patients likely to respond to CRT and offer added predictive value beyond current guideline criteria. Both AR and SF are surrogate markers of a typical contraction pattern of the left ventricle (most frequent in left bundle branch block [LBBB]) characterized by early septal contraction followed by the late contraction of the posterolateral walls, which is the target of the therapy. Typically, AR and SF are visually assessed and qualitatively described, which requires training and expertise of the investigator, making an objective and quantifiable approach desirable.
Speckle-tracking-based myocardial deformation imaging has emerged as an easy-to-use and validated clinical tool for the quantification of global and regional myocardial function. Image data can be quickly processed and color-coded in a bull’s-eye (BE) display, offering an immediate overview over the regional distribution of a large number of parameters.
In this study, we tested the clinical value of two new speckle-tracking-based parameters that have been developed to detect and analyze myocardial deformation patterns commonly associated with a favorable response to CRT.
Methods
Study Population
Data from 125 patients with heart failure (mean age, 63 ± 10 years; 71% men; 43% with ischemic cardiomyopathy), with good acoustic window, complete LBBB, and in sinus rhythm, who underwent CRT according to current guideline criteria (moderate to severe symptoms, New York Heart Association functional class III or IV on optimized pharmacologic therapy for ≥3 months, QRS duration ≥120 msec, and LV ejection fraction ≤35%) were retrospectively collected from two European centers (University Hospital Gasthuisberg, Leuven, Belgium; and St Vinzenz-Hospital, Cologne, Germany) and analyzed in Leuven, Belgium, and Cluj-Napoca, Romania. Ischemic etiology was documented by coronary angiography or by a history of myocardial infarction. Myocardial scar was assessed using magnetic resonance imaging or scintigraphy.
From our study population, a subgroup of 25 subjects was randomly sampled for deriving cutoff values for the tested parameters (derivation group [DER]). The remaining 100 subjects were used to test the performance of the new methods (test group [TEST]).
The study was approved by the Ethical Committee of the University Hospital Leuven, and the need to obtain written informed consent was waived due to its retrospective nature.
Echocardiography
All subjects underwent a standard echocardiographic examination with a Vivid 7 or Vivid E9 ultrasound system (GE Vingmed Ultrasound, Horten, Norway) immediately before and 6 to 12 months after CRT implantation. All images were acquired in the left lateral decubitus position. Care was taken to obtain high-quality standard apical views with a typical frame rate between 40 and 80 frames/sec. From each view, typically three cardiac cycles were digitally stored for later offline analysis. Spectral Doppler traces of the aortic and mitral valve were stored as reference for cardiac time intervals.
Echocardiographic Data Analysis
All echocardiographic data were analyzed offline using an EchoPAC BT12 SWO (GE Vingmed Ultrasound, Haifa, Israel) in a prerelease version providing the two new analysis options.
LV volumes and ejection fraction were measured in the baseline and follow-up recordings using the modified biplane Simpson method. A decrease in end-systolic volume during follow-up of ≥15% was regarded as response to CRT.
Readers
LV mechanical dyssynchrony was analyzed by two expert readers (R.O.M., I.S.) with 4 and 6 years’ experience in general echocardiography, respectively, and with extensive training in dyssynchrony analysis (>1,000 examinations) as well as speckle-tracking postprocessing.
Data were further analyzed by six novices to dyssynchrony analysis (no previous exposure at all) but 3 to 15 years of experience in general echocardiography (novice readers) (R.B., J.D., C.M., L.M., H.R., A.S.). Novice readers were personally trained by the expert readers in both visual and quantitative analysis using 10 data sets from the DER group.
Visual Dyssynchrony Analysis
Readers were asked to detect SF and AR and to identify CRT responders on the basis of all information they could retrieve from the visual interpretation of the three apical views (including visual assessment of scar extent), but fully blinded to outcome data.
New Quantitative Dyssynchrony Analysis
Standard two-dimensional speckle-tracking analysis was performed on all three apical views of the baseline examinations. For this, end-diastole was manually set as peak R in each image view, while end-systole was defined according to the visible closure of the aortic valve in the apical three-chamber view. Results were displayed in an 18-segment BE. The prerelease software version allowed the generation of two additional parameters for the evaluation of myocardial deformation patterns: start systolic index (SSI) and peak longitudinal displacement (PLD) ( Figure 1 ).

SSI
For SSI, the segmental longitudinal strain during the first half of systole is analyzed. The amplitude of the segmental strain peaks is measured, normalized to the peak global longitudinal strain of the same image view, and multiplied by a factor of 100. Results are displayed as value per segment and as color in a BE format. Negative values are color-coded in orange; positive values are coded in blue. Neutral values are indicated by light green. Whereas the homogeneous contraction of a normal heart will usually result in a completely light green BE, LBBB-like dyssynchrony results in an orange area in the anteroseptal region and blue color in the rest of the BE display. For our analysis, we counted the number of septal and anteroseptal segments with negative values ( Figure 2 ).

PLD
For PLD, the longitudinal displacement during the cardiac cycle is analyzed. The maximum (positive or negative peak) displacement is detected in each segment and displayed numerically and color-coded in a BE format. Any displacement toward the apex, defined as the most distant point from the middle of the connection line between the mitral valve leaflet hinge points, results in a positive value and is coded in green, while displacement away from the apex (negative value) is coded in red. Therefore, normal ventricles show up in a homogeneous green color. A heart with LBBB-like dyssynchrony will typically result in red color in the lateral and posterior walls while the rest of the left ventricle remains green. For our study, we analyzed the lowest displacement value of all segments in the lateral and posterior wall ( Figure 3 ).

Performance of Dyssynchrony Assessment Methods
Using outcome data from the DER group, the associations of both SSI and PLD with response to CRT were explored, and cutoff values for best distinction of responders from nonresponders were defined using receiver operating characteristic analysis ( Figure 4 ).

Subsequently, these cutoff values were applied to data from the TEST group. Sensitivity, specificity, and accuracy for the identification of CRT responders, by analyzing the BE displays of SSI and PLD, were calculated.
The ability of the new quantitative SSI and PLD parameters to identify CRT responders was compared with the visual analysis of dyssynchrony. Furthermore, the dependence of the performance of either approach on the reader’s experience was evaluated by comparing results from expert readers and novice readers. Interobserver agreement between readers was tested for both the quantitative and the visual approach.
Subsequently, a subgroup analysis was performed in patients with QRS width <150 msec.
We further explored to what extent the quantitative parameters SSI and PLD produced similar results to their visual counterparts, SF and AR, by measuring Cohen’s κ.
Statistical Analysis
Continuous data are expressed as mean ± standard deviation. Comparison between groups was done using unpaired t test for continuous variables and the Fisher exact test for categorical variables. Sensitivity, specificity, and accuracy were calculated for the methods using categorical data. For each speckle-tracking-derived parameter, a receiver operating characteristic (ROC) analysis was performed, and the area under the curve (AUC) with the 95% CI was determined. AUCs were used to compare the performance of the visual and the speckle-tracking-based analyses. Interobserver agreement of both quantitative and visual analysis was tested by using Cohen’s κ. Analyses were performed using MedCalc version 14.10.2 (MedCalc Software bvba, Ostend, Belgium). Statistical significance was set at a two-tailed P value of <.05.
Results
Study Population
The characteristics of the study population are summarized in Table 1 . The DER group and TEST group did not differ in any of the parameters. We found no significant difference between responders and nonresponders with regard to age, gender, etiology, presence of a scar in the posterolateral region, QRS width, or baseline LV morphology and function. However, significantly more women responded to CRT (40 vs 8, P < .05).
All patients ( n = 125) | Responders ( n = 77) | Nonresponders ( n = 48) | P | |
---|---|---|---|---|
Age (y) | 63 ± 10 | 64 ± 11 | 62 ± 10 | .49 |
Men/women | 89/36 | 49/28 | 40/8 ∗ | .69 |
QRS width (msec) | 170 ± 26 | 173 ± 21 | 164 ± 32 | .10 |
Ischemic etiology | 54 | 26 | 28 | 1.00 |
Postero-lateral scar | 18 | 7 | 11 | 1.00 |
AR | 77 | 69 | 8 | <.001 |
SF | 83 | 67 | 16 | <.001 |
LVEDV at baseline (mL) | 201 ± 61 | 197 ± 62 | 207 ± 59 | .49 |
LVESV at baseline (mL) | 150 ± 50 | 151 ± 51 | 149 ± 50 | .85 |
LVEF at baseline (%) | 25 ± 7 | 24 ± 6 | 27 ± 7 | .07 |
ΔLVESV (mL) | −27 ± 33 | −44 ± 26 | 6 ± 19 | <.001 |
ΔLVEF (%) | 10 ± 11 | 16 ± 8 | −1 ± 7 | <.001 |
Dyssynchrony Assessment
Visual Dyssynchrony Analysis
Performance of the visual identification of CRT responders on the basis of AR and SF is illustrated in Table 2 . Our results showed that expert readers were more proficient in detecting CRT responders than novice readers ( P < .05).
Sensitivity (%) | Specificity (%) | Accuracy (%) | |
---|---|---|---|
Visual analysis | |||
Expert readers | 85 ± 2 | 82 ± 2 | 84 ± 1 |
Novice readers | 74 ± 7 ∗ | 55 ± 11 ∗ | 68 ± 1 ∗ |
SSI | |||
Expert readers | 73 ± 1 | 85 ± 2 | 77 ± 1 |
Novice readers | 72 ± 3 | 84 ± 4 | 75 ± 2 |
PLD | |||
Expert readers | 80 ± 1 | 78 ± 1 | 80 ± 1 |
Novice readers | 80 ± 1 | 75 ± 2 | 79 ± 2 |
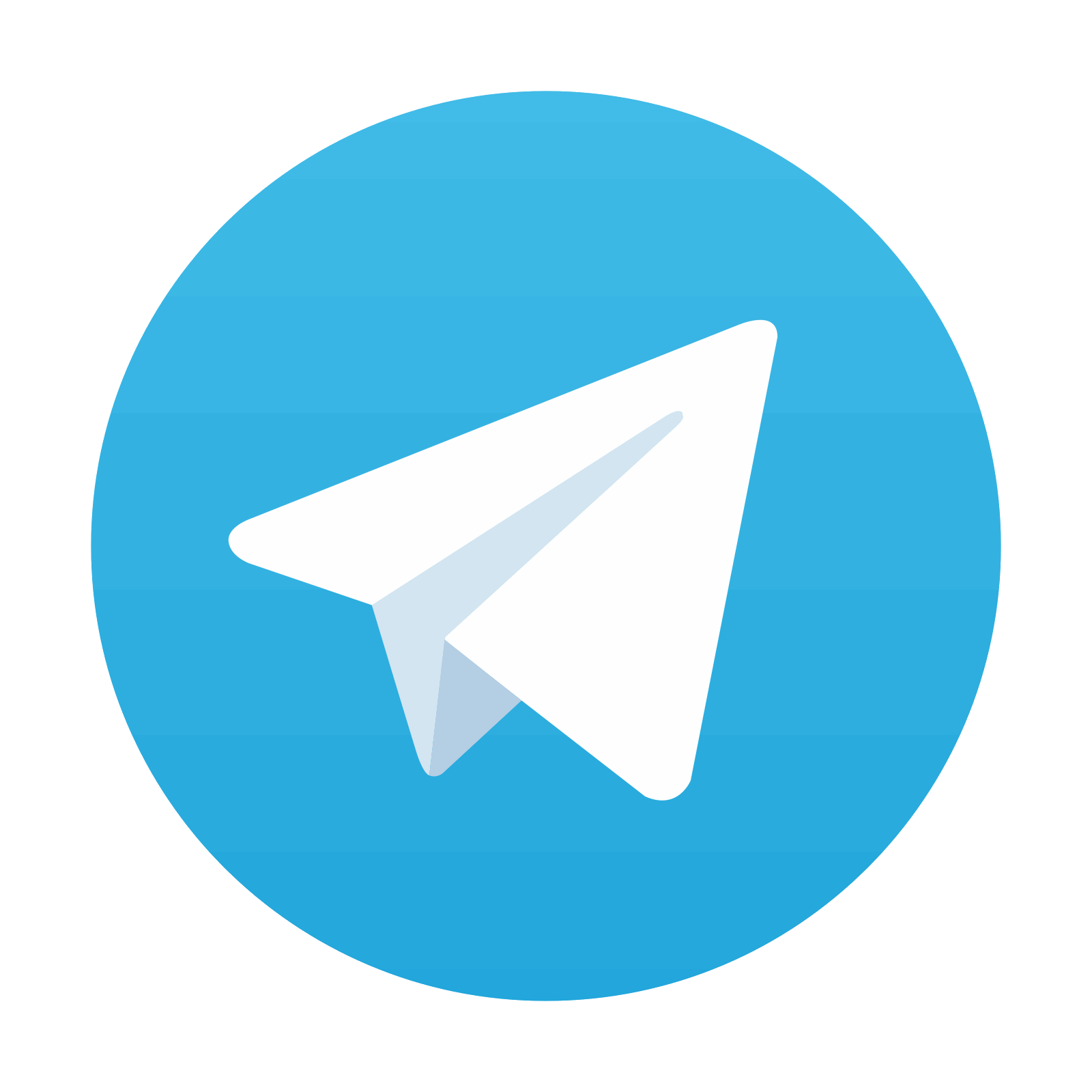
Stay updated, free articles. Join our Telegram channel

Full access? Get Clinical Tree
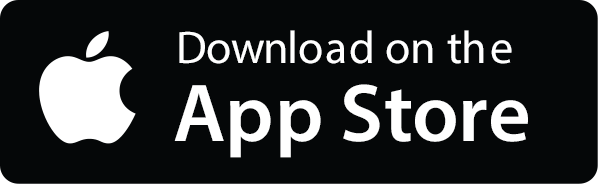
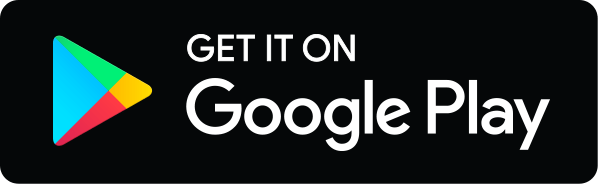
