Chapter 68 Neurosurgery
Intracranial Dynamics
The first principle is obvious. The cranial cavity has a fixed volume comprised of (1) brain tissue (parenchyma), (2) CSF, and (3) blood vessels and intravascular blood. According to the Monro-Kellie doctrine, the sum of these components within the fixed volume of the cranial cavity implies that an increase in one component must be accompanied by an equal and opposite decrease in one or both of the remaining components.1 If this does not occur, the ICP will rise to levels close to the systemic blood pressure, producing a reverberating blood flow pattern with no net flow. The clinical implications are also straightforward. For each intracranial component, there is a family of pathologic conditions of excess volume and a means to improve that excess (Table 68-1). A consequence of this principle is that if there is an elevation in the volume of any one compartment, there is a stage of compensation in which the volume of one or more other compartments can be reduced to avoid elevations in the ICP.
Table 68-1 Intracranial Excess Volume Syndromes and Therapy
COMPONENT | EXCESS VOLUME SYNDROME | SPECIFIC TREATMENT |
---|---|---|
Brain tissue | Edema: Cytotoxic, vasogenic, perineoplastic, inflammatory | Diuretics: Mannitol, furosemide, hypertonic saline; steroids for perineoplastic and inflammatory vasogenic edema |
Vascular | Elevated PCO2: Hyperperfusion state with loss of autoregulation as in severe hypertension, after trauma or AVM removal; relative venous obstruction | Increased ventilation; diuretics (in hyperperfusion state, avoid mannitol), barbiturates; clear venous obstruction; elevate head of bed (to reduce venous volume) |
Cerebrospinal fluid | Impaired absorption with congenital, posthemorrhagic, or postinfectious hydrocephalus, communicating or obstructive; loculations; arachnoid or periventricular cysts; rare increased production of CSF with choroid plexus papilloma | Ventricular external drainage (or lumbar drainage only if no threat of herniation) or shunt; with flocculation, or with some types of obstructive hydrocephalus, endoscopic fenestration or third ventriculostomy may be possible; acetazolamide and steroids may temporarily decrease CSF production |
Mass lesion | Tumor, cyst, abscess, hematoma, radiation necrosis, or cerebral infarction necrosis | Remove, fenestrate, aspirate lesion (often with stereotactic guidance); less commonly, might be useful to enlarge intracranial volume by decompression |
The blood flow to any brain area is generally abundant, exceeding demand by a wide margin, so that O2 extraction ratios are often low. The brain vasculature matches the blood flow to tissue metabolic demand and the CBF generally maintains what is needed, despite wide variations in systemic blood pressure, by a phenomenon known as autoregulation. Factors such as an elevated or decreased arterial PCO2 shift the curve as indicated. In the setting of traumatic brain injury, the curve becomes more pronounced (i.e., smaller changes in blood pressure or PCO2) and affects the CBF dramatically (Fig. 68-1). If tissue demand exceeds autoregulation, or if CBF declines for pathologic reasons, the first defense is that the O2 extraction will increase (i.e., arteriovenous O2 difference, AVDO2). The tissue begins to dysfunction at levels below 0.25 mL per g of brain tissue per minute. With levels between 0.15 and 0.20 we may encounter reversible ischemia; however, infarction will occur when levels range between 0.10 and 0.15 (Fig. 68-2). The metabolic consumption of oxygen in the brain (CMRO2) is decreased after traumatic brain injury to levels between 0.6 and 1.2 µmol/mg/min. Complete loss of blood flow to any brain area results in infarction (irreversible damage) within a few minutes. Swelling of the infarcted tissue takes days to peak and weeks to resolve.2
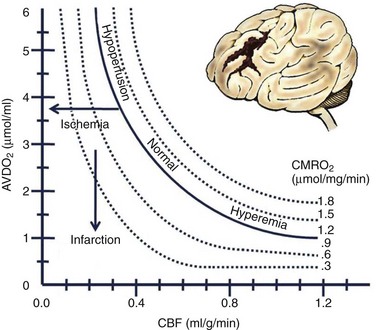
FIGURE 68-2 Relationships among cerebral flow, metabolism, and oxygen extraction in normal and pathologic circumstances.
(From Rangel-Castilla L, Gasco J, Nauta HJ, et al: Cerebral pressure autoregulation in traumatic brain injury. Neurosurg Focus 25:E7, 2008.)
A fourth principle derives from the other three and the fact that injured tissue swells, making obvious the potential for a cascading injury by a vicious cycle mechanism (Fig. 68-3). If the stage of compensation (see earlier), even with therapy, is exceeded, and ICP is elevated high enough by some mechanism so that cerebral perfusion pressure (CPP) declines, CBF can decline to levels at which tissue injury occurs.
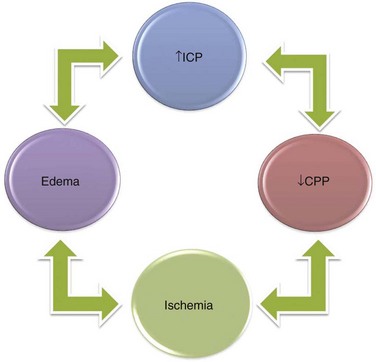
FIGURE 68-3 Relationship among increased ICP, reduced CPP, development of ischemia and infarction, and cerebral edema.
In the management of intracranial pathology, ICP and CPP are easy to measure continuously and thus serve as highly practical surrogates for the more fundamental, but much more difficult to measure, CBF. However, these are not equivalent, and the limitations of these parameters for guiding therapy need to be remembered. Regardless of causation, when concern arises about the possibility of cascading injury, every effort is made to keep the CPP in the realm of 60 mm Hg (range, 50 to 70 mm Hg) and ICP below 20 mm, Hg if possible. Routinely using pressors and volume expansion to maintain CPP higher than 70 mm Hg is not supported based on systemic complications.3
The Glasgow Coma Score (GCS; see later, “Traumatic Brain Injury”) provides a clinical functional measure of the degree of mass effect and advanced raised ICP. Although it is a useful standardized functional measure of the more advanced stages of mass effect or raised ICP, it was never really intended to focus on more subtle functional changes. A recently introduced coma scale by Widjicks and colleagues4 has delineated the FOUR score (full outline of unresponsiveness) consisting of four components (eye, motor, brainstem, respiration). Each has a maximal score of 4 and takes into account the subtleties related to brainstem activity and breathing patterns occurring during the rostrocaudal decay described.
Normal ICP varies over a wide range, with generally accepted values between 0 and 20 mm Hg. Diffuse raised ICP, in the fully evolved pure form, results in a clinical picture that may include symptoms of headache, nausea and vomiting, double vision, and obscuration of vision. The accompanying clinical signs may include papilledema and sixth cranial nerve palsy with lateral rectus weakness and side by side diplopia, initially worse on far vision or gaze directed toward the side of the palsy. The papilledema is a mostly chronic phenomenon and is not seen acutely. The sixth nerve palsy of raised ICP can occur regardless of its cause and does not imply direct involvement by a mass lesion, large or small, on the sixth nerve. In this situation, the sixth nerve palsy is a false localizing sign. With raised ICP, there may also be obscurations of vision, in which patients report that their vision temporarily fades or becomes gray, in combination with headache. Again, these obscurations are caused by the effect of diffusely increased ICP on the sensitive optic nerves. They do not imply the presence of a focal mass lesion directly affecting the optic nerves or pathways. Intuitively, it seems that if there is a slow increase in a process raising ICP, the pressure would also rise slowly and evenly, in pace with the evolving process. However, as first shown by Lundberg in 1960,5 the intermediate stages of decompensation are characterized by transient pronounced elevations in ICP (to 60 mm Hg) that characteristically plateau for up to 45 minutes and then transiently cycle down again to a more normal range.
Table 68-2 summarizes the relationships among ICP, mass lesions, and ventriculomegaly.
Cerebrovascular Disorders
Cerebrovascular disorders encompass a host of disorders, congenital and acquired (Box 68-1).
Arteriovenous Malformation and Fistula
Clinical presentation is usually that of a hemorrhagic stroke picture, typically an intracerebral or subarachnoid hemorrhage, or some combination. The patient complains of sudden-onset severe headache with or without focal neurologic deficit and meningismus. These symptoms can occur in all degrees of severity, but are less commonly fatal than after aneurysmal subarachnoid hemorrhage into the basal cisterns. Investigation usually begins in the emergency department with a computed tomography (CT) scan showing the hemorrhage. MRI or magnetic resonance angiography (MRA) studies typically follow, often showing enlarged afferent and efferent vessels. The role of CT angiography is evolving. Regardless of the mode of presentation, diagnosis is ultimately made by conventional catheter cerebral angiography (Fig. 68-4). It is based on demonstration of arteries and veins on the same conventional angiographic image, proving the high-flow shunting of blood through the nidus network or fistulous vessels. In an arteriovenous fistula, the shunting occurs through short, sparse, larger-diameter channels so that a cloudlike nidus of smaller vessels is not evident. Instead, the enlarged afferent arteries appear to connect directly with the enlarged efferent veins. In the typical AVM, there is a cloudlike nidus, or network of smaller vessels, seen well on angiography but not necessarily fully appreciated on MRI or MRA. The AVM can occur in all locations and with varying degrees of size, complexity, and compactness.
Therapy can include surgical excision, endovascular embolization of the nidus, and/or SRS. The decision to embark on any treatment depends on the assessment of the patient’s treatment risk in comparison with the natural risk. The studies of Ondra and associates6 have fairly well characterized the natural risk. Hemorrhage occurs with a frequency of approximately 4%/year, and because only approximately 25% of these are severe, with permanently severe disability or death, the catastrophe risk is only approximately1%/year. The treatment risk, therefore, must be favorably low to justify action in most situations, and delays in treatment to allow for patient acceptance and optimize the circumstances for therapy are understandable and reasonable. The main reason to recommend treatment is that the treatment may offer a lower risk over the long term. Younger patients have the most to gain by such an assessment. It may be possible to define features of individual AVMs that adjust the natural risk upward or downward, but so far these have been difficult to prove. For the purposes of clinical decision making, the hemorrhage risk is generally taken across the board for all AVMs as a starting point. An across-the-board assessment of treatment risk, however, is clearly not warranted because each AVM. Size, location relative to access by the treatment method considered, and location relative to proximity to deficit-prone brain structures are all important variables to consider. Another feature is the compactness of the AVM, with some forming a tightly clustered nidus, with little brain tissue in between, and others being diffuse and rambling, with scattered small clusters of nidus vessels encompassing large intervening areas of functional brain.
Obviously, the smaller, more compact AVMs located superficially in areas of silent brain function are the most attractive for open surgical resection. Diffuse large AVMs encompassing deficit-prone areas of brain are least attractive to open surgery and other methods. Spetzler and Martin7 have devised a grading system for assessing risk with open operation. However, any decision is ultimately based on how an individual neurosurgeon assesses the risk for that AVM in a patient, with his or her limits of risk tolerance.
Cavernous Angioma: Cavernous Malformation
The cavernous angioma is a highly characteristic, usually almost spherical, discrete lesion, composed of a cluster of vascular sinusoids fed by small vessels in the arteriolar size range or smaller. Unlike the AVM, large feeding arteries and draining veins are not seen, and diagnosis by catheter angiography is not possible. The sinusoids are tightly compacted and there is no intervening brain tissue, a diagnostic feature. At surgery, they often appear as a discrete, mulberry-like collection of thin-walled vascular sinusoids with a greenish hemosiderin rim in the surrounding brain edge. When incised, bleeding from the lesion is minimal and easy to control, unlike the AVM, which bleeds vigorously if entered. The sinusoids often seem to be admixed with small chronic hematomas containing blood in various stages of decomposition. Many are older and contain birefringent yellow cholesterol crystals. Early autopsy studies8 noted out that they should be more common than recognized clinically. However, the lesions were not usually evident on CT or conventional catheter angiography available at that time. The clinical entity was not fully appreciated until the more widespread availability of MRI in the 1980s. The lesions are easily seen on MRI, where their appearance is diagnostic. They show a center containing some small, high-intensity signal foci surrounded by a null signal corona, dark on T1- and T2-weighted images, that corresponds to hemosiderin deposition in the adjacent brain.
Traumatic Arteriovenous Fistula
Both the internal carotid artery (ICA) and vertebral artery enter the cranial cavity immediately after passing through a venous network. The ICA passes through the cavernous sinus, which communicates with the superior ophthalmic vein, petrosal sinus, and sphenoparietal sinus. The vertebral artery passes through a venous plexus at the occipital-C1 epidural space, which communicates with the jugular vein, epidural venous plexus, and paraspinal venous plexus. Trauma leading to a tear in the carotid or vertebral artery at its tether point passing through the skull base can lead to fistula with the surrounding venous plexus. The consequences may vary in severity and suddenness but typically include periorbital swelling, with proptosis and scleral edema in the case of the carotid-cavernous fistula (CCF) and prominent pulsatile bruit in the case of the vertebral-jugular fistula. Intraocular pressure measurement by tonometry can guide the urgency in treating CCF. Radiologically, dilation of the superior ophthalmic vein is characteristic (Fig. 68-5). These lesions are usually treated by endovascular techniques. A catheter is advanced through the tear in the artery into the venous side of the fistula. The high-flow and large fistulous channel facilitate this process. Embolic material, a coil, or a detachable balloon is then used to occlude the venous side of the fistula. When conventional transvenous routes fail, a direct approach via transorbital puncture may be required to provide endovascular therapy.9
Cerebral Saccular (Berry) Aneurysms
Distal embolization of clot material is a rare occurrence. Calcification can occur in the wall in advanced cases and the adjacent brain can become gliotic from chronic pressure, making seizures also a possible presentation. An enlarging aneurysm can also compress an adjacent cranial nerve; the optic and third nerves are usually affected by this mechanism. Generally, however, it is much more common for an aneurysm to present with subarachnoid hemorrhage. The proliferative ability of the connective tissue in the dome of the forming aneurysm can be exceeded by the stretching force, leading to rupture. The onset is unpredictable and appears to occur at a surprisingly low rate. Incidentally discovered unruptured aneurysms bleed at a rate depending on size. Those smaller than 1 cm bleed at a rate of 0.05% to 0.5%/year, whereas those larger than 1 cm bleed at a rate of 1% to 2%/year. Treatment recommendations for unruptured aneurysms smaller than 5 mm in diameter vary widely based on patient preference, aneurysm accessibility to treatment, and surgeon’s assessment of risk. Once hemorrhage from an aneurysm occurs, the situation changes dramatically. Bleeding of highly oxygenated arterial blood occurs suddenly into the surrounding CSF-containing subarachnoid space, which initially offers little backpressure. Aneurysmal subarachnoid hemorrhage can occur in all gradations of severity. In most patients, accumulated blood in the basal spinal fluid cisterns leads to a coagulum that spontaneously stops the bleeding. In 10% to 15% of patients, the bleeding is so severe at the outset that death occurs before they even reach the hospital. Approximately 40% die following the initial hemorrhage but at a later stage. Rebleeding occurs with a peak incidence in the first 24 hours after the initial event. If the aneurysm is left unsecured, the rebleed rate is 20% in the first 2 weeks, 50% in the first 6 months, and thereafter 3% to 4%/year.10 Rebleeding is the principal cause of death, usually by raised ICP.
Treatment of the vasospasm includes elevating the blood pressure, blood volume, and cardiac output in an attempt to bring more blood flow past the narrowing in the vessels (Box 68-2). The calcium channel blocker nimodipine appears to reduce the incidence of delayed ischemic neurologic deficits and prevents vasospasm, probably through opening collaterals to the ischemic brain. Its direct effect on the vasospasm is still in question. Phase IIA trials with clazosentan, an endothelin A antagonist, have been promising in reducing the frequency and severity of cerebral vasospasm.11 Attenuation of vasospasm by statins or magnesium sulfate is controversial according to recent literature.12,13
Investigation of patients with a suspected aneurysmal subarachnoid hemorrhage begins with an assessment of the history. Because the hemorrhage occurs into the subarachnoid space and not brain tissue, there is usually no focal neurologic deficit. Symptoms of sudden-onset headache with meningismus are classic and reflect a sudden rise in ICP and irritation of the basal meninges by the blood. In severe cases, the patient may be comatose or uncooperative. A report of a strokelike picture of sudden onset of neurologic signs and symptoms should prompt CT scanning, which reveals blood filling the subarachnoid cisterns (Fig. 68-6A). Because the hemorrhage can occur in all gradations of severity, the difficulty comes in recognizing the patient with the small sentinel hemorrhage who arrives in good condition with only the complaint of an alarmingly severe sudden onset of headache. Headache from increased ICP may be only transient until compensatory mechanisms occur. Residual headache and neck stiffness from meningismus, although usually present, may not be impressive.
If the CT scan or lumbar puncture is positive for subarachnoid blood, the next step is usually conventional catheter cerebral angiography (see Fig. 68-6B). CT angiography with bolus IV contrast has become increasingly convenient and accurate; thus, it is being used more frequently. Newer scanners are capable of large numbers of simultaneous slices so that three-dimensional reconstruction with good registration and surprising detail is possible (see Fig. 68-6C). With selections in density windowing, the relation of the aneurysm to any blood clot can also be visualized.
Treatment of a cerebral aneurysm can be performed in a variety of ways:
In terms of the aneurysm configuration, a narrow neck is a favorable attribute for treatment by open surgical clipping or endovascular embolization methods. Wide-necked or sessile aneurysms with a high neck-to-fundus ratio make it difficult to contain coils in the fundus without protrusion into the parent artery. A stent placed in the parent artery may address this problem, but the technology is evolving and stenting is currently practical only in more proximal locations. Generally, wide-necked aneurysms gravitate toward open surgical treatment, although stent-assisted coiling can be also used in some cases. Particularly interesting is the recently introduced concept of functional reconstruction with the use of flow diverters. These stents induce reverse remodeling and delayed disappearance of the aneurysm via thrombosis. They are especially useful for very small, giant, wide-necked, or otherwise difficult to treat aneurysms and can be used with intramural coil placement.14,15
In situations in which the aneurysm location or configuration presents a clear advantage of one method over the other, decision making is fairly easy, even at centers at which all current methods are available. Problems in decision making involve aneurysms that are favorably situated and configured so that they would be attractive to treat by open surgical clipping or endovascular coil occlusion. In the past, open surgical clipping was considered first,and the patient was referred for endovascular coil occlusion only if there were reasons to avoid open surgery. However, since the European prospective randomized trial of surgery versus open clipping, this paradigm is now open to question; it is becoming more common to consider endovascular treatment before open surgical clipping in aneurysms treatable by either method.16
Spontaneous Intracerebral Hemorrhage
Spontaneous intracerebral hemorrhages into the brain parenchyma are common, accounting for approximately 10% of all strokes. They generally occur in older patients, usually because of degenerative changes in the cerebral vessels that are often associated with chronic hypertension (Box 68-3). In younger patients, they are more likely related to drug abuse or vascular malformation. They can occur anywhere in the cerebral circulation or brainstem but are classically described in association with small degenerative aneurysms (microaneurysms; also known as Charcot-Bouchard aneurysms) at the junctions of the perforating vessels and larger vessels at the skull base. They are typically on the MCA junctions with the small perforating lenticulostriate vessels, leading to hemorrhage into the putamen. The clinical presentation is with a stroke pattern of sudden-onset neurologic signs and symptoms that depend on the area of brain affected. Symptoms are more likely to include headache than ischemic stroke. The diagnosis is with CT, usually done in an emergency department setting. The size and location of the acute hematoma are well visualized with CT, as well as any associated brain shift or hydrocephalus (Figs. 68-9A and 68-10). In older patients with a known history of hypertension and classic CT appearance of a hematoma in the putamen, thalamus, cerebellum, or pons, further diagnostic studies are generally not indicated. Rehemorrhage is unlikely in that setting. However, further investigation might be warranted with an atypical hematoma location or appearance, especially if there is any component of subarachnoid blood. Also, investigation is usually recommended for younger patients without known hypertension and those with a potential underlying cause for hemorrhage (e.g., history of neoplasm, blood dyscrasias, bacterial endocarditis).
The Surgical Trial in Intracerebral Hemorrhage has noted a lack of clinical outcome difference when comparing early surgery with conservative management.17 If indicated, surgical evacuation is usually done by craniotomy over the most accessible part of the hematoma (see Fig. 68-9B). Intraoperative ultrasound is often helpful in finding hematomas that do not quite come to the cortical surface and in monitoring the progress of the evacuation. The goal of surgery is decompression more than complete removal, but is generally done as far as safely practical. The wall of the hematoma cavity is inspected for any underlying cause and a biopsy is taken, if indicated. Putamen hemorrhage can sometimes be evacuated with minimal surgical damage to the overlying brain by a trans-sylvian fissure–transinsular approach. Stereotactic aspiration and methods with fibrinolytic agents are being developed and may be a consideration for patients with hematomas in deep locations that are otherwise difficult to access.
A special situation to consider is the patient with cerebellar hemorrhage (see Fig. 68-10). Surgery is offered more readily in these cases because the danger of sudden deterioration from brainstem compression is more of a concern, and because even extensive damage to the cerebellum itself is generally survivable, with good functional outcome. Patients with fourth ventricular obstruction and hydrocephalus from cerebellar hemorrhage can sometimes be treated with ventricular drainage alone but are usually offered surgical evacuation of the hematoma by suboccipital craniotomy because of the risk for brainstem compression.
Central Nervous System Tumors
Intracranial Tumors
In general, the incidence of primary brain tumors is higher in whites than in blacks, and mortality is higher in males than in females. According to the Central Brain Tumor Registry of the United States (CBTUS), the overall incidence of primary brain tumors was 14.8/100,000 person-years between 1998 and 2002 (CBTUS statistical report, 2005-2006). On the other hand, secondary tumors outnumber primary brain tumors by 10 : 1 and occur in 20% to 40% of cancer patients.18 Because no national cancer registry documents brain metastases, the exact incidence is unknown, but it has been estimated that 98,000 to 170,000 new cases are diagnosed in the United States each year.19
Imaging Studies
The initial workup generally involves a relatively inexpensive diagnostic tool, a CT scan of the brain. CT provides a rapid means of evaluating changes in brain density such as calcifications, hyperacute hemorrhages (<24 hours old), and skull lesions. MRI of the brain, however, is the gold standard modality for diagnosis, presurgical planning, and post-therapeutic monitoring of brain tumors. Gadolinium contrast enhancement with MRI is more sensitive in demonstrating defects in the blood-brain barrier and localizing small metastases (up to 5 mm). It can be used in patients allergic to iodine and those with renal failure. Advances in MRI techniques have evolved from strictly morphology-based imaging to a modality that encompasses function, physiology, and anatomy. Diffusion-weighted imaging can help distinguish between gliomas and abscesses, and perfusion-weighted imaging can predict response to radiotherapy in low-grade gliomas. Functional MRI can be used when planning surgery for tumors in eloquent areas of the brain to enable radical resection with less morbidity. Diffusion tensor imaging can demonstrate the effect of a tumor on white matter tracts. MRA is used more routinely as a noninvasive modality to evaluate the vascularity of a tumor or anatomic relationship of a tumor to normal cerebral vasculature.20
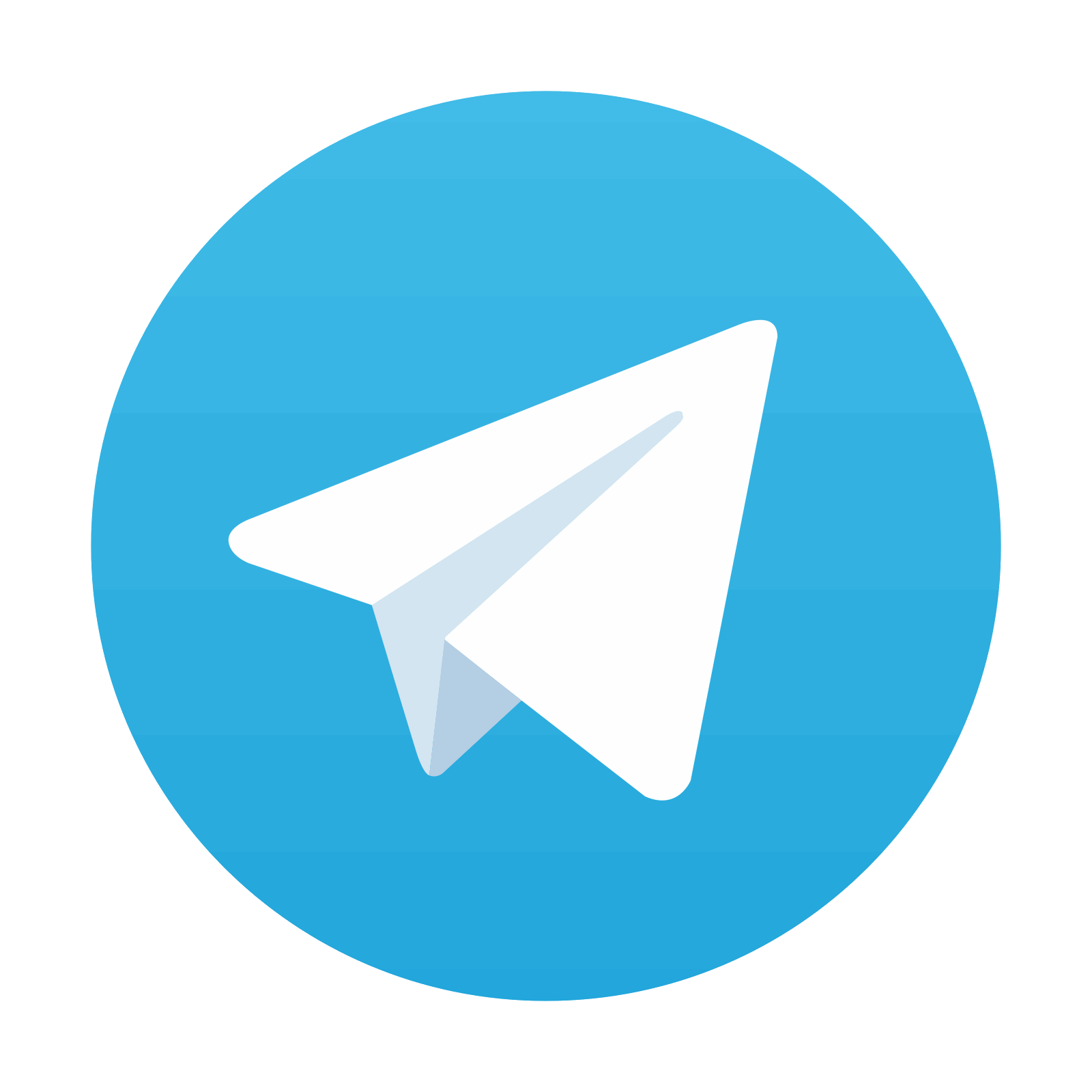
Stay updated, free articles. Join our Telegram channel

Full access? Get Clinical Tree
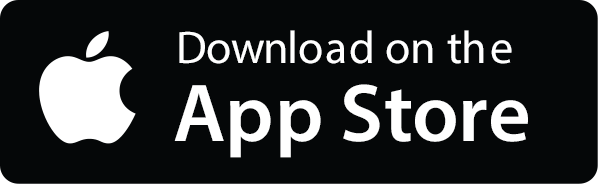
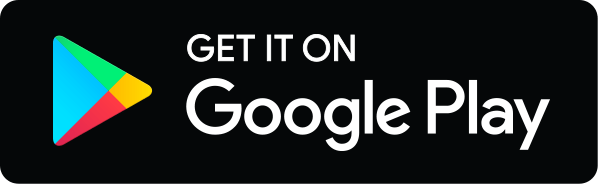