Neurodevelopmental Outcomes after Heart Surgery in Children
Caren S. Goldberg
Jane W. Newburger
The survival of children with congenital heart disease has improved dramatically over the past four decades. As a result, the number of adults with congenital heart disease is now believed to exceed the number of children (1,2,3,4). As mortality has declined, neurologic and developmental morbidities in survivors have come increasingly into focus. Poor school performance and the resultant need for educational support across the developmental span from kindergarten through 12th grade may have considerable personal and societal costs. Furthermore, the increasing number of adults with congenital heart disease has highlighted the consequences of neurodevelopmental impairments for employability and mental health (5). Neurodevelopmental disabilities can derive from innate or genetic factors, from aberrant fetal circulation, from the physiology and sequelae of congenital heart disease itself (e.g., chronic severe cyanosis, failure to thrive, cardiac arrests secondary to arrhythmia), or from the procedures, such as cardiac catheterization or cardiac surgery, used to treat congenital heart disease. Particularly in congenital heart disease, it can be difficult to separate developmental outcomes for a particular diagnosis and its genetic underpinnings from consequences of surgical and transcatheter procedures used in its management. It is likely that central nervous system effects of congenital heart disease are cumulative and affected by the complex interaction of genetic, preoperative, intraoperative, and postoperative factors (6,7). In this chapter, we review variables that contribute to neurodevelopmental outcomes in children after heart surgery and summarize findings related to long-term neurodevelopmental outcomes for more common, complex congenital heart malformations.
Genetic Abnormalities
Genetic abnormalities may cause both congenital heart defects and abnormalities of central nervous system structure and function. Examples include chromosomal disorders (e.g., trisomy 13, 18, or 21), microdeletions (e.g., velocardiofacial syndrome secondary to 22q11 microdeletion), or mutations (e.g., Williams syndrome, Alagille syndrome, or CHARGE syndrome). The genetic causes of other syndromes with multiple congenital anomalies, such as VATER syndrome, are unknown. Children with genetic syndromes have much worse neurodevelopmental outcome than those without recognizable syndromes (8,9). Furthermore, it is suspected that genetic factors may independently underlie delayed development, either through a primary effect on the central nervous system or by affecting host susceptibility and resiliency, even in some patients without a recognizable constellation of congenital abnormalities.
Specific types of congenital heart defects may be associated with different chromosomal abnormalities with varying molecular pathways that impact central nervous system structure and function. For example, tetralogy of Fallot (TOF) can be associated with mutation or deletion of several different genes (NKX2.5, JAG1, TBX5, TBX1, and FOXC2) and with several clinical syndromes, such as velocardiofacial syndrome or trisomy 21 (10,11). Some genes associated with congenital heart disease (e.g., TBX5, NKX2.5, and JAG1) have been found in the brain as well as the heart (12). JAG1, mutated or deleted in Alagille syndrome, encodes a ligand for the notch intercellular signaling pathway of tremendous importance in brain development (13,14,15,16), and approximately one in five patients with Alagille syndrome have profound developmental disabilities (17). In TOF patients without other features of Alagille syndrome, polymorphisms in JAG1 may be associated with both cardiac and neurocognitive phenotypes.
Mutations causing congenital heart defects may be associated with specific neurodevelopmental profiles. For example, although the clinical phenotype associated with 22q11 microdeletion is variable (18), a reasonably consistent neurodevelopmental profile has emerged. Intelligence quotient (IQ) can range from that associated with profound mental retardation (19) to average (20,21,22,23,24), with the prevalence of learning disabilities approaching 100% (21). In adults with velocardiofacial syndrome, specific deficits have been reported in visual–spatial ability, problem solving and planning (executive functions), abstract social thinking, and attentiveness (23,25,26). Studies in children report that verbal IQ tends to exceed performance IQ, with velocardiofacial syndrome patients displaying relative strengths in rote verbal knowledge and memory and relative weaknesses in visual–spatial and perceptual skills and mathematical abilities (27,28). Pathogenic copy number variants (regions of DNA gains or loss) have been associated with worse neurodevelopmental outcomes in children with single ventricle (29).
Finally, some genetic mutations that cause structural heart defects are associated with psychiatric illness, including, the 22q11 microdeletion (19,23,28,29,30,31,32). In adults with 22q11 microdeletion, the prevalence of late-onset psychosis, most commonly schizophrenia and schizo-affective disorders, is 10% to 20% (21,33,34). With advances in research on genetic causes of congenital heart disease, it is likely that an increasing number of genetic and epigenetic abnormalities will be linked to neurologic and developmental outcomes in congenital heart disease patients.
Brain Anatomy, Pathology, and Neuroimaging
Cerebral dysgenesis has been reported in autopsy series to occur in 10% to 29% of children with congenital heart disease and may include such features as microdysgenesis, incomplete operculization, microcephaly, and agenesis of the corpus callosum (7,35,36,37,38). The incidence varies by lesion and is particularly high in hypoplastic left heart syndrome (HLHS) (35,36,37,39). The cause of cerebral dysgenesis may be related to genetic factors or to abnormalities of fetal cerebrovascular hemodynamics caused by the particular congenital heart defect. For example, fetuses with HLHS, whose cerebral perfusion is supplied retrogradely through the ductus arteriosus, as well as those with hypoplastic right heart syndrome and other congenital defects, have been shown to have cerebrovascular resistances that are lower than normal (40,41). Interestingly, Williams et al. found
that among children with single ventricle malformations, having a lower cerebrovascular resistance was associated with better scores on developmental assessments (42).
that among children with single ventricle malformations, having a lower cerebrovascular resistance was associated with better scores on developmental assessments (42).
Fetuses with congenital heart disease and with low cerebral-to-placental resistance ratios (<1) have smaller head circumferences than normal (41). Multiple studies have demonstrated an association of smaller brains and congenital heart disease (41,43,44,45). Limperopoulos et al. (44) performed brain magnetic resonance imaging (MRI) on third-trimester fetuses with a variety of congenital cardiac malformations and found that compared to fetuses with normal cardiac anatomy, with adjustment for gestational age and weight, those with cardiac malformations had smaller brain volumes and impairment of neuroaxonal development and metabolism. In addition, work by Schaer et al. (45) supports the interaction between the hemodynamic consequences of congenital heart disease and its genetic background on neurologic development. These investigators performed MRI on 53 patients with 22q11.2 deletion and found that compared to healthy controls, cerebral volume was 6.9% less; yet in those patients with 22q11.2 deletion and associated congenital heart disease that required cardiac surgery, cerebral volume was reduced by 16.9% (45).
In addition to cerebral dysgenesis, infarction may be seen on histopathologic examination of the brains of infants and children with congenital heart disease. Thromboembolic events related to cardiac catheterization, cardiac surgery, or endocarditis may cause focal infarction. Decreased cerebral perfusion, related to hypotension, hypoperfusion, or cardiac arrest, is associated with a diffuse pattern of cerebral injury (46). Kinney et al. (47) analyzed the neuropathology of 38 infants who died after undergoing reparative or palliative cardiac surgery. Although a spectrum of gray matter lesions was evident, cerebral white matter damage, composed of either periventricular leukomalacia or diffuse white matter gliosis, was the most significant finding. Neonates were more likely than infants to have periventricular leukoencephalopathy, reflecting the vulnerability of the immature (premyelinating) white matter to hypoxic–ischemic injury. Otherwise, the timing and type of surgery were unrelated to the pattern and severity of overall brain injury.
MRI allows the opportunity to evaluate cerebral structure and pathology in living patients, both preoperatively and postoperatively. In the preoperative period, McConnell et al. (48) found that one-third of infants undergoing cardiac surgery had ventriculomegaly and enlargement of subarachnoid spaces consistent with cerebral atrophy. The Total Maturation Scoring System developed by Childs to measure brain maturation in premature infants incorporates information related to myelination, cortical infolding, glial cell migration bands, and the presence of germinal matrix tissue (49). Licht et al. (50) using this score found neonates with HLHS and transposition of the great arteries (TGA) to have structural immaturity of the brain, on average delayed by 1 month, compared to healthy neonates (50). Utilizing this same measurement of brain maturation, Andropoulos et al. (51) demonstrated that postoperative neurologic injury as measured by MRI was greater in newborns undergoing cardiac surgery with less mature brain development. Mahle et al. (52) reported that among 24 infants undergoing cardiac surgery, preoperative MRI revealed periventricular leukoencephalopathy in 16% and infarct in 8%. Moreover, preoperative cerebral lactate peaks were elevated in more than half of infants evaluated by magnetic resonance spectroscopy. In the early postoperative period, 48% of infants had new periventricular leukoencephalopathy, 19% had new infarcts, and 33% had new parenchymal hemorrhage. Interestingly, on late postoperative MRI, performed in 17 infants, all previously detected periventricular leukoencephalopathy had resolved. However, cerebral atrophy was detected in two, old infarct in one, and new infarct in one subject. Similarly, Galli et al. (53) detected periventricular leukoencephalopathy in 54% of neonates and 4% of infants who underwent brain MRIs in the period between 6 and 14 days following open heart surgery. Recently, Beca et al. performed serial MRIs in young infants undergoing cardiac operations and found 20% to have white matter injury preoperatively. Postoperative MRI revealed new white matter injury in 44% of the cohort. Lower scores on developmental assessments were not associated with the presence of new postoperative white matter injury but did correlate with preoperative white matter injury and brain immaturity (54).
Changes in central nervous system structure may underlie neurocognitive abnormalities in children with congenital heart disease. In adolescents with TGA, regions of reduced white matter microstructure by fractional anisotropy were associated with worse cognitive performance in the expected patterns from previous neuroimaging work in other populations (55). For example, worse performance in math problem solving and numerical operations was correlated with reduced left parietal fractional anisotropy. In a mixed group of congenital heart disease adolescents, compared to controls, brain volume was reduced, and the extent of brain volume reduction was significantly associated with scores on tests of cognition, executive function, and motor function (56). While the predictive value of MRI findings on longer-term developmental outcome remains unclear, evidence is accumulating that white matter injury during fetal life and the perioperative period contributes to neurodevelopmental sequelae in children with congenital heart disease.
Perioperative Risk Factors
Prospective studies of central nervous system protection and injury have mainly focused on risk factors related to cardiac surgery and the perioperative period. The intense attention to perioperative risk factors is likely related to the ability of investigators to study the brain during this high-risk period, which includes planned brain ischemia–reperfusion injury with use of hypothermic cardiopulmonary bypass and total circulatory arrest techniques. Furthermore, perioperative management strategies can be tested in randomized clinical trials.
Perioperative Monitoring Approaches
As investigators have sought to optimize neurodevelopmental outcomes by modifying surgical and medical perioperative approaches, one limiting factor has been difficulty in identifying early predictive markers of longer-term developmental outcomes. To date, MRI has been performed primarily in a research setting. However, some centers have adopted perioperative monitoring strategies that include continuous electroencephalogram, near-infrared spectroscopy, and/or transcranial Doppler ultrasound (51,57,58). Clinical adoption of these monitoring techniques has outpaced establishment of definitive evidence for their clinical benefit. However, recently, Kussman et al. (59) found that among infants undergoing cardiac operation for biventricular repair, decreased cerebral oxygen delivery as measured by near-infrared spectroscopy during the perioperative period was associated with lower scores on the Psychomotor Development Index (PDI) and increased abnormalities found at brain MRI. Further study of this technique, other perioperative monitoring approaches and additional potential early markers are needed to better understand how late outcomes can be predicted in newborns and infants undergoing cardiac surgery. Nonetheless, a great deal has been learned since the 1990s related to perioperative risk factors of central nervous system insults for children with congenital heart disease.
Intraoperative Support Techniques
Repair of congenital heart disease commonly requires the use of cardiopulmonary bypass, in which blood is exposed to artificial surfaces. This may cause a systemic inflammatory response, with induction
of proinflammatory cytokines, chemokines, and endotoxin, as well as activation of the complement system, leukocytes, and the endothelium (60). Furthermore, cardiopulmonary bypass is accompanied by risks of gaseous and particulate embolism, macroemboli, and hypoperfusion resulting in diffuse ischemia/reperfusion injury (61).
of proinflammatory cytokines, chemokines, and endotoxin, as well as activation of the complement system, leukocytes, and the endothelium (60). Furthermore, cardiopulmonary bypass is accompanied by risks of gaseous and particulate embolism, macroemboli, and hypoperfusion resulting in diffuse ischemia/reperfusion injury (61).
During the most complex cardiac operations in the neonate, vital organ support is accomplished using deep hypothermia with low-flow continuous cardiopulmonary bypass (low-flow bypass) and/or deep hypothermic circulatory arrest (DHCA) (62,63,64). These techniques are often used sequentially in the same patient. Hypothermia is the principal technique used to protect vital organs during use of low-flow bypass or DHCA (65). Its effects are derived, in part, from a reduction in metabolic activity reflected in reduced oxygen consumption. Additional mechanisms of hypothermic protection of the brain and other organs during ischemia include preservation of intracellular stores of high-energy phosphates and of high intracellular pH, as well as protection against reperfusion injury including the no-reflow phenomenon, calcium influx, and free radical damage (65).
Circulatory arrest has been widely used since the 1960s in centers with expertise in infant open cardiac surgery. This technique has advantages for the surgeon of absence of perfusion cannulae and of blood from the operative field, though it may increase the risk for neurologic insult. In recent years, regional (antegrade) cerebral perfusion has been advocated to avoid exposure to DHCA (66,67) during neonatal aortic arch reconstruction. However, one single-center randomized trial did not show any benefit of regional cerebral perfusion, compared to DHCA, as a method of vital organ support during the Norwood operation with respect to developmental outcomes before Stage II surgery or at 1 year of age (68). In addition, using MRI, Dent et al. found that the rate of new periventricular leukomalacia in the postoperative period was not reduced in patients who underwent the Norwood operation using selective cerebral perfusion, compared to historical controls in whom a DHCA-only strategy had been employed (69). Most recently, Algra et al. also used MRI to measure postoperative brain injury and with a sequential analysis, found no benefit of antegrade cerebral perfusion over DHCA for neonates requiring aortic arch reconstruction (70).
When evaluated as a continuous variable, a longer duration of total circulatory arrest has been associated with increased risk of seizures, choreoathetosis, release of brain isoenzymes, and developmental delay (71,72,73,74,75,76,77,78,79,80,81) though in some studies, the duration of circulatory arrest has not been a significant predictor of outcome (68,82). The absence of an effect may be related, in part, to a narrow range of circulatory arrest times, small sample sizes, or overwhelming effects of other risk factors for adverse outcome, such as underlying genetic abnormalities or severe hemodynamic instability in the preoperative or postoperative period. However, Wypij et al. (83) analyzed the relationship between the duration of total circulatory arrest and neurodevelopmental outcome at age 8 years in infants with TGA who underwent the arterial switch operation in the neonatal period or early infancy. A nonlinear relationship was observed. Developmental scores did not decline until the duration of total DHCA exceeded a threshold of approximately 41 minutes (83). A universally “safe” duration of total circulatory arrest cannot be determined, however, because of its potential interaction with patient factors, such as age and a host of other perfusion variables that affect outcomes, including the depth of hypothermia (84), the rate and duration of core cooling (85), acid–base management during core cooling (86,87), and the degree of hemodilution (88).
The level of cerebral blood flow during cardiopulmonary bypass is affected by arterial carbon dioxide tension (PCO2) (89,90). In the alpha-stat strategy of pH management, arterial PCO2 is not corrected for the patient’s temperature during hypothermic cardiopulmonary bypass. Thus, patients are relatively alkalotic during hypothermia. In contrast, in the pH-stat strategy, CO2 is added to the bypass circuit to maintain a pH of 7.40 at the patient’s hypothermic temperature. Because higher arterial PCO2 is associated with greater cerebral blood flow, the pH-stat strategy may provide better brain protection during the periods of global brain hypoperfusion that occur during use of hypothermic cardiopulmonary bypass techniques (86,87,89,90).
Hemodilution during hypothermic cardiopulmonary bypass has also been studied with respect to its effects on brain injury during infant heart surgery. At the profoundly low temperatures (15° to 18°C) used during infant and neonatal cardiac surgery, hypothermia increases the viscosity of blood and red blood cell aggregation (91), potentially increasing the risk of microvascular occlusion. Hemodilution has been used to counter these risks (92) and has been shown to increase cerebral blood flow (93), but could reduce the oxygen-carrying capacity of blood. Furthermore, because hypothermia induces a leftward shift of oxyhemoglobin dissociation, hemodilution has the potential to limit oxygen delivery to the central nervous system (92). In a randomized single-center clinical trial of hemodilution strategy in infants undergoing reparative open heart surgery using hypothermic bypass techniques, low hematocrit (20%) at the onset of low-flow bypass was shown to be a risk factor for adverse neurodevelopmental outcome, specifically lower PDI score, at age 1 year (88). A subsequent trial showed no differences in neurodevelopmental outcome at 1 year with hemodilution within the range of 25% to 35% (94).
It is likely that factors such as hematocrit, temperature, pH strategy, and duration of circulatory arrest or very reduced flow interact in their effects on the central nervous system (95,96). For example, the reduced oxygen-carrying capacity of a low hematocrit during cardiopulmonary bypass can be compensated for by the use of the pH-stat strategy, by increasing flow rate, reducing the duration of circulatory arrest, or reducing temperature.
Preoperative Factors and Host Susceptibility
Host susceptibility is likely to affect the response of the central nervous system to cardiopulmonary bypass and perioperative events (97). Preoperative patient characteristics such as low Apgar scores at 5 minutes, younger gestational age, lower birth weight, and other attributes have been found to be independent risk factors for adverse neurodevelopmental outcomes (89,98). An increasing MRI literature suggests that brain immaturity at birth, reflecting impaired brain microstructure and metabolism during fetal life, is associated with greater risk of perioperative brain injury and also with adverse neurodevelopmental outcomes (54,99).
Furthermore, the response to cardiac surgery may be mediated by genetic polymorphisms in the pathways affected by exposure to cardiopulmonary bypass, including inflammation, thrombosis, vascular reactivity, and oxidative stress (100). Indeed, it is most likely that the effects of bypass on individual patients are mediated by many different genetic polymorphisms in the domains of inflammation, coagulation, and response to ischemia/reperfusion injury.
The influence of genetic polymorphisms on postoperative morbidity has been extensively studied among adults undergoing open heart surgery (101,102,103,104,105). For example, postoperative bleeding is more common among adults with polymorphisms in genes coding for coagulation proteins and platelet glycoproteins (105), while postoperative thrombotic complications have been associated with gene polymorphisms in fibrinogen and angiotensin-converting enzyme (106). Postoperative stroke has been found to be more common among patients with genetic variants for C-reactive protein (CRP) and interleukin-6 (IL-6) (107).
Gaynor et al. (108) examined the effect of Apolipoprotein E (APOE) genotype on neurodevelopmental outcomes in infants after cardiac surgery. The APOE ε2 allele was an independent risk factor for worse PDI scores in multivariable regression adjusting for
preoperative and postoperative covariates. Indeed, children with the APOE ε2 allele had PDI scores that were approximately one-half standard deviation (SD) lower than those with other genotypes. Of note, although an IQ deficit of approximately 0.5 SD is unlikely to be clinically significant for an individual child, when it represents a mean decline in a population its implications are substantial (109). The effect of APOE genotype was seen across the spectrum of children with and without genetic syndromes. The association of the APOE ε2 allele with worse neurodevelopmental outcomes after infant heart surgery was recently validated in infants with single ventricle enrolled in either the Infant with Single Ventricle or Single Ventricle Reconstruction trial (110). The adverse effect of APOE ε2 allele is most likely related to decreased neuroresiliency and impaired neuronal repair after central nervous system injury. Interestingly, this finding underscores that children and adults may differ with respect to the effects of particular genotypes. In contrast to infants, adults carrying the APOE ε4 allele who undergo open heart surgery have increased levels of biochemical markers of brain injury (111,112) and a greater rate of postoperative cognitive decline (113,114).
preoperative and postoperative covariates. Indeed, children with the APOE ε2 allele had PDI scores that were approximately one-half standard deviation (SD) lower than those with other genotypes. Of note, although an IQ deficit of approximately 0.5 SD is unlikely to be clinically significant for an individual child, when it represents a mean decline in a population its implications are substantial (109). The effect of APOE genotype was seen across the spectrum of children with and without genetic syndromes. The association of the APOE ε2 allele with worse neurodevelopmental outcomes after infant heart surgery was recently validated in infants with single ventricle enrolled in either the Infant with Single Ventricle or Single Ventricle Reconstruction trial (110). The adverse effect of APOE ε2 allele is most likely related to decreased neuroresiliency and impaired neuronal repair after central nervous system injury. Interestingly, this finding underscores that children and adults may differ with respect to the effects of particular genotypes. In contrast to infants, adults carrying the APOE ε4 allele who undergo open heart surgery have increased levels of biochemical markers of brain injury (111,112) and a greater rate of postoperative cognitive decline (113,114).
Postoperative Factors
Various risk factors for brain injury occur in the postoperative period. A low cardiac output syndrome is common in the first 24 to 48 hours following repair of complex congenital heart disease (115,116). Hemodynamic instability and low cardiac output syndrome may be especially damaging to the vulnerable central nervous system of the neonate who has just undergone cardiac surgery using deep hypothermic cardiopulmonary bypass techniques. Hypoxic–ischemic insult related to hypothermic cardiopulmonary bypass techniques disrupts the integrity of cerebral vasoregulatory systems in the early postoperative period, and autoregulation of cerebral blood flow is impaired (117,118,119,120). Persistence of such disturbances in cerebrovascular control renders the brain vulnerable to subsequent insults, such as hypotension or hypoxia. Galli et al. (53) reported that hypotension and hypoxemia are independent risk factors for periventricular leukomalacia, and found such injury to occur in 50% of neonates undergoing open heart surgery. Similarly, Demitropoulos et al. confirmed the association of newly acquired postoperative brain injury on MRI with lower postoperative systolic and mean blood pressures (99).
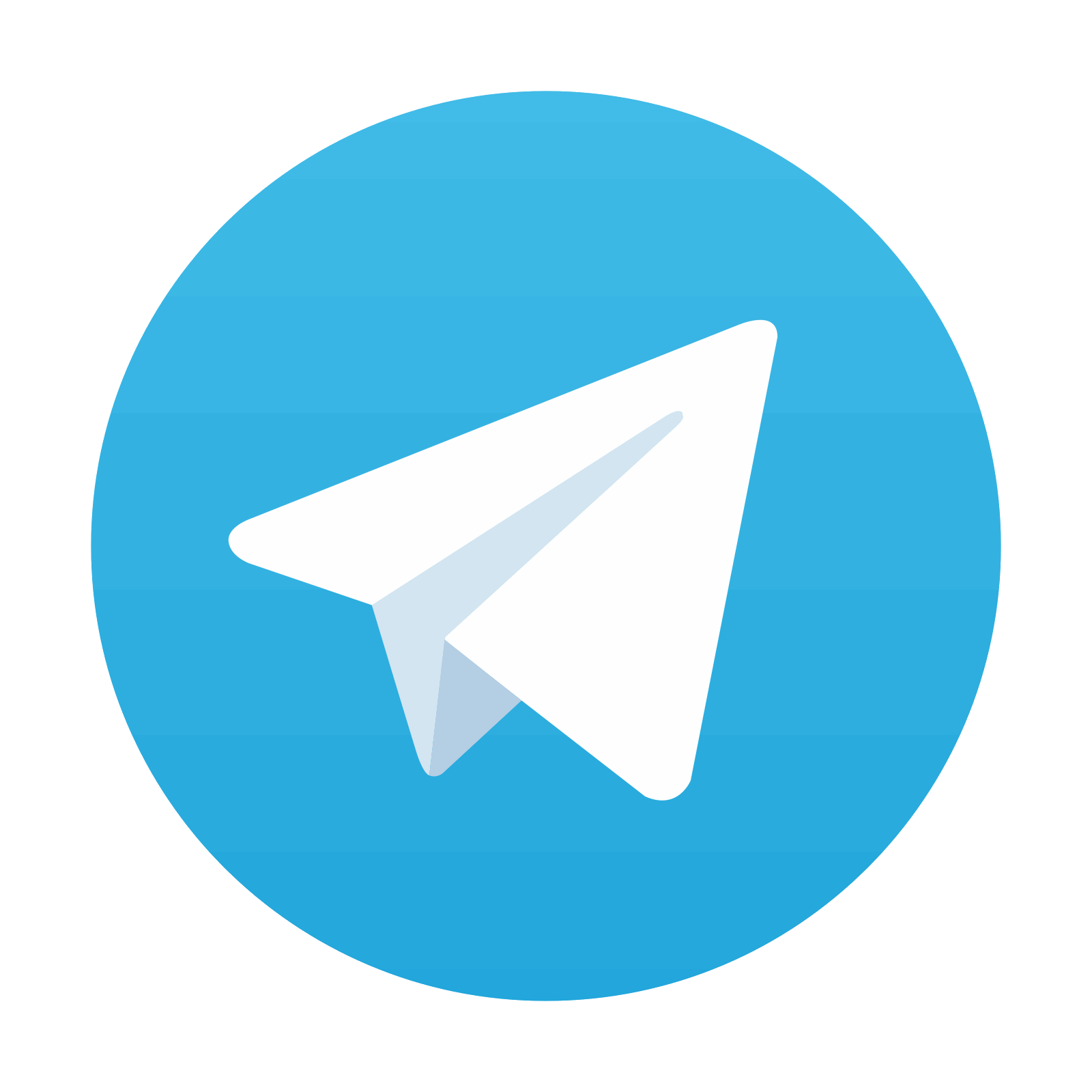
Stay updated, free articles. Join our Telegram channel

Full access? Get Clinical Tree
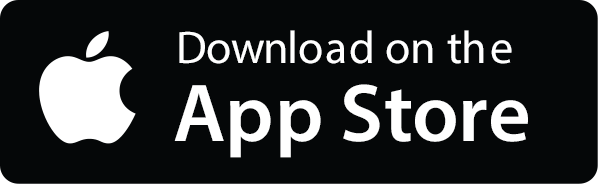
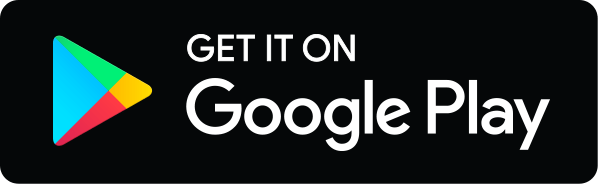