Myocyte Structure and Function
Arnold M. Katz
What the Cardiac Myocyte Does
The pumping of the heart is made possible by interactions between contractile proteins that transform the chemical energy derived from adenosine triphosphate (ATP) into mechanical work. These interactions are activated by a process called excitation–contraction coupling, in which plasma membrane depolarization causes ionized calcium (Ca2+) to be released into the cytosol, where this activator can bind to the contractile machinery. The heart relaxes when this Ca2+ is pumped out of the cytosol. Ca2+ fluxes into the cytosol, which are passive (downhill), are mediated by Ca2+ channels, whereas relaxation is an active (uphill) process that occurs when ion pumps and exchangers transport Ca2+ out of the cytosol. This chapter describes the mechanisms responsible for excitation–contraction coupling, contraction, and relaxation by the working cells of the atria and ventricles, and some of the cytoskeletal proteins that help maintain cell architecture and participate in cell signaling (for general reviews, see references 1,2,3,4).
Myocyte Structure
The adult human heart contains working myocardial cells in the atria and ventricles, which are specialized for contraction (Fig. 98.1), nodal cells in the sinoatrial and atrioventricular nodes that are responsible for pacemaker activity and atrioventricular conduction, respectively, and Purkinje fibers that effect rapid conduction throughout the ventricles. All form a functional syncytium in which cells are linked electrically and mechanically by intercalated discs. The latter contain low-resistance gap junction channels made up of connexin molecules that allow electrical current to flow freely between the interiors of adjacent cardiac myocytes (5,6).
Specialized Myocytes
The sinoatrial and atrioventricular nodes are made up of small nodal cells rich in glycogen and contain few contractile filaments; they conduct slowly because their action potentials depend on Ca2+ channels (1,7). His-Purkinje cells, which are specialized for rapid conduction, are large pale cells that, like nodal cells, contain more glycogen and fewer contractile filaments than the cells of the working myocardium. These cells make up the atrioventricular bundle and bundle branches, and course along the ventricular endocardium where they synchronize ventricular systole. Fast conduction by the His-Purkinje cells is made possible by the many large Na+ channels in their plasma membranes (1,7). Transition cells, intermediate in appearance between the His-Purkinje cells and working myocardial cells, are found in both the atria and the ventricles.
Working Myocardium
Working myocardial cells are filled with cross-striated myofibrils (Fig. 98.2). Their contractile activity is regulated by the plasma membrane, which separates the cytosol from the
extracellular space, and by the internal membranes of the sarcoplasmic reticulum. Mitochondria, which are responsible for aerobic metabolism and oxidative phosphorylation, generate ATP.
extracellular space, and by the internal membranes of the sarcoplasmic reticulum. Mitochondria, which are responsible for aerobic metabolism and oxidative phosphorylation, generate ATP.
Membrane Structures
The plasma membrane, which separates the cytosol from the extracellular space, includes the sarcolemma that surrounds the cell, and a transverse tubular (t-tubular) system that penetrates the cell interior. The t-tubules, whose lumens open to the extracellular space, rapidly transmit action potentials to the cell interior. Dyads, which are composite structures formed by the plasma membrane and sarcoplasmic reticulum (Fig. 98.3), regulate the release of activator Ca2+ during excitation–contraction coupling.
The heart’s sarcoplasmic reticulum, which is a specialization of the endoplasmic reticulum and so is sometimes called the sarco(endo)plasmic reticulum, takes up, stores, and
releases Ca2+. This internal membrane system includes the subsarcolemmal cisternae and sarcotubular network. The former contains Ca2+-binding proteins that store this activator cation and Ca2+ release channels that release Ca2+ into the cytosol to initiate systole. The sarcotubular network, which surrounds the contractile proteins, contains a densely packed array of Ca2+ pump adenosine triphosphatase (ATPase) proteins that relax the heart by transporting Ca2+ out of the cytosol into the lumen of the sarcoplasmic reticulum.
releases Ca2+. This internal membrane system includes the subsarcolemmal cisternae and sarcotubular network. The former contains Ca2+-binding proteins that store this activator cation and Ca2+ release channels that release Ca2+ into the cytosol to initiate systole. The sarcotubular network, which surrounds the contractile proteins, contains a densely packed array of Ca2+ pump adenosine triphosphatase (ATPase) proteins that relax the heart by transporting Ca2+ out of the cytosol into the lumen of the sarcoplasmic reticulum.
Myofibrils
The contractile proteins are organized in darkly staining A bands and lightly staining I bands (see Fig. 98.2). Each I band is bisected by a Z line that contains a number of cytoskeletal proteins (see below). The sarcomere, which is the morphologic unit of muscle, lies between two Z lines and consists of a central A band and two adjacent half I bands.
TABLE 98.1 Contractile Proteins of the Heart | |||||||||||||||||||||
---|---|---|---|---|---|---|---|---|---|---|---|---|---|---|---|---|---|---|---|---|---|
|
The A bands contain thick filaments, whereas the half I bands at either side of the A band contain only thin filaments. The thick filaments are composed largely of myosin, and the thin filaments are actin polymers that include the regulatory proteins tropomyosin and the troponin complex. At physiologic sarcomere lengths in the resting heart, the thin filaments extend from the Z lines almost to the center of the A band, so that as the muscle shortens the extent of overlap between the thick and thin filaments increases. At short sarcomere lengths, there is double overlap between the thin filaments from the two adjacent I bands in the center of the sarcomere.
Sarcomere shortening occurs when the thin filaments are pulled toward the center of the A band by motion of the myosin cross-bridges, which project from the thick filaments to establish links with adjacent thin filaments. Like the oars of a racing shell, cross-bridge motion sweeps the thin filaments toward the center of the sarcomere in a reaction that is energized by ATP hydrolysis.
Myocyte Function
Contractile Proteins
Myocardial contraction and its control can be understood in terms of the interactions between six myofibrillar proteins (8) that hydrolyze ATP, undergo physicochemical changes that develop tension and cause shortening, and are controlled by Ca2+ in a manner that mimics physiologic excitation–contraction coupling (Table 98.1).
Myosin
Myosin, the major protein of the thick filament, is a large, elongated molecule with a filamentous “tail” and a globular “head” (Fig. 98.4); the latter makes up the cross-bridges that interact with actin to release the chemical energy that is used for contraction. Each myosin molecule contains two heavy chains and four light chains. The heavy chains extend into the cross-bridge and contain the sites responsible for both ATPase activity and the ability to interact with actin, whereas the light chains serve a regulatory function. Myosin heavy chains and light chains differ in different muscle types, different regions of the heart, and even adjacent cells.
Myosin forms aggregates in the thick filaments in which the tails are wound together to form a backbone and the heads project as cross-bridges. The cross-bridges in resting muscle are perpendicular to the long axis of the thick filament, whereas in active muscle their tips interact with actin in reactions that “row” the thin filaments toward the center of the sarcomere (Fig. 98.5).
Myosin heavy chains are the major determinant of muscle-shortening velocity and myocardial contractility. A higher
ATPase myosin heavy chain isoform (called α) is associated with more rapid shortening velocity, higher contractility, and more efficient contraction against light loads, whereas hearts containing a lower ATPase myosin heavy chain isoform (called β) have a lower contractility but contract more efficiently at heavy loads. In the normal human heart, atrial myosin heavy chains are mostly a high-ATPase isoform, whereas the ventricle contains only a small amount of the fast myosin heavy chain.
ATPase myosin heavy chain isoform (called α) is associated with more rapid shortening velocity, higher contractility, and more efficient contraction against light loads, whereas hearts containing a lower ATPase myosin heavy chain isoform (called β) have a lower contractility but contract more efficiently at heavy loads. In the normal human heart, atrial myosin heavy chains are mostly a high-ATPase isoform, whereas the ventricle contains only a small amount of the fast myosin heavy chain.
Actin
Actin is a globular protein that forms a double-stranded macromolecular helix which serves as the backbone of the thin filament (Fig. 98.6). Human hearts contain mainly α-cardiac actin, along with a smaller amount of α-skeletal actin.
Tropomyosin
Tropomyosin, an elongated molecule made up of two α-helical peptide chains, is present in the heart as two isoforms, α and β. This protein is found in the thin filament and in the longitudinal grooves between the two strands of actin (see Fig. 98.6), where it regulates the interactions between myosin, actin, and the troponin complex.
Troponin Complex
The troponin complex is made up of three proteins (Figs. 98.6 and 98.7). Troponin I, along with tropomyosin, reversibly inhibits the interactions between actin and myosin; troponin T binds the troponin complex to tropomyosin; and troponin C contains the high-affinity Ca2+-binding sites that recognize the appearance of Ca2+ in the cytosol as a signal to initiate contraction.
Troponin C is a member of a family of Ca2+-binding proteins that includes the myosin light chains and calmodulin, an intracellular Ca2+-mediated regulator. Troponin I, which inhibits actin–myosin interactions in resting muscle, allows actin to interact with the myosin cross-bridges when Ca2+ is bound to troponin C. Phosphorylation of cardiac troponin I by cyclic adenosine monophosphate–dependent protein kinase (PK-A) reduces the Ca2+ sensitivity of troponin C, which contributes to the lusitropic effect of β-adrenergic stimulation. Isoform switches in troponin T, which binds the troponin complex to tropomyosin, modify the Ca2+ sensitivity of tension development in failing hearts.
Regulation of Contractile Protein Interactions
The major physiologic regulator of the contractile protein interactions is Ca2+ released into the cytosol during excitation–contraction coupling. In resting muscle, where cytosolic Ca2+ concentration is low, actin–myosin interactions are inhibited by tropomyosin and the troponin complex (see Fig. 98.7). Ca2+ binding to troponin C initiates contraction by reversing this inhibitory effect, which occurs when cooperative interactions among the regulatory proteins in the thin filament shift the position of tropomyosin in the grooves between the double-stranded F-actin polymer. The muscle returns to its resting state when a fall in cytosolic Ca2+ dissociates this activator from troponin C, which returns tropomyosin to its inhibitory position in the thin filament.
Contractile protein interactions are also regulated by preload and afterload. The former, which allows end-diastolic
volume to regulate cardiac performance (Starling’s Law of the Heart), is due mainly to sarcomere length–dependent changes in the Ca2+ sensitivity of the contractile proteins and Ca2+ release from the sarcoplasmic reticulum (9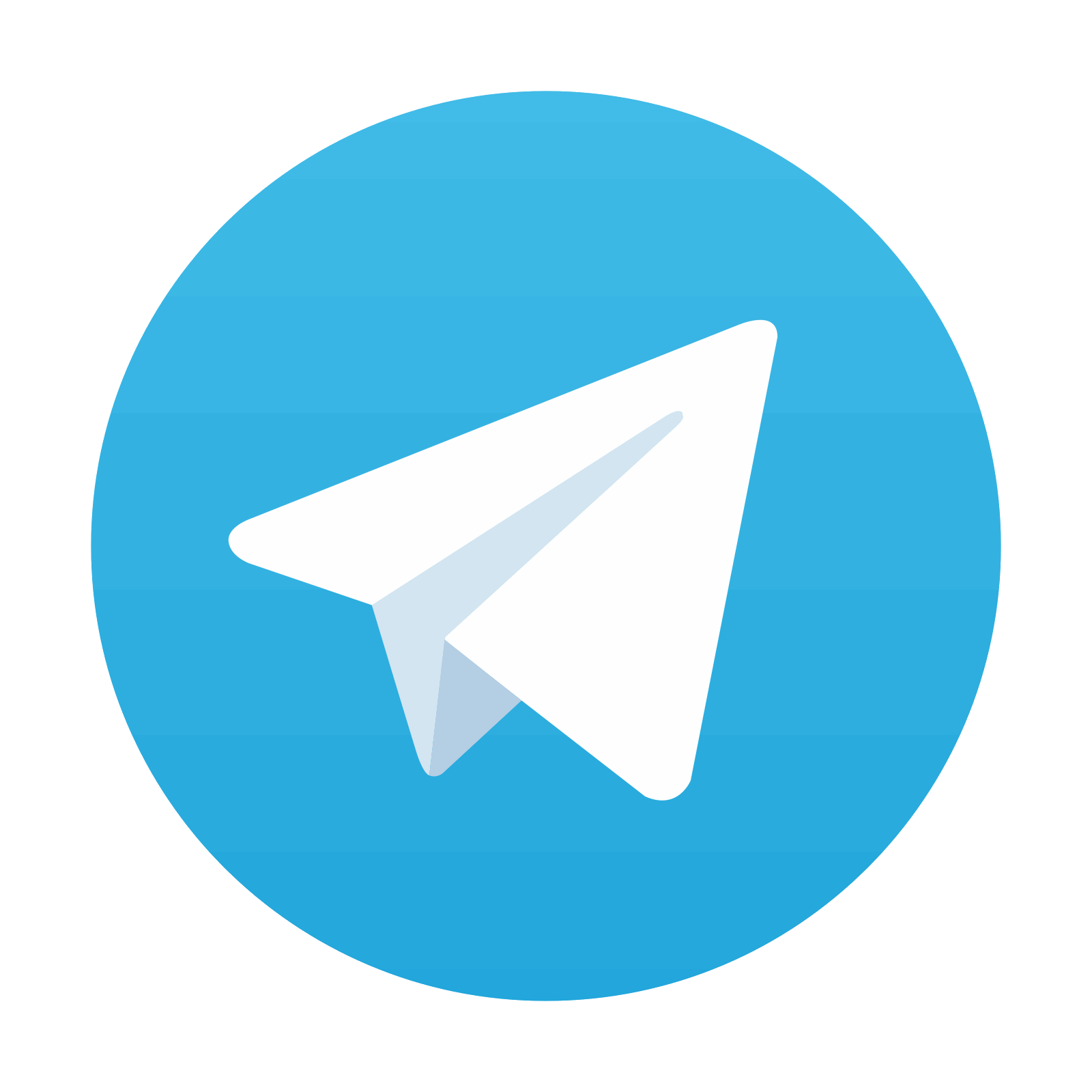
volume to regulate cardiac performance (Starling’s Law of the Heart), is due mainly to sarcomere length–dependent changes in the Ca2+ sensitivity of the contractile proteins and Ca2+ release from the sarcoplasmic reticulum (9
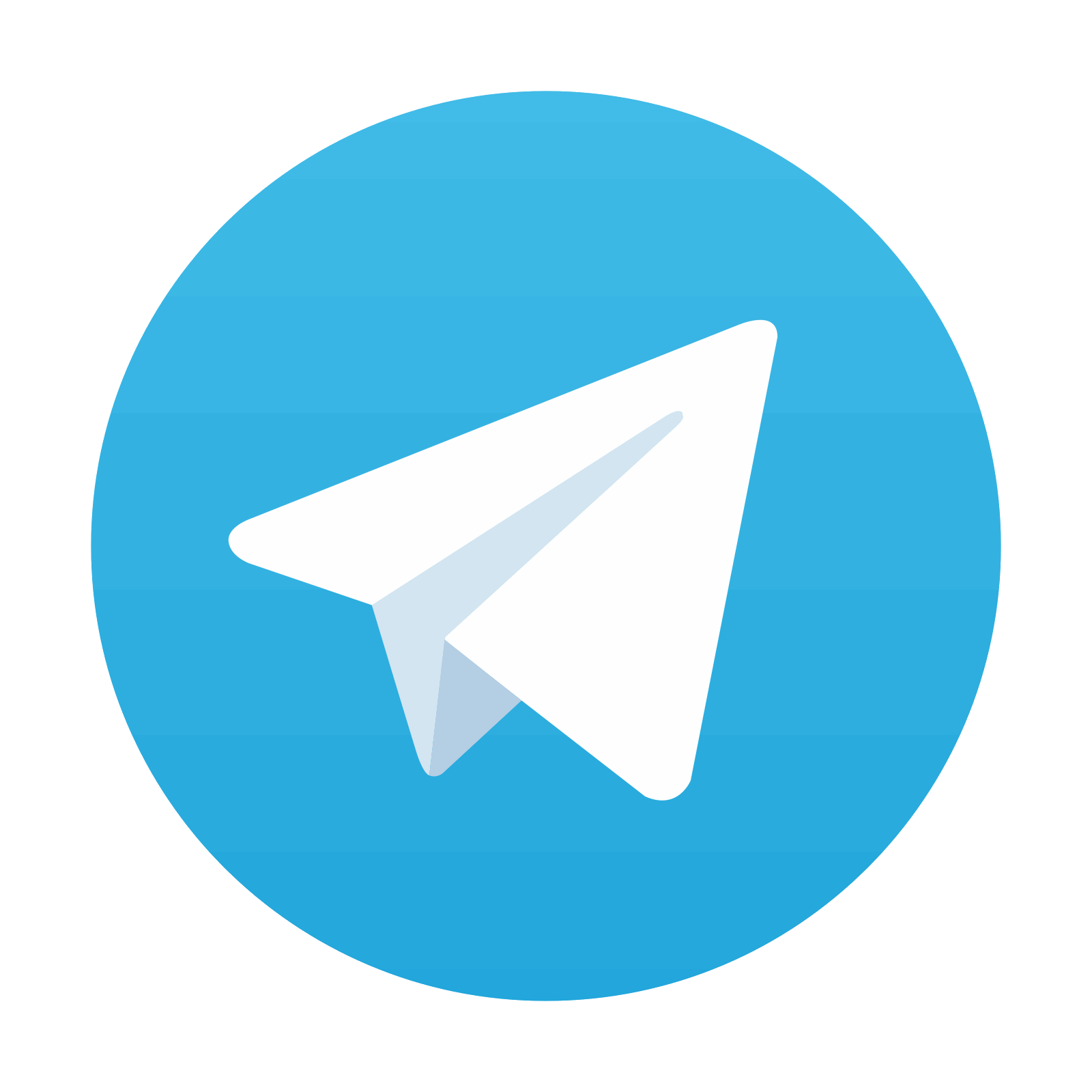
Stay updated, free articles. Join our Telegram channel

Full access? Get Clinical Tree
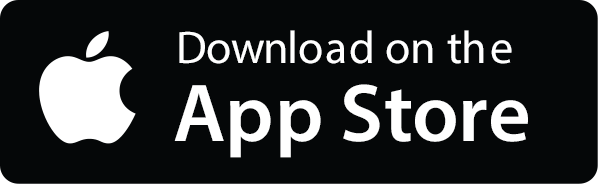
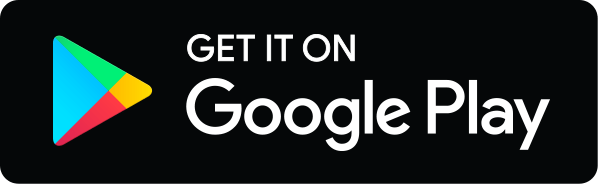
