Background
The investigators compared pulsed-wave tissue Doppler (PWTD) and color tissue Doppler (CTD) ventricular tissue velocities obtained using Vivid 7 (GE) and iE33 (Philips) ultrasound systems within a 30-minute time frame and examined interobserver and intraobserver variability.
Methods
Longitudinal PWTD and CTD images were prospectively acquired in 49 children from the base of the right and left ventricular free wall and septum and stored for offline analysis.
Results
Intraobserver and interobserver coefficients of variation for PWTD velocities ranged from 4% to 6% and 5% to 10% for GE and from 1% to 10% and 2% to 14% for Philips, respectively. Intraobserver and interobserver coefficients of variation for CTD velocities ranged from 4% to 15% and 6% to 24% for GE and from 6% to 19% and 7% to 25% for Philips, respectively. Mean biases between the two systems for PWTD velocities, expressed as percentage differences, were around 0%, with the narrowest limits of agreement for systolic velocities at the septal annulus and the widest limits of agreement for early diastolic velocities at the lateral tricuspid valve annulus, respectively. Peak CTD-derived velocities were significantly and consistently lower for Philips compared with GE.
Conclusions
Agreement between GE-derived and Philips-derived PWTD velocities was reasonable, whereas CTD-derived velocities differed substantially.
Doppler tissue velocity imaging (TVI) to measure the velocity of myocardial contraction and relaxation is widely applied in the evaluation of systolic and diastolic ventricular function. TVI has been used to noninvasively estimate left ventricular filling pressure, to determine annular displacement, and to time cardiac events. Currently available TVI modalities are spectral or pulsed-wave tissue Doppler (PWTD) and color tissue Doppler (CTD). PWTD velocities can be measured instantaneously at the bedside, whereas CTD-derived TVI requires offline postprocessing of the stored data, using dedicated analysis software. Another difference is that PWTD detects the frequency shift between the emitted and returning ultrasound signals and measures peak myocardial velocities, whereas CTD uses phase-shift analysis with autocorrelation techniques and provides mean myocardial velocities. Consequently, CTD myocardial velocities are lower than PWTD velocities, and the two are therefore not interchangeable.
Normal PWTD and CTD reference values have been published for the fetal, pediatric, and adult population, and several studies have reported the important clinical relevance of abnormal TVI-derived findings in these age groups. Not surprisingly, PWTD and CTD are now an integrated software application in most commercially available ultrasound systems. This raises the question of whether measurements obtained by different ultrasound equipments are truly comparable, thus allowing different systems to be used interchangeably. To date, studies comparing myocardial tissue velocities among different ultrasound systems and software analysis packages are largely missing. Whether tissue velocity measurements are truly comparable among ultrasound systems has important clinical implications, for example, for the serial assessment of cardiac function and the interpretation of published TVI reference data that have been obtained with different ultrasound equipment and software. The aim of this study in children was therefore to compare the agreement between the two most widely used ultrasound systems in measuring longitudinal myocardial Doppler velocities by PWTD and CTD.
Methods
The institutional research ethics board approved this prospective study, and written informed consent was obtained from each participant and/or their parents before enrollment. A total of 49 children with and without heart disease were enrolled. Excluded from participation were patients with functional single ventricular physiology, unstable hemodynamics, and cardiac arrhythmias.
Echocardiographic Examinations
Echocardiographic examinations were performed using Vivid 7 (GE Medical Systems, Wauwatosa, WI) and iE33 (Philips Medical Systems, Andover, MA) ultrasound systems. For GE, we used either an S4 or an S5 curved-array transducer and for Philips an S5 curved-array probe. Image acquisition was performed by the same experienced sonographer (C.S.) within a 30-minute time frame. Each participant first underwent an extensive initial assessment of the anatomy and the cardiac function with one ultrasound system and subsequently a less extensive assessment with the other ultrasound system. The primary choice of ultrasound equipment depended on the indication for the exam. The GE system was the first choice to evaluate children with cardiomyopathy and children without heart disease. The Philips system was preferred for the baseline assessment of children with known structural heart disease. Baseline echocardiography included M-mode recordings from the parasternal long and short axes to calculate ejection time, cardiac cycle length, left and right ventricular dimensions, and shortening fraction.
PWTD
Spectral Doppler tissue images were obtained from the apical cardiac four-chamber view at a sweep speed of 50 to 100 mm/sec. A 5 × 5 mm Doppler sample volume was placed within the center of the myocardial wall just distal to the mitral annulus in the left ventricular lateral and septal walls and just distal to the tricuspid annulus in the right ventricular free wall. The ultrasound beam was aligned as parallel as possible (<30°) to the direction of the longitudinal wall motion. No angle correction was used. The sector width was reduced if necessary to obtain high–frame rate images. Gain settings and saturation levels were adjusted to avoid aliasing, to eliminate background noise, and to obtain clear PWTD velocity tracings ( Figures 1 A and 1 B). Systolic (S′) and diastolic (E′ and A′) velocities were measured offline from three consecutive cardiac cycles, using EchoPAC version 7 (GE Medical Systems) and Xcelera version 2009 (Philips Medical Systems). PWTD measurements were done by two different observers (by C.S. for GE and by L.P.K. for Philips).

CTD
CTD images of three cardiac cycles of the left free wall, septum, and right free wall were acquired and stored for offline analysis, using the same frame rates (>130 frames/sec). CTD velocities were measured using EchoPAC and QLAB version 7.0 (Philips Medical Systems). For GE, a 5 × 5 mm sample volume was placed just below the valvar annulus (same locations as for PWTD). For Philips, a 10-mm M-line was placed at the same locations as for GE, without using the track-all button (which automatically keeps the M-line at the same region of the myocardium throughout the cardiac cycle). The default filter settings were used to obtain smooth velocity curves for both systems (see Figures 1 C and 1 D for examples). CTD measurements were done by two different observers (C.S. for GE and L.P.K. for Philips).
The angle of insonation was measured offline using SyngoDynamics version 5.1 (Siemens Medical Solutions Health Services, Malvern, PA) for both GE and Philips images.
Reproducibility of Measurements
Interobserver and intraobserver variability in offline measurements was assessed in 15 randomly selected studies representing children with and without cardiac disease. TVI velocities were measured by two independent observers and subsequently ≥2 weeks later by one of the observers to avoid recall bias. C.S. performed the interobserver measurements for Philips, and L.P.K. performed the interobserver measurements for GE. Each observer was blinded to the results of the other observer.
Statistical Analysis
Data are presented as mean ± SD, ranges, and frequencies, as appropriate. PWTD and CTD velocities from three consecutive cardiac cycles were averaged. Agreement between machines, software, and Doppler modalities for continuous variables was calculated using Bland-Altman statistics. This included the calculation of mean bias (average difference between measurements), the statistical significance of the mean bias using a paired t test (the null hypothesis was zero bias), and the limits of agreement (95% confidence interval). For all Bland-Altman plots, the percentage difference (the difference between two measurements divided by the average of the two measurements times 100) were used. Pearson’s correlation and its statistical significance as measures of raw associations between measurements were calculated. Interobserver and intraobserver agreement was calculated using the same methodology and by calculating the coefficient of variation (the standard deviation of the difference of the paired samples divided by the average of the paired samples).
To investigate whether the difference in myocardial velocities between GE and Philips was related to the difference in frame rate between the systems, univariate linear regression models using maximum likelihood estimates for parameter determination were used. These models used the difference of a given velocity parameter between GE and Philips as the dependent variable and the difference in frame rate between GE and Philips as the independent variable. A similar approach was used to assess the influence of the absolute myocardial velocity on the difference in myocardial velocity between GE and Philips (the difference of a given velocity parameter between GE and Philips was the dependent variable, and the average of myocardial velocity by GE and Philips was the independent variable). All statistical analyses were performed using SAS version 9.1 (SAS Institute Inc., Cary, NC).
Results
Table 1 shows the baseline clinical and echocardiographic characteristics of the study cohort. Of the five children with a cardiomyopathy, three had dilated cardiomyopathy and two had hypertrophic cardiomyopathy. Diagnosis of congenital heart disease ( n = 21) included tetralogy of Fallot ( n = 5), ventricular septal defects ( n = 6), left ventricular outflow tract obstructions ( n = 7), Ebstein’s anomaly of the tricuspid valve ( n = 1), pulmonary stenosis ( n = 1), and transposition of the great arteries ( n = 1), and all lesions were surgically corrected. The majority of the participants had normal shortening fractions and left ventricular dimensions.
Variable | Value |
---|---|
Age (years) | 12.4 ± 4 (4.4 to 17.7) |
Male | 31 (63%) |
Weight (kg) | 42 ± 16 (16.4 to 73.8) |
Height (cm) | 146 ± 19 (105 to 171) |
BSA (m 2 ) | 1.29 ± 0.3 (0.69 to 1.76) |
BMI (kg/m 2 ) | 19 ± 4 (12 to 30) |
Systolic blood pressure (mm Hg) | 102 ± 13 (81 to 114) |
Diastolic blood pressure (mm Hg) | 57 ± 9 (40 to 61) |
Diagnosis | |
Normal heart | 12 (24%) |
Heart transplant recipient | 6 (12%) |
Systemic arterial hypertension | 3 (6%) |
Cardiomyopathy | 5 (10%) |
Kawasaki disease | 1 (2%) |
Anthracycline treatment | 1 (2%) |
Congenital heart disease | 21 (43%) |
Heart rate (beats/min) | 72 ± 15 (41 to 119) |
LV EDD Z score | 0.73 ± 1.61 (−3.2 to 6.4) |
LV ESD Z score | 0.40 ± 1.56 (−3.3 to 6.9) |
Shortening fraction (%) | 37 ± 6 (23 to 53) |
Left ventricular ejection time (msec) | 296 ± 44 (201 to 458) |
Feasibility of Image Acquisition and Reproducibility
The total time for offline analysis of PWTD and CTD velocities was approximately 10 minutes for both GE and Philips. A detailed comparison between the two ultrasound systems in image acquisition is shown in Table 2 . Heart rate and angle of insonation were similar, but frame rates were significantly higher with the Philips system. Measurements of PWTD velocity were obtained in 94% for E′ and A′ velocities and in 100% for S′ velocities for both systems. Measurements of CTD velocity were achieved in 96% to 100% for GE and 76% to 96% for Philips. The main reason for the inability to measure diastolic CTD velocities was the inability to define two separate diastolic waves (because of either fusion of the E′ and A′ waves or the identification of only one diastolic wave). The results for intraobserver and interobserver variability are shown in Tables 3 and 4 . Overall, the average intraobserver and interobserver bias for PWTD-derived velocities was close to zero, indicating no systemic bias, and the coefficient of variations were <15% for both ultrasound systems. For CTD velocities by Philips, for most parameters, the mean bias was also small, although there seemed to be a trend such that the observer performing the interobserver variability assessment (C.S.) measured CTD velocities slightly lower compared with the observer who performed the measurements for all participants (L.P.K.) (see Table 4 , bias calculated as value by C.S. minus value by L.P.K.). Intraobserver and interobserver coefficients of variation for CTD-derived velocities ranged from 4% to 24% for GE and from 6% to 25% for Philips.
GE | Philips | ||
---|---|---|---|
Variable | ( n = 49) | ( n = 49) | p |
Frame rate (frames/sec) | |||
Mitral valve free wall | 171 ± 36 | 227 ± 49 | <.001 |
Mitral valve septum | 171 ± 35 | 230 ± 50 | <.001 |
Tricuspid valve free wall | 176 ± 37 | 225 ± 52 | <.001 |
Heart rate (beats/min) | |||
Mitral valve free wall | 70 ± 16 | 72 ± 15 | .07 |
Mitral valve septum | 70 ± 16 | 72 ± 15 | .21 |
Tricuspid valve free wall | 71 ± 16 | 69 ± 18 | .35 |
Angle of insonation (°) | |||
Mitral valve free wall | 23.3 ± 6.6 | 21.9 ± 6.0 | .11 |
Mitral valve septum | 11.7 ± 6.4 | 11.3 ± 6.4 | .61 |
Tricuspid valve free wall | 20.7 ± 11.1 | 20.4 ± 9.8 | .76 |
General Electric | Philips | |||||||||
---|---|---|---|---|---|---|---|---|---|---|
Measurement | Bias (cm/sec) | LCL (cm/sec) | UCL (cm/sec) | Pearson’s R | COV (%) | Bias (cm/sec) | LCL (cm/sec) | UCL (cm/sec) | Pearson’s R | COV (%) |
Intraobserver | ||||||||||
Mitral valve free wall E′ | −0.31 | −1.74 | 1.13 | 0.97 | 4.1 | 0.19 | −0.50 | 0.88 | 0.99 | 2.0 |
Mitral valve free wall A′ | −0.06 | −0.82 | 0.71 | 0.98 | 6.1 | 0.11 ‡ | −0.23 | 0.45 | 1.00 | 3.5 |
Mitral valve free wall S′ | −0.08 | −0.82 | 0.66 | 0.98 | 3.8 | 0.04 | −0.40 | 0.49 | 0.99 | 2.0 |
Mitral valve septal E′ | −0.01 | −1.26 | 1.24 | 0.98 | 4.6 | 0.16 ‡ | −0.24 | 0.55 | 1.00 | 1.4 |
Mitral valve septal A′ | −0.18 | −0.87 | 0.52 | 0.96 | 5.5 | 0.08 | −0.58 | 0.74 | 0.96 | 5.3 |
Mitral valve septal S′ | −0.27 ‡ | −0.98 | 0.44 | 0.93 | 4.5 | 0.09 | −1.53 | 1.70 | 0.84 | 10.0 |
Tricuspid valve free wall E′ | −0.13 | −1.39 | 1.13 | 0.97 | 4.5 | 0.55 ‡ | −0.95 | 2.05 | 0.96 | 5.8 |
Tricuspid valve free wall A′ | 0.08 | −0.75 | 0.91 | 0.99 | 4.9 | 0.08 | −0.94 | 1.10 | 0.97 | 6.8 |
Tricuspid valve free wall S′ | 0.28 | −1.12 | 1.68 | 0.97 | 5.8 | −0.03 | −0.54 | 0.48 | 0.99 | 2.4 |
Interobserver | ||||||||||
Mitral valve free wall E′ | −0.85 | −2.65 | 0.95 | 0.96 | 5.1 | 1.03 ∗ | −0.78 | 2.84 | 0.98 | 4.9 |
Mitral valve free wall A′ | −0.41 | −1.53 | 0.71 | 0.95 | 9.7 | 0.41 † | −0.66 | 1.47 | 0.95 | 8.6 |
Mitral valve free wall S′ | 0.69 | −3.37 | 4.75 | 0.78 | 9.8 | 0.11 | −0.72 | 0.93 | 0.98 | 3.9 |
Mitral valve septal E′ | −0.54 | −2.46 | 1.37 | 0.93 | 7.3 | 0.43 ∗ | −0.12 | 0.97 | 0.99 | 2.1 |
Mitral valve septal A′ | −0.33 | −1.38 | 0.72 | 0.94 | 7.9 | 0.23 ∗ | −0.17 | 0.63 | 0.99 | 3.5 |
Mitral valve septal S′ | 0.01 | −1.26 | 1.28 | 0.75 | 7.4 | 0.14 | −1.93 | 2.21 | 0.70 | 13.0 |
Tricuspid valve free wall E′ | −0.14 | −2.39 | 2.12 | 0.87 | 7.7 | 1.04 ‡ | −1.76 | 3.84 | 0.93 | 10.0 |
Tricuspid valve free wall A′ | −0.31 | −1.40 | 0.78 | 0.98 | 6.0 | 0.18 | −1.86 | 2.22 | 0.87 | 14.0 |
Tricuspid valve free wall S′ | 0.43 | −1.38 | 2.24 | 0.95 | 7.3 | −0.05 | −0.85 | 0.75 | 0.98 | 3.1 |
General Electric | Philips | |||||||||
---|---|---|---|---|---|---|---|---|---|---|
Measurement | Bias (cm/sec) | LCL (cm/sec) | UCL (cm/sec) | Pearson’s R | COV (%) | Bias (cm/sec) | LCL (cm/sec) | UCL (cm/sec) | Pearson’s R | COV (%) |
Intraobserver | ||||||||||
Mitral valve free wall E′ | 0.07 | −2.21 | 2.36 | 0.92 | 8.8 | −0.07 | −1.40 | 1.27 | 0.89 | 6.7 |
Mitral valve free wall A′ | 0.12 | −0.88 | 1.12 | 0.95 | 14.1 | 0.08 | −0.39 | 0.55 | 0.96 | 19.4 |
Mitral valve free wall S′ | 0.06 | −1.85 | 1.97 | 0.89 | 13.8 | −0.05 | −0.75 | 0.65 | 0.95 | 14.2 |
Mitral valve septal E′ | 0.13 | −0.71 | 0.98 | 0.98 | 4.0 | 0.11 | −1.36 | 1.59 | 0.93 | 5.7 |
Mitral valve septal A′ | −0.06 | −1.17 | 1.04 | 0.90 | 14.7 | 0.54 ‡ | −1.23 | 2.31 | 0.70 | 12.8 |
Mitral valve septal S′ | −0.13 | −0.82 | 0.56 | 0.93 | 6.1 | 0.15 | −1.10 | 1.40 | 0.85 | 10.5 |
Tricuspid valve free wall E′ | −0.13 | −1.24 | 0.97 | 0.96 | 5.3 | −0.10 | −0.94 | 0.74 | 0.97 | 6.8 |
Tricuspid valve free wall A′ | −0.13 | −1.36 | 1.10 | 0.96 | 11.7 | 0.08 | −0.56 | 0.72 | 0.98 | 18.9 |
Tricuspid valve free wall S′ | −0.02 | −0.70 | 0.66 | 0.99 | 3.6 | −0.18 | −0.98 | 0.62 | 0.97 | 12.8 |
Interobserver | ||||||||||
Mitral valve free wall E′ | 0.39 | −1.40 | 2.19 | 0.95 | 7.8 | −1.51 ∗ | −4.27 | 1.24 | 0.50 | 17.4 |
Mitral valve free wall A′ | 0.16 | −1.28 | 1.60 | 0.90 | 8.5 | −0.35 † | −1.18 | 0.47 | 0.84 | 15.7 |
Mitral valve free wall S′ | 0.11 | −1.83 | 2.05 | 0.89 | 7.5 | −0.50 ∗ | −1.34 | 0.34 | 0.94 | 9.6 |
Mitral valve septal E′ | −0.08 | −1.18 | 1.02 | 0.97 | 7.7 | −0.62 | −3.17 | 1.93 | 0.83 | 14.2 |
Mitral valve septal A′ | 0.01 | −0.88 | 0.91 | 0.93 | 24.2 | 0.33 | −1.48 | 2.13 | 0.66 | 25.2 |
Mitral valve septal S′ | −0.11 | −1.25 | 1.02 | 0.80 | 13.8 | 0.19 | −1.03 | 1.40 | 0.84 | 13.4 |
Tricuspid valve free wall E′ | −0.45 ‡ | −1.78 | 0.87 | 0.95 | 5.5 | −0.41 ‡ | −1.72 | 0.90 | 0.91 | 8.7 |
Tricuspid valve free wall A′ | −0.36 | −2.32 | 1.60 | 0.90 | 8.7 | −0.07 | −2.00 | 1.87 | 0.75 | 24.2 |
Tricuspid valve free wall S′ | −0.21 | −2.65 | 2.24 | 0.90 | 5.9 | −0.33 | −1.34 | 0.68 | 0.96 | 7.3 |
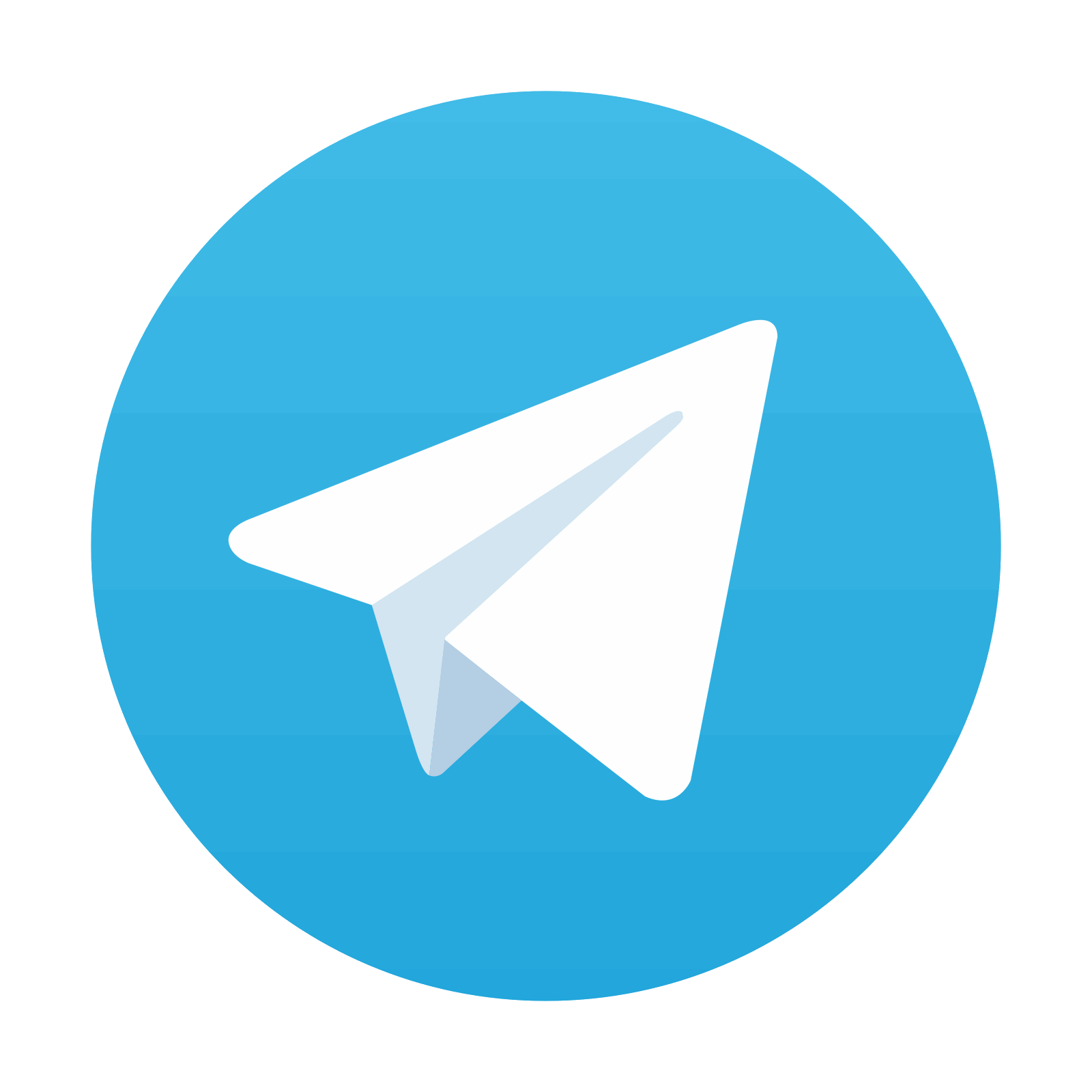
Stay updated, free articles. Join our Telegram channel

Full access? Get Clinical Tree
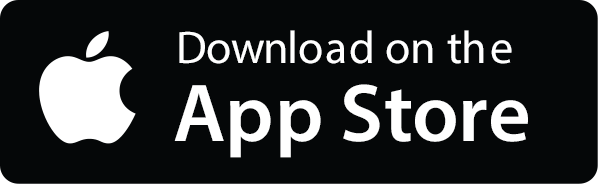
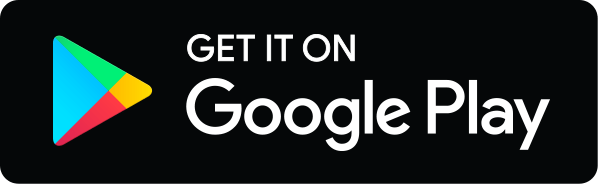
