Background
Standard marathon running can result in a depression of left ventricular (LV) diastolic function during early recovery. Left atrial (LA) mechanics are integral in maintaining an early diastolic pressure gradient as well as being responsive to changes in LV diastolic function, and therefore the detailed assessment of LA mechanics may provide further insight. The aim of this study was to determine the impact of prolonged strenuous exercise on mechanics of the left atrium and the association with changes in LV diastolic function.
Methods
Myocardial speckle-tracking echocardiograms of the left atrium and left ventricle were obtained prior to, immediately after, and 6 hours after the completion of a marathon (42.2 km) in 17 healthy adult men. Speckle tracking was used to determine peak atrial deformation, early diastolic deformation rate, and contractile function, including atrial activation time. LA volumes throughout the cardiac cycle were also assessed to provide reservoir, conduit, and booster pump volumes. Diastolic assessment of the left ventricle included peak early diastolic strain rate, late diastolic strain rate, and standard indices. Temporal assessment of LV “twist” and “untwist” was also evaluated.
Results
All 17 subjects completed the marathon (mean finishing time, 209 ± 19 minutes; range, 173-241 minutes). Although contractile function was significantly increased, there was a reduction in early diastolic deformation rate that was correlated with reduced atrial deformation. Atrial activation time was significantly increased after the race. All LV indices of diastolic function were reduced in early diastole, whereas late diastolic function was increased after the race. LV torsion was significantly reduced at end-systole and significantly elevated in the isovolumic period and early diastole, after the race. All indices returned toward baseline at 6 hours after exercise.
Conclusions
This study demonstrates transient changes in LV diastolic relaxation following prolonged exercise that appear to have a direct impact on subsequent LA deformation. The impact of reduced LA preload on these findings and the delay in LA activation time requires further exploration.
There is growing evidence of a reduction in left ventricular (LV) systolic and diastolic function during recovery from prolonged exercise. A recent meta-analysis suggested that postexercise changes in LV diastolic function were consistent across exercise mode and duration. Whether the alteration in LV diastolic filling is due to reduced preload or to changes in intrinsic myocardial function is controversial. Surprisingly, little attention has been paid to the role of the left atrium during recovery from prolonged exercise and whether this is influenced by changes in LV diastolic filling.
During systole, the left atrium acts as a reservoir, dependent on myocardial compliance, preload, and descent of the LV base, while in early diastole, it acts as a conduit delivering blood into the left ventricle while “pulling” blood from the pulmonary veins. In late diastole, the left atrium acts as a contractile unit to “top up” LV volume. Diastolic filling is a complex interaction involving the effects of preload, afterload, and myocardial function on both the left atrium and left ventricle. The rate and volume of flow in early diastole are driven by the rate of LV relaxation and “untwist” in conjunction with the rise and maintenance of pressure within the left atrium. The development of this pressure gradient between the left atrium and left ventricle during diastole is therefore an important determinant of early diastolic function. Although the contribution of LV relaxation and motion to this process has been assessed, the interrogation of left atrial (LA) mechanics in response to prolonged exercise would provide additional insight and direction in this complex topic.
To the best of our knowledge, no studies have comprehensively assessed LA mechanics following prolonged strenuous exercise. In one study, the indirect assessment of the left atrium, via pulmonary venous flow interrogation, suggested reduced suction through the atrial conduit after prolonged exercise, but this could reflect changes in LA mechanics, an alteration in LV performance, or a combination of both. LV performance following prolonged strenuous exercise has previously been assessed using myocardial or two-dimensional (2D) speckle tracking. This has allowed myocardial strain (ϵ) and strain rate (SR) to be measured in circumferential, radial, and longitudinal planes and has facilitated the measurement of LV rotation and torsion. Recently, speckle tracking of the left atrium in providing deformation data was reported to be feasible and reproducible, and thus its application in studies of prolonged exercise may be insightful.
In view of (1) evidence of a consistent depression in diastolic function during recovery from marathon running, (2) evidence of the importance of LA function in LV relaxation and filling, and (3) the recent development of 2D speckle tracking to assess ϵ and SR in the left atrium, the primary aim of this study was to assess LA mechanics during recovery from prolonged exercise. A secondary aim was to investigate the relationship between changes in LV diastolic function and alterations in LA structure or ϵ and SR immediately and 6 hours after the completion of a marathon race. These broad aims allowed the following hypothesis to be assessed: a transient depression in LV diastolic filling following prolonged strenuous exercise is temporally associated with transient changes in LA mechanics.
Methods
Subjects
Seventeen male recreational runners (mean age, 33 ± 6 years; range, 26-44 years) volunteered to participate in the study and run a marathon (42.2 km). All subjects were healthy and free from known cardiovascular disease and any early family history of cardiovascular disease and were not currently taking any form of prescribed medication. All subjects provided written informed consent to participate, and the study was granted ethics approval by the ethics committee of the Brompton, Harefield and the National Heart and Lung Institute.
Design
Echocardiography was performed approximately 24 hours prior to starting the marathon. Postrace echocardiographic studies were completed within 1 hour of race completion, and a further acquisition was made 6 hours after marathon completion. All subjects refrained from training for 48 hours and avoided alcohol and caffeine 24 hours prior to the race. During the marathon, participants were permitted to consume food and fluid ad libitum. On the day of the marathon, start times ranged from 5 to 7 am . Maximum air temperature reached 10°C, with approximately 40% humidity at sea level.
Procedures
After a full explanation of procedures, subjects lay supine, and after a 5-minute resting period, the echocardiographic examination was initiated. Heart rate was taken from electrocardiography, inherent to the ultrasound system. All echocardiographic images were acquired using a commercially available ultrasound system (Vivid 7; GE Vingmed Ultrasound AS, Horten, Norway) with a 1.5-MHz to 4-MHz phased-array transducer. All images were acquired by a single experienced sonographer with the subject lying in the left lateral decubitus position and recorded to DVD in a raw Digital Imaging and Communications in Medicine format. Acquisition and analysis were performed by the same single experienced sonographer. All data were analyzed offline using commercially available software (EchoPAC version 6.0; GE Vingmed Ultrasound AS), and a minimum of 3 cardiac cycles were averaged for all peak indices.
Conventional Echocardiography
Standard 2D, pulsed-wave Doppler, and pulsed tissue Doppler echocardiographic parameters were obtained from parasternal and apical acoustic windows. All settings were optimized to obtain maximum signal-to-noise ratio and 2D images to provide optimal endocardial delineation. LV end-diastolic dimension, LV end-diastolic volume, LV end-systolic volume, LA anterior-posterior diameter, and LA volume at end-systole (LAES) were measured in accordance with recommendations from the American Society of Echocardiography. LA volume was also measured prior to atrial contraction and at end-diastole (LAED). From these data, LA reservoir volume was calculated as LAES − LAED, LA conduit volume as the difference between LV stroke volume and LA reservoir volume, and LA booster pump volume as LA volume prior to atrial contraction − LAED, as previously described. All volumes were derived using Simpson’s biplane methodology, and LV ejection fraction was calculated. LV diastolic function was assessed using pulsed-wave Doppler recordings from apical 4-chamber and 3-chamber orientations. For the assessment of diastolic function, a 4-mm sample volume was placed at the tips of the mitral leaflets in diastole, and transmitral flow was acquired to obtain peak early (E) and atrial (A) flow velocities. To assess isovolumic relaxation time, the 4-mm pulsed-wave sample was positioned between the LV inflow and outflow from an apical 3-chamber orientation. For the tissue Doppler assessment of E′, the apical 4-chamber orientation was used, and a 2-mm sample volume was positioned at the septal aspect of the mitral valve annulus, ensuring the best alignment between wall motion and the ultrasound beam. The high-pass filter was bypassed, and gains were set to minimal values to obtain the best signal-to-noise ratio. The Nyquist limit was set between 10 and 35 cm/s. Peak early diastolic (E′) tissue myocardial velocity was recorded and the E/E′ ratio calculated. Right ventricular (RV) function was assessed using 2D images to provide a RV fractional area change (RVFAC).
Two-Dimensional Myocardial Speckle Tracking
For the acquisition of LA functional data, the apical 4-chamber orientation was used, with the region of interest placed around the endocardial surface of the atrial lateral wall, superior wall, and atrial septum (see Figure 1 ). The image was optimized with the focal point positioned at the mid atrial wall, and frame rates were maintained as high as possible, but no greater than 90 frames/s. Offline analysis allowed the assessment of peak data of the left atrium and was assessed from the lateral wall, only providing indices of deformation during ventricular systole (Aϵ and ASRs), during ventricular diastole (ASRe), and during atrial contraction (ASRa) (see Figures 2 and 3 ). Atrial activation time (Aact) was also measured and defined as the time from the onset of the electrocardiographic P wave to the onset of mechanical atrial contraction.



LV radial and circumferential ϵ and SR and rotational data were derived from a parasternal short-axis view at the base, defined as at the tips of the mitral valve. The calculation of torsion also required the acquisition of an apical short-axis view at the level immediately above the level of systolic cavity obliteration. The focal point was positioned close to the center of the LV cavity to provide optimal beam width while reducing the effects of divergence. Longitudinal ϵ and SR data were obtained from an apical 4-chamber view only. The focal point was positioned at the level of the mitral valve. In both orientations, frame rates were maximized with an acceptable level >40 and <90 frames/s. All images were optimized with gain, compression, and dynamic range to enhance myocardial definition, while depth, frequency, and insonation angle were standardized from all acquisitions to reduce the impact of variability.
For the left ventricle, parasternal short-axis segments provided data from the inferoseptum, anteroseptum, and anterior, lateral, posterior, and inferior walls. The apical 4-chamber orientation provided data from the basal, mid, and apical septum and basal, mid, and apical lateral walls. All myocardial segments were considered acceptable and included in the analysis. Peak rotation and rotation rate were obtained, and torsion was calculated as the net difference between apical and basal rotation. Peak LV circumferential, radial, and longitudinal diastolic function was assessed from early diastolic SR (LVSRe) and late diastolic SR (LVSRa) directly from the EchoPAC software. A number of data points, dependent on the maximum frame rate of the 2D image, from LV rotation and torsion and ϵ curves were stored from the offline workstation and exported to a spreadsheet (Excel 2007; Microsoft Corporation, Redmond, WA). The spreadsheet was then used to select data points from each subject in 10% increments of both systole and diastole, essentially providing 20 data points from each cardiac cycle. The mean values of all 20 data points from the 17 subjects were then calculated, allowing the graphical representation of the complete cardiac cycle, before, immediately after, and 6 hours after the marathon.
Previous data collected in our laboratory on 20 subjects from two separate acquisitions 2 days apart revealed coefficients of variation for peak atrial ϵ, ASRe, ASRa, and Aact of 8.9%, 7.3%, 6.2%, and 3.4% respectively. The coefficients of variation for peak LV radial, circumferential, and longitudinal peak early diastolic LVSRe were 5.3%, 7.1%, and 10.1% and for late diastolic LVSRa were 4.5%, 6.7%, and 8.1%, respectively.
Statistical Analysis
Echocardiographic data related to LA and LV structure and function assessed before, immediately after, and 6 hours after exercise were analyzed using repeated-measures analysis of variance and post hoc Tukey tests as appropriate. For LA and LV functional indices that were significantly altered after the race, bivariate correlational analysis was used to establish any relationship to exercise-related changes in heart rate and preload (end-diastolic volume). Further changes in LV function were correlated with changes in LA mechanics.
Results
All 17 subjects completed the marathon (mean finishing time, 209 ± 19 minutes; range, 173-241 minutes). Postrace body mass was reduced (80 ± 9.2 to 78.8 ± 8.6 kg, P < .001), but heart rate was increased (57 ± 8 to 80 ± 12 beats/min, P < .001) and remained elevated 6 hours after the marathon (68 ± 11 beats/min, P < .001). LV end-diastolic volume was reduced after the race but did not reach statistical significance (154 ± 30 to 142 ± 33 mL, P = .07; 146 ± 32 mL at 6 hours).
Conventional Echocardiography
All standard 2D and Doppler findings are presented in Table 1 . Ejection fraction was not significantly altered after the race. Transmitral E/A ratio was reduced by 35% after the race as a consequence of reduction in peak E velocity and a compensatory increase in peak A velocity. The ratio had not completely returned to baseline at 6 hours. There was no significant change in the isovolumic relaxation time or early diastolic deceleration time. Early diastolic annular velocity (E′) decreased slightly ( P < .05), and thus E/E′ was also unchanged. RVFAC was reduced by 9% after the race but had returned to the baseline level at 6 hours following race completion.
Parameter | Before exercise | Immediately after exercise | 6 hours after exercise |
---|---|---|---|
Heart rate (beats/min) | 57 (53-62) | 80 (74-86) ∗ † | 68 (62-73) |
E (m/s) | 0.75 (0.68-0.83) | 0.70 (0.65-0.75) † | 0.76 (0.7-0.82) |
A (m/s) | 0.46 (0.4-0.51) | 0.67 (0.58-0.76) ∗ † | 0.54 (0.48-0.60) |
E/A | 1.7 (1.5-1.9) | 1.1 (0.9-1.3) ∗ † | 1.5 (1.3-1.7) |
IVRT (ms) | 76 (66-86) | 74 (64-82) | 66 (57-75) |
LVd (mm) | 54 (52-56) | 52 (50-55) ∗ | 53 (51-55) |
EDV (mL) | 154 (139-170) | 142 (125-159) | 147 (130-163) |
ESV (mL) | 52 (46-59) | 53 (45-61) | 51 (45-57) |
EF (%) | 66 (64-68) | 63 (60-65) | 65 (63-67) |
E′ (cm/s) | 13 (11 to15) | 11 (10-13) ∗ | 12 (11-14) |
E/E′ | 6.2 (5.4-7.1) | 6.6 (5.5-7.7) | 6.3 (5.7-6.9) |
LAd (mm) | 40 (38-42) | 38 (36-41) ∗ | 40 (37-42) |
RVFAC (%) | 49 (46-52) | 44 (41-49) ∗ | 47 (43-52) |
∗ P < .05, immediately after versus before exercise.
LA Deformation and Volumes
Peak Aϵ and peak ASRe were reduced after the race (17% and 26%, respectively), with both variables returning to baseline at 6 hours after exercise. There was a 10% increase in atrial contractility rate immediately after the race, which returned to baseline at 6 hours after exercise. The 38% increase in Aact after the race remained present at 6 hours after the race. LA anterior-posterior diameter, LAES, and LAED were significantly reduced after the race (5%, 13%, and 5%, respectively) but had returned to baseline levels at 6 hours after the race. Reservoir and conduit volume also reduced after the race by 18% and 11%, respectively, while atrial booster pump volume increased by 40% (see Table 2 ).
Parameter | Before exercise | Immediately after exercise | 6 hours after exercise |
---|---|---|---|
Aact (TS-onset) (ms) | 33.5 (25.6 to 41.4) | 53.5 (42.4 to 64.6) ∗ | 51.1 (41.5 to 60.8) † |
Aact (TS-peak) (ms) | 108.8 (97.9 to 119.7) | 118.3 (106.3 to 130.2) | 114.2 (104.7 to 123.6) |
Aϵ (%) | 53.1 (42.8 to 63.2) | 44.2 (37.1 to 51.1) ∗ | 51.7 (43.1 to 60.4) † |
ASRs (L/s) | 2.79 (2.35 to 3.25) | 2.62 (2.15 to 3.09) | 2.77 (2.24 to 3.3) |
ASRe (L/s) | −4.37 (−5.12 to −3.63) | −3.24 (−3.84 to −2.64) ∗ | −3.90 (−4.66 to −3.14) |
ASRa (L/s) | −2.95 (−3.56 to −2.33) | −3.24 (−4.02 to −2.54) ∗ | −2.88 (−3.66 to −2.10) |
LAES (mL) | 65 (43 to 79) | 57 (43 to 76) ∗ | 67 (45 to 85) ‡ |
LApreA (mL) | 47 (32 to 64) | 49 (37 to 65) | 49 (35 to 67) |
LAED (mL) | 37 (26 to 48) | 35 (27 to 46) ∗ | 38 (28 to 48) ‡ |
Reservoir volume (mL) | 28 (16 to 37) | 23 (10 to 47) ∗ | 29 (14 to 47) ‡ |
Conduit volume (mL) | 74 (50 to 112) | 66 (36 to 103) ∗ | 66 (35 to 103) |
Booster volume (mL) | 10 (5 to 17) | 14 (4 to 29) ∗ | 11 (4 to 25) |
∗ P < .05, immediately after versus before exercise.
† P < .05, before versus 6 hours after exercise.
LV Torsion and ϵ Diastolic Function
Torsion remained significantly elevated in early diastole (20%, 30%, and 40% of the diastolic interval) when postrace data were compared with prerace, data suggesting a reduced rate of diastolic untwisting. This was confirmed by basal and apical LV rotation data, which were still elevated during early diastole after the race (see Figure 4 ). All values returned close to baseline at 6 hours after the race.

Changes in peak LV ϵ and SR data (see Table 3 ) provide support for the torsion and untwist data. Peak LVSRe was reduced in all planes of relaxation. Circumferential, radial, and longitudinal SRe/SRa was significantly reduced after the race as a result of reduced LVSRe and an increased LVSRa. Peak circumferential and longitudinal early diastolic SR occurred 10% later in diastole immediately after the race. At 6 hours after the race, this had returned to baseline. Regional data for radial and circumferential SRe/SRa were reduced from all myocardial segments, but longitudinal SRe was significantly reduced in the septum but not the lateral wall. Interestingly, SRa increased in both septal and lateral walls, causing a concomitant reduction in SRe/SRa at both sites after the race.
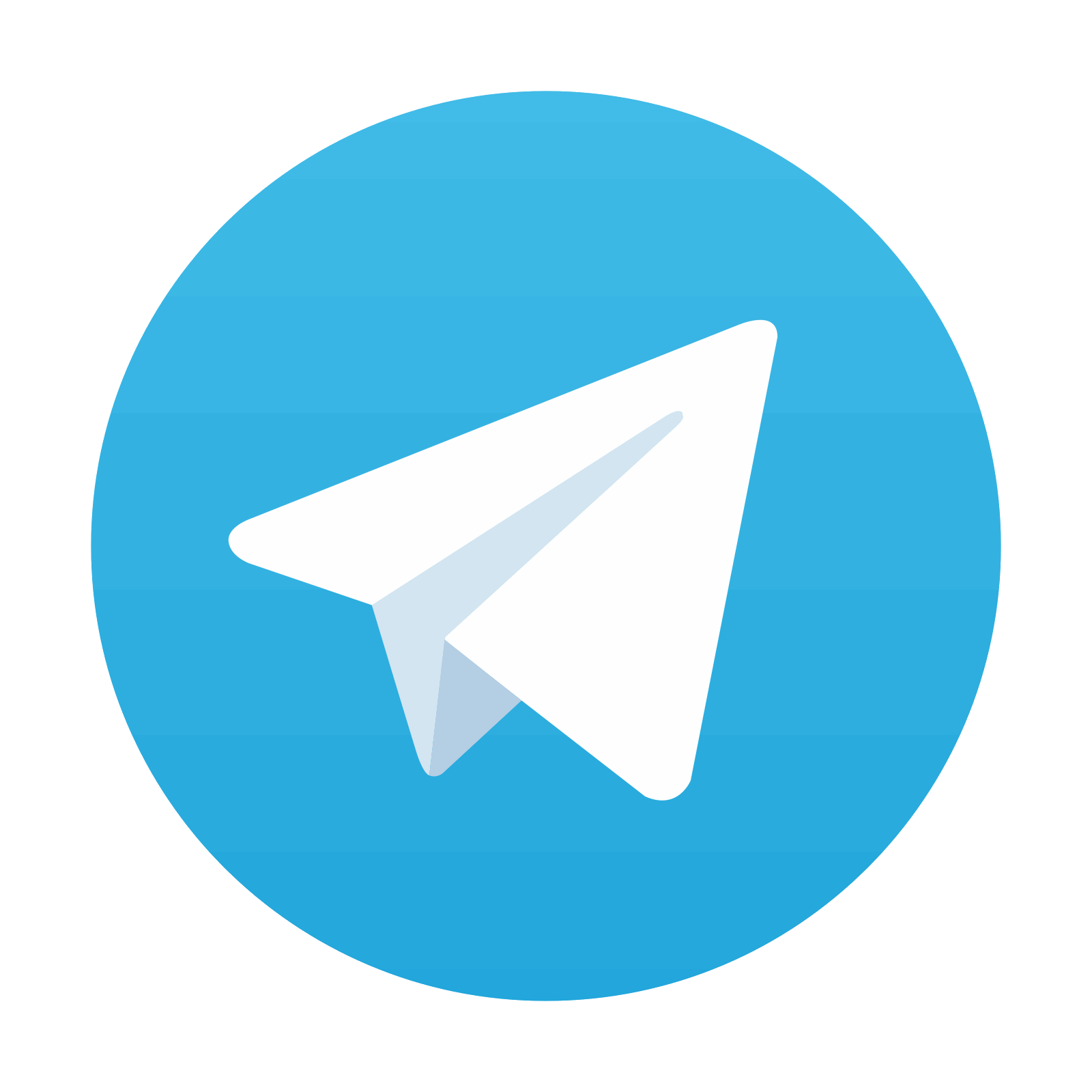
Stay updated, free articles. Join our Telegram channel

Full access? Get Clinical Tree
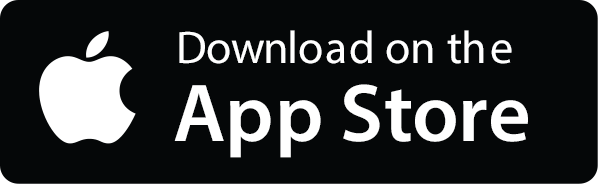
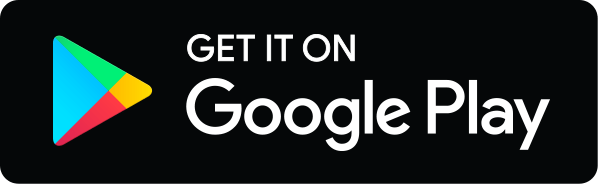
