TABLE 29.1. Biology of myocardial protection | ||||||||||||||||||||||||||||||||||||
---|---|---|---|---|---|---|---|---|---|---|---|---|---|---|---|---|---|---|---|---|---|---|---|---|---|---|---|---|---|---|---|---|---|---|---|---|
|
between species and is thought to be due to upregulation of 5′-adenosine monophosphate-activated protein kinase (9). During this period of development, the neonatal myocardium shows a progressive decline in glucose uptake which can be stimulated by insulin, that is, insulin resistance and a much greater capacity to store glycogen (10). The greater ability of the immature myocardium to utilize anaerobic glycolysis may partially account for the greater tolerance to ischemia. Laboratory and clinical studies in adults have found that enhanced glucose uptake and oxidation is associated with enhanced functional myocardial recovery, despite normalization of fatty acid oxidation (6).
TABLE 29.2. Physiologic differences between pediatric and adult myocardium and the potential impact of these differences on ischemia tolerance of the pediatric heart | ||||||||||||||||||||||||||||||||||||||||||||||||
---|---|---|---|---|---|---|---|---|---|---|---|---|---|---|---|---|---|---|---|---|---|---|---|---|---|---|---|---|---|---|---|---|---|---|---|---|---|---|---|---|---|---|---|---|---|---|---|---|
|
tissue per minute) averaged 6.7 mL in the working state (adult 8 mL), 3.2 mL in the empty beating state (adult 5.6 mL), 1.3 mL in the potassium-arrested heart at 37°C (adult 1.1 mL), 0.37 mL in the hypothermic (15°C) heart, and 0.32 mL in the hypothermic (15°C) potassium-arrested heart (43,44).
(and pulmonary) function as a result of ischemia-reperfusion injury and the associated endothelial dysfunction (49). In neonatal lambs, the addition of L-arginine (NO precursor) or nitroglycerine (NO donor) during reperfusion resulted in significantly higher preload recruitable stroke work and cardiac index (50). A clinical practice at Boston Children’s Hospital is to start a nitroglycerine infusion (1 µg/kg/min) 5 to 10 minutes before removal of the aortic cross-clamp and continue it until the end of rewarming.
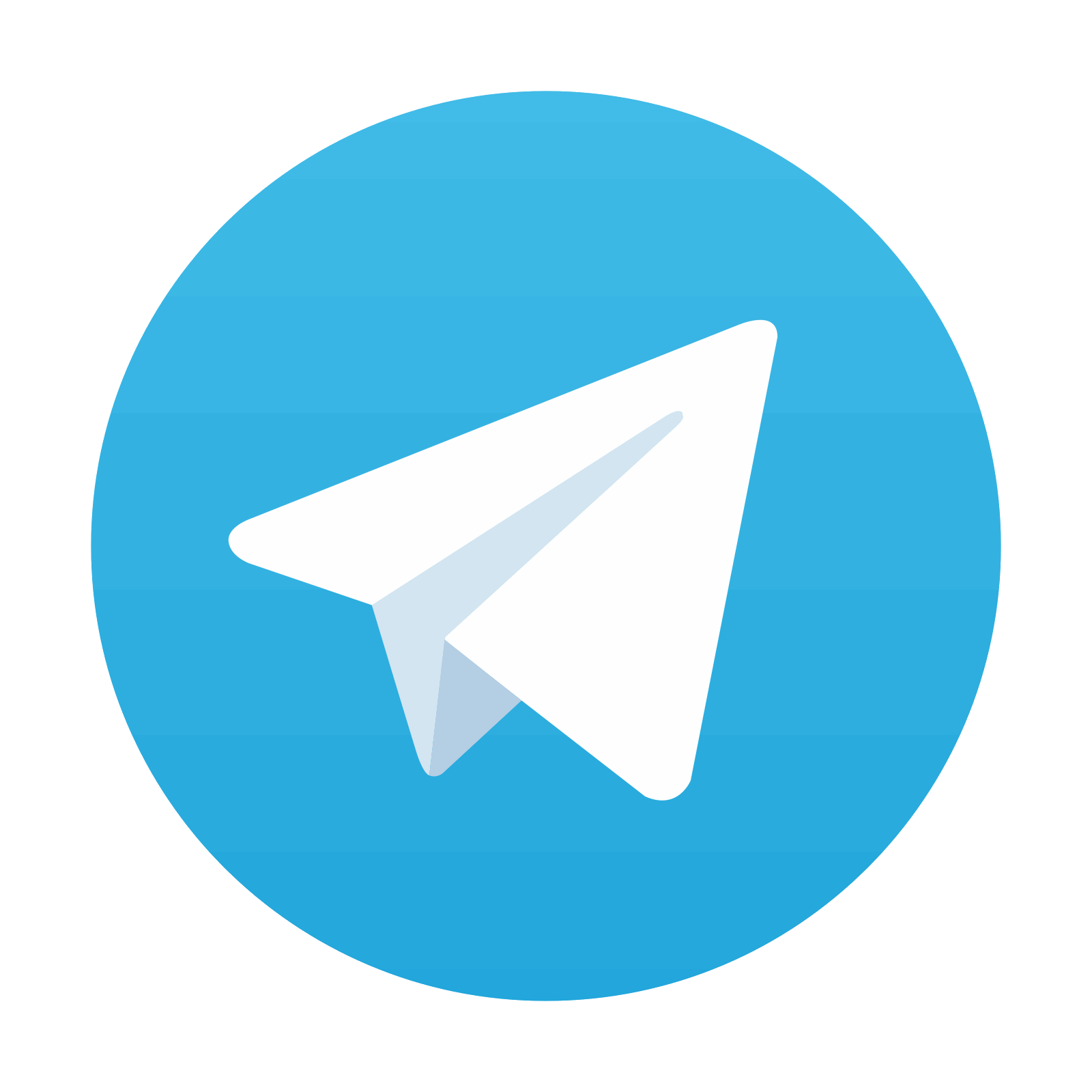
Stay updated, free articles. Join our Telegram channel

Full access? Get Clinical Tree
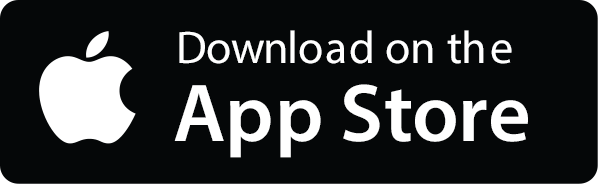
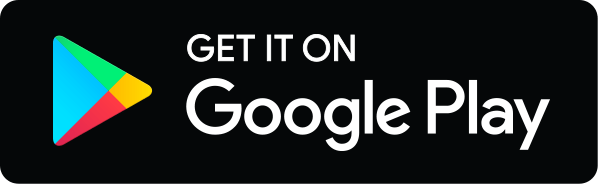
