Perfusion for Thoracic Aortic Surgery
Scott A. LeMaire
Joseph S. Coselli
Steven A. Raskin
Terry N. Crane
Eric Jenkins
Murphy Rayle
Victoria Vasileiadou
Kim I. de la Cruz
Surgical repair of thoracic aortic aneurysm and dissection has been an active field of innovation and investigation for more than 50 years. Although outcomes have greatly improved over the past few decades, operative mortality and neurologic complications remain the greatest challenges associated with open aortic repair. The diversity of surgical approaches across institutions is a testament to the complexity of these procedures and the lack of evidence regarding the efficacy of many technical details (1).
Because of the risks related to open repair, hybrid and purely endovascular approaches to treating disease of the thoracic aorta have recently been developed as less invasive alternatives for high-risk patients (1,2). The main pitfalls of procedures involving endovascular techniques include the risks of embolic stroke and repair failure due to stent migration or endoleak. Although the feasibility of hybrid and endovascular techniques has been demonstrated, long-term durability and survival data are yet to be collected, and once they are, they must be evaluated against data from contemporary series of open surgical repairs (1,3). Extending hybrid and purely endovascular techniques to low-risk surgical candidates with extensive thoracic aortic disease would be premature at this point, and although the indications for these approaches will no doubt continue to expand, there will remain a need for open repair and the associated perfusion techniques for many years to come.
Thoracic aortic aneurysms require individualized treatment. The specific challenges of each case must be considered, and decisions should be based on the aneurysm’s extent, rate of progression, and cause, as well as the patient’s overall health. Careful attention to imaging studies and preoperative planning are imperative for devising optimal surgical strategies. In these complex operations, protecting critical organs—including the brain, heart, spinal cord, kidneys, and other viscera—must be a primary goal, because periods of ischemia often determine the degree of injury and the eventual outcome.
Since the last edition of this book was published, there have been several important changes in the methods, equipment, and techniques used in thoracic aortic aneurysm surgery. The goal of perfusion in aneurysm operations is to enable the required repairs while minimizing ischemic injury, especially to the central nervous system. Many different strategies are needed, in part because each approach must be suited to the section of the aorta that is being treated.
The aorta can be thought of as having three major segments:
The ascending aorta. The ascending aorta begins at the aortic valve annulus and ends just proximal to the origin of the innominate artery.
The transverse aortic arch. The transverse aortic arch is the segment from which the three brachiocephalic branches arise, extending from the origin of the innominate artery to the origin of the left subclavian artery (LSCA).
The descending thoracic/thoracoabdominal aorta. The descending thoracic/thoracoabdominal aorta extends from just beyond the LSCA to the aortoiliac bifurcation.
Thoracic aortic aneurysms and dissections do not respect distinct boundaries; so the surgical and perfusion techniques used in their treatment must be adaptable for use in different segments. Because the perfusion techniques used in operations on the ascending and transverse sections often overlap, they are discussed together in the following section covering proximal aortic operations. Perfusion techniques used during repair of descending thoracic aortic aneurysm (DTAA) and thoracoabdominal aortic aneurysm (TAAA) are described in the subsequent section regarding distal aortic operations.
PROXIMAL AORTIC OPERATIONS
Proximal aortic operations are approached most commonly by median sternotomy. For nonemergent operations limited to the ascending aortic segment, we use cardiopulmonary bypass (CPB), whereas in cases of aneurysm rupture or acute dissection, we use hypothermic circulatory arrest (HCA) with selective antegrade cerebral perfusion (ACP). Although acute proximal aortic dissection can be repaired without HCA, using this technique eliminates the need for aortic clamping. The “open” distal anastomosis technique—originally advocated by Cooley and Livesay in 1981 (4)—avoids clamp injury of the fragile dissecting membrane, allows identification of tears within the transverse arch, and facilitates assessment of
aortic arch diameter. We also use HCA with ACP during graft replacement of the transverse aortic arch (5).
aortic arch diameter. We also use HCA with ACP during graft replacement of the transverse aortic arch (5).
Options for Cannulation
Disease that is limited to the aortic root or ascending aorta is approached with CPB. The arterial cannula can be often placed in the ascending aorta or transverse aortic arch, with the aortic cross-clamp applied proximal to the innominate artery. For patients in whom aortic cannulation is not feasible—such as those with disease extending into the aortic arch—one alternative for cannulation is the femoral artery, a particularly useful cannulation site in emergency situations with hemodynamically unstable patients. However, delivering CPB inflow from the femoral artery has several disadvantages in patients with extensive aortic disease (6). Most notably, retrograde flow through the diseased atheromatous distal aorta can potentially cause stroke and other complications by plaque embolization (7). Moreover, in cases of aortic dissection, blood flow may preferentially pressurize the false lumen, causing cerebral malperfusion.
The problems related to femoral artery cannulation can be avoided by using the right axillary artery for CPB inflow (Fig. 27.1) (8). The axillary artery is rarely involved in aneurysmal disease or dissection, which makes it a better site for establishing inflow. Furthermore, the right axillary artery provides a direct route to the right common carotid artery for ACP (as described in the section regarding adjuncts for cerebral protection).
Several groups, including ours, have reported that innominate cannulation is a safe and effective alternative for arterial inflow in proximal aortic surgery (Fig. 27.2) (9). We continue to use right axillary cannulation in selected redo operations when reentry is difficult or the innominate artery appears poorly suited for cannulation. We generally reserve femoral artery cannulation for patients who present in extremis and are hemodynamically unstable. When the right axillary and innominate arteries are unusable, alternative proximal options include the left axillary and carotid arteries.
When an arterial cannula is used, the size of the cannula is chosen to minimize the pressure gradient across the cannula at the patient’s calculated flow rate, which is based on a standard cardiac index of 2.2 to 2.4 L/min2 for adults at normothermic temperatures. The cannula should be large enough to provide the calculated flow rate with a gradient of less than 100 mm Hg. After the cannula is inserted into the vessel, it is connected to the circuit tubing during a forward pump roll to facilitate air removal at the connection. If a femoral arterial cannula is used, it is inserted percutaneously over a guidewire, de-aired, and attached to the arterial side of the circuit as fluid is slowly run forward in order to create a wet connection
during a forward pump roll. After the cannula is placed, reverse flow is briefly allowed to ensure that a false lumen has not been cannulated accidentally. Poor arterial return into the cannula during this maneuver may indicate proximal arterial obstruction, which necessitates repositioning the cannula or changing to an alternative cannulation site. A forward roll is then commenced to confirm good access (5).
during a forward pump roll. After the cannula is placed, reverse flow is briefly allowed to ensure that a false lumen has not been cannulated accidentally. Poor arterial return into the cannula during this maneuver may indicate proximal arterial obstruction, which necessitates repositioning the cannula or changing to an alternative cannulation site. A forward roll is then commenced to confirm good access (5).
![]() FIGURE 27.2. Innominate artery perfusion is delivered through a graft sutured to the anterior aspect of the artery. (Used with permission of Baylor College of Medicine.) |
Venous cannulation is accomplished with a right atrial dual-stage cannula, bicaval cannulas, or a long femoral venous cannula that extends up the inferior vena cava and into the base of the right atrium. This long cannula sometimes requires vacuum or kinetic assistance for drainage. Like femoral arterial cannulation, femoral venous cannulation is routinely used in hemodynamically unstable patients who require pump support before sternotomy. It is particularly useful in patients who are at risk of aortic injury during sternotomy, including patients undergoing reoperation and those with large ascending aortic aneurysms abutting the sternum. In such patients, the femoral cannula is placed before sternotomy is performed.
A left ventricular sump cannula is inserted through a purse-string suture placed at the junction of the right superior pulmonary vein and the left atrium. The sump minimizes preload, prevents ventricular distention, reduces myocardial rewarming, prevents ejection of air, and facilitates exposure of the aortic valve. An in-line pressure-relief valve is located between the sump cannula and a roller pump, which returns the vented blood to the bypass circuit either through the cardiotomy reservoir or directly into the venous reservoir. The degree of sump suction is constantly regulated, because too little suction allows ventricular distension, and too much can cause ventricular collapse and injury.
Myocardial protection is achieved by using systemic hypothermia and a combination of antegrade and retrograde cardioplegia, which facilitates adequate delivery of cardioplegia to all areas of the heart. We use a one-pass circuit to deliver 4°C blood-crystalloid (4:1) cardioplegia solution. The retrograde cardioplegia cannula is inserted into the coronary sinus after venous cannulation so that the coronary sinus cannula will not be dislodged when the venous cannula is positioned in the inferior vena cava. The initial bolus of antegrade cardioplegia is delivered either into the aortic root through an aortic needle placed proximal to the aortic cross-clamp, or directly into the coronary ostia through a cannula held by forceps after the aorta is opened. Then, additional boluses of cardioplegia solution are delivered directly into each coronary ostium at a rate of 250 mL/min approximately every 6 minutes in the amount specified at the time by the surgeon.
Hypothermic Circulatory Arrest and Cerebral Perfusion Adjunct
Improvements in aortic arch surgery outcomes have been partly attributed to better cerebral protection techniques. When DeBakey et al (10) first successfully replaced the aortic arch in the 1950s, they directly perfused the brachiocephalic branches during the operation. Since that first arch-replacement procedure, and since the first application of hypothermia to aortic surgery by Griepp and colleagues in 1975 (11) by inducing deep HCA at a body temperature of 18°C, the two main methods of brain protection have been inducing different degrees of hypothermia and using adjuncts for cerebral perfusion (12).
Soon after Griepp’s report was published, Crawford and Saleh (13) adopted the HCA technique and reported its efficacy in reducing morbidity and mortality. The protective limits of HCA were defined in 1993 by Svensson et al. (14), who reported Crawford’s experience with 656 patients who underwent HCA during proximal aortic surgery. The overall rates of transient stroke, permanent stroke, and early mortality were low, but the incidence of perioperative neurologic complications rose sharply when the HCA time exceeded 40 minutes,
and mortality increased dramatically when HCA time exceeded 65 minutes. The limitations of using HCA alone (i.e., without perfusion adjuncts) are now well recognized. During arch repairs that only necessitate short periods of HCA (i.e., less than 30 minutes, such as in elective hemiarch replacement), HCA alone provides satisfactory brain protection. However, during more complex repairs (e.g., total arch replacement) that necessitate substantially longer HCA times, the use of HCA alone is associated with a high risk of stroke and death (14).
and mortality increased dramatically when HCA time exceeded 65 minutes. The limitations of using HCA alone (i.e., without perfusion adjuncts) are now well recognized. During arch repairs that only necessitate short periods of HCA (i.e., less than 30 minutes, such as in elective hemiarch replacement), HCA alone provides satisfactory brain protection. However, during more complex repairs (e.g., total arch replacement) that necessitate substantially longer HCA times, the use of HCA alone is associated with a high risk of stroke and death (14).
In the late 1980s, we adopted the use of retrograde cerebral perfusion (RCP) as an adjunct to HCA (Fig. 27.3) (15,16,17). Electroencephalographic (EEG) monitoring was used to guide the induction of deep or profound hypothermia (<20°C); cooling was stopped when EEG silence was achieved. To enhance brain protection, dexamethasone was given 3 minutes before HCA was initiated. Once HCA was started, RCP was used to direct oxygenated blood from the CPB circuit into the head through the snared superior vena caval cannula. The RCP flow was delivered through a connection between the arterial inflow line and the superior vena caval cannula line (16).
Although RCP became a widely used adjunct in the 1990s, the expanding experimental and clinical data did not consistently support the efficacy of RCP for cerebral protection (18,19,20,21,22,23). There were questions as to whether RCP produces sufficient flow to meet the metabolic demands of the brain or even whether RCP provides any flow through the cerebral
microvasculature. Experimental data increasingly suggested that RCP does not effectively deliver blood to the brain; rather, the benefits of RCP seemed to be chiefly related to maintaining regional hypothermia and to flushing air and debris out of the cerebral circulation.
microvasculature. Experimental data increasingly suggested that RCP does not effectively deliver blood to the brain; rather, the benefits of RCP seemed to be chiefly related to maintaining regional hypothermia and to flushing air and debris out of the cerebral circulation.
We used RCP during HCA for many years; however, as evidence mounted for the benefits of ACP, in the last decade, we have shifted to using HCA and selective ACP almost exclusively when repairing acute ascending aortic dissection or aortic arch aneurysm (5). Our initial approach to ACP involved placing balloon-tipped catheters in the origins of the innominate artery and the left common carotid artery (LCCA) to deliver ACP during the HCA period (Fig. 27.4). Subsequently, the shift from using RCP to using ACP was further facilitated by a second major change in technique: the introduction of right axillary artery perfusion (Figs. 27.1 and 27.5A) (8). Once systemic HCA has begun, ACP is initiated by reducing axillary inflow to 10 mL/kg/min and occluding the innominate artery by using either a Rumel tourniquet or a vascular clamp. Because most patients have a complete circle of Willis, right-sided ACP generally provides adequate left-sided cerebral protection. When direct left-sided ACP is indicated, it can be delivered through the LCCA by inserting a balloon-tipped catheter that has been connected to a Y-limb from the arterial CPB line (Fig. 27.5) (16).
Multiple studies (16,24,25) have shown that ACP extends the safe duration of arch intervention beyond what HCA alone provides, especially when the distal arch repair time exceeds
30 minutes. However, the ideal core temperature for HCA with ACP has yet to be determined (1). We found that temperature below 20°C is associated with a 9-fold increase in hospital death, as well as significant increases in the 30-day mortality rate and CPB time and a nonsignificant increase in the incidence of stroke (12). Our current routine approach to open repair of the aortic arch features either right axillary artery or innominate artery CPB inflow and moderate HCA (23°C-25°C) with ACP. We use this approach for all openarch replacement procedures, including hemiarch replacements, total arch replacements, elephant trunk procedures, aortic dissection repairs, as well as repairs of aortic aneurysm without dissection.
30 minutes. However, the ideal core temperature for HCA with ACP has yet to be determined (1). We found that temperature below 20°C is associated with a 9-fold increase in hospital death, as well as significant increases in the 30-day mortality rate and CPB time and a nonsignificant increase in the incidence of stroke (12). Our current routine approach to open repair of the aortic arch features either right axillary artery or innominate artery CPB inflow and moderate HCA (23°C-25°C) with ACP. We use this approach for all openarch replacement procedures, including hemiarch replacements, total arch replacements, elephant trunk procedures, aortic dissection repairs, as well as repairs of aortic aneurysm without dissection.
The Trifurcated Graft Technique for Arch Replacement
When performing total arch replacement, we currently use the trifurcated graft technique (Fig. 27.6), which we incorporated into our practice in 2006 (26). This technique employs a single- or double-Y-graft that can be constructed to suit the patient’s anatomy and the planned reconstruction. Alternatively, an appropriately sized, prefabricated Y-graft can be used. In either case, the trifurcated graft is used to enable continuous delivery of ACP during the HCA period and to attach the brachiocephalic branches to the main aortic graft. This technique enables the use of bilateral ACP for cerebral protection, permits a shorter duration of cerebral HCA, and isolates the brachiocephalic vessels from the often-diseased atheromatous aorta near their origins. It provides continuous brain protection and allows great versatility of the Y-graft to accommodate arch anomalies or to modify the sequence of anastomoses according to intraoperative findings. Further, the trifurcated graft can be precisely tailored to the patient’s anatomy, so the potential for kinking is lower than it is with the use of prefabricated four-branched arch grafts, in which the position of the branches is fixed.
Technical Details of Aortic Arch Repair Procedures
Before general anesthesia is induced, we begin bilateral cerebral near-infrared spectroscopy with an INVOS Cerebral Somatic Oximeter (Covidien, Mansfield, MA). Noninvasive sensors are placed on the forehead, over the frontal lobes, to monitor brain oxygenation throughout the procedure. General anesthesia is then induced with etomidate, midazolam, fentanyl, and isoflurane. Before incision, patients receive either aminocaproic acid or tranexamic acid as an antifibrinolytic (27). Whenever possible, we collect one to three autologous units of blood before heparinization to achieve a hematocrit of 23% to 25%; this is mainly done in patients whose baseline hematocrit is less than 20%. The autologous units are then administered after protamine administration and surgical hemostasis. To enhance cerebral protection during HCA, we add 25 g of mannitol to the pump prime and administer an intravenous dose of dexamethasone (100 mg) before initiating CPB, although the supporting evidence for this practice is equivocal.
Performing HCA safely requires the careful monitoring of several temperatures throughout the procedure, including the patient’s nasopharyngeal temperature. Also monitored in the perfusion circuit are the temperatures of the arterial blood at the oxygenator outlet, the venous blood about to enter the venous reservoir, and the water for the heat exchanger unit. Other possible temperatures to monitor are those of the patient’s bladder, arterial perfusate, venous perfusate, and cardioplegia solution.
Our arterial cannulation strategy has shifted from femoral and direct aortic cannulation to right axillary and, more recently, innominate artery cannulation (8,9). Unlike femoral cannulation, both right axillary and innominate artery cannulation prevent malperfusion and retrograde cerebral atheroembolism, and facilitate delivery of ACP throughout the HCA period (Fig. 27.5). The axillary artery is approached through a 4- to 6-cm incision made at the right deltopectoral groove (Fig. 27.1). After the fibers of the pectoralis major muscle are separated, the underlying pectoralis minor is divided to expose the fat pad immediately posterior to the muscle. The brachial plexus lies beneath the fat pad, so care must be taken to avoid causing direct trauma or electrical injury to it during cautery. The axillary vein is usually retracted inferiorly to expose the artery. A short segment of the artery is then mobilized and encircled with a vessel loop. After the artery is exposed, the sternum is opened and, once sternal and mediastinal hemostasis has been achieved, a 3-mg (300 International Units/kg) bolus of heparin is administered. A partial occluding clamp is then applied to achieve proximal and distal control. An 8-mm polyester graft is anastomosed to the axillary artery in end-to-side manner with running 6-0 polypropylene suture. After the graft is carefully flushed and de-aired, it is secured to the inflow, or arterial, arm of the CPB circuit with a 3/8- to ¼-inch reducer connector, a plastic band, and heavy silk ligature. Alternatively, the axillary artery can be cannulated directly. During cooling, the innominate artery is exposed, posterior and superior to the innominate vein, and encircled with an umbilical tape passed through a tourniquet.
Innominate artery cannulation has several advantages over axillary artery cannulation. First, there is no need for an additional incision; thus, the operative time is potentially shorter. Second, because the cannulation site remains under the surgeon’s direct vision at all times, additional blood loss and possible kinking of the inflow graft can be avoided. Third, the innominate artery is technically easier to cannulate in obese patients than the axillary artery. Fourth, the risk of brachial plexus injury and arm ischemia or claudication is eliminated.
The innominate artery is exposed to its bifurcation and encircled with an umbilical tape (Fig. 27.2). Three minutes after 1 mg/kg of heparin is given, a partial occluding clamp
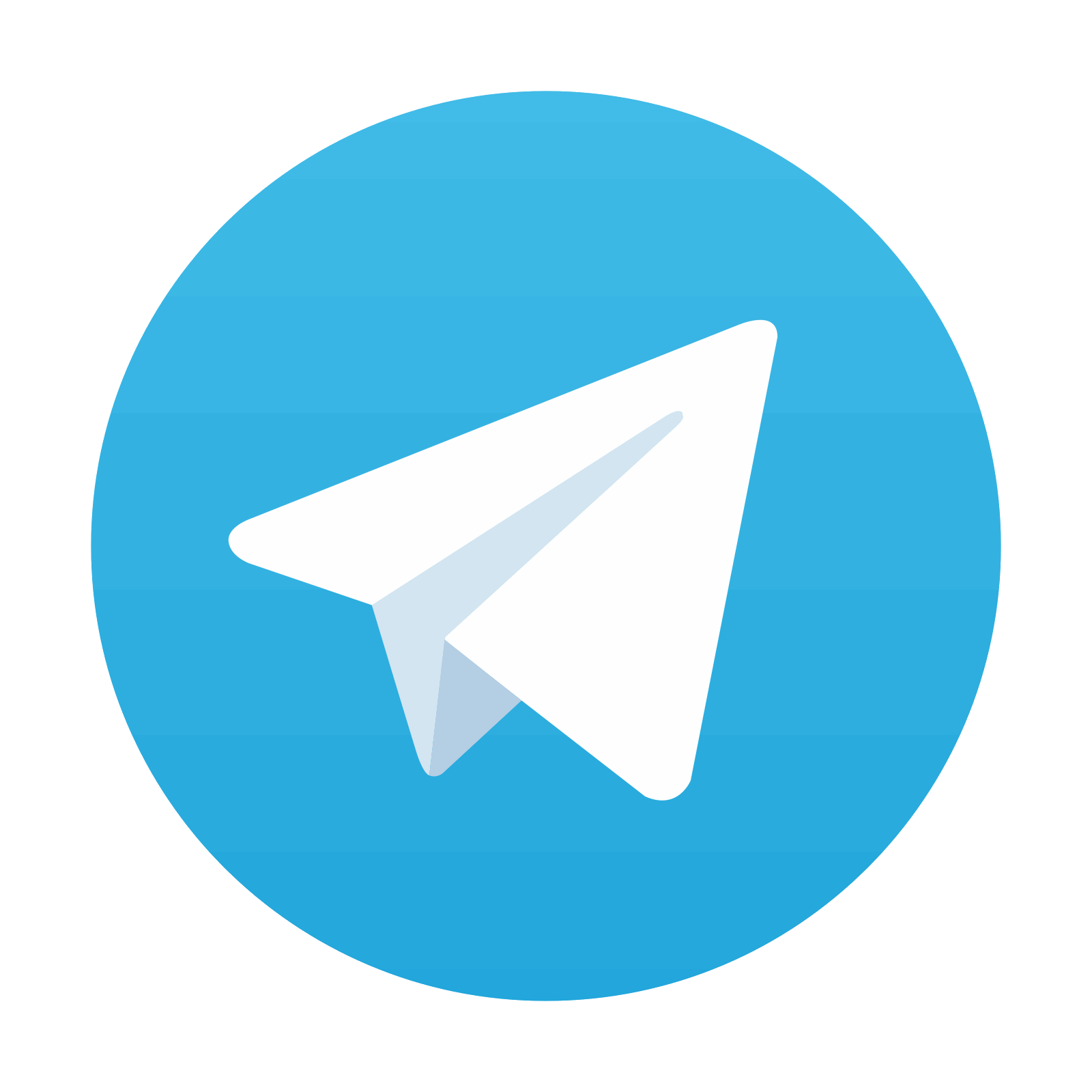
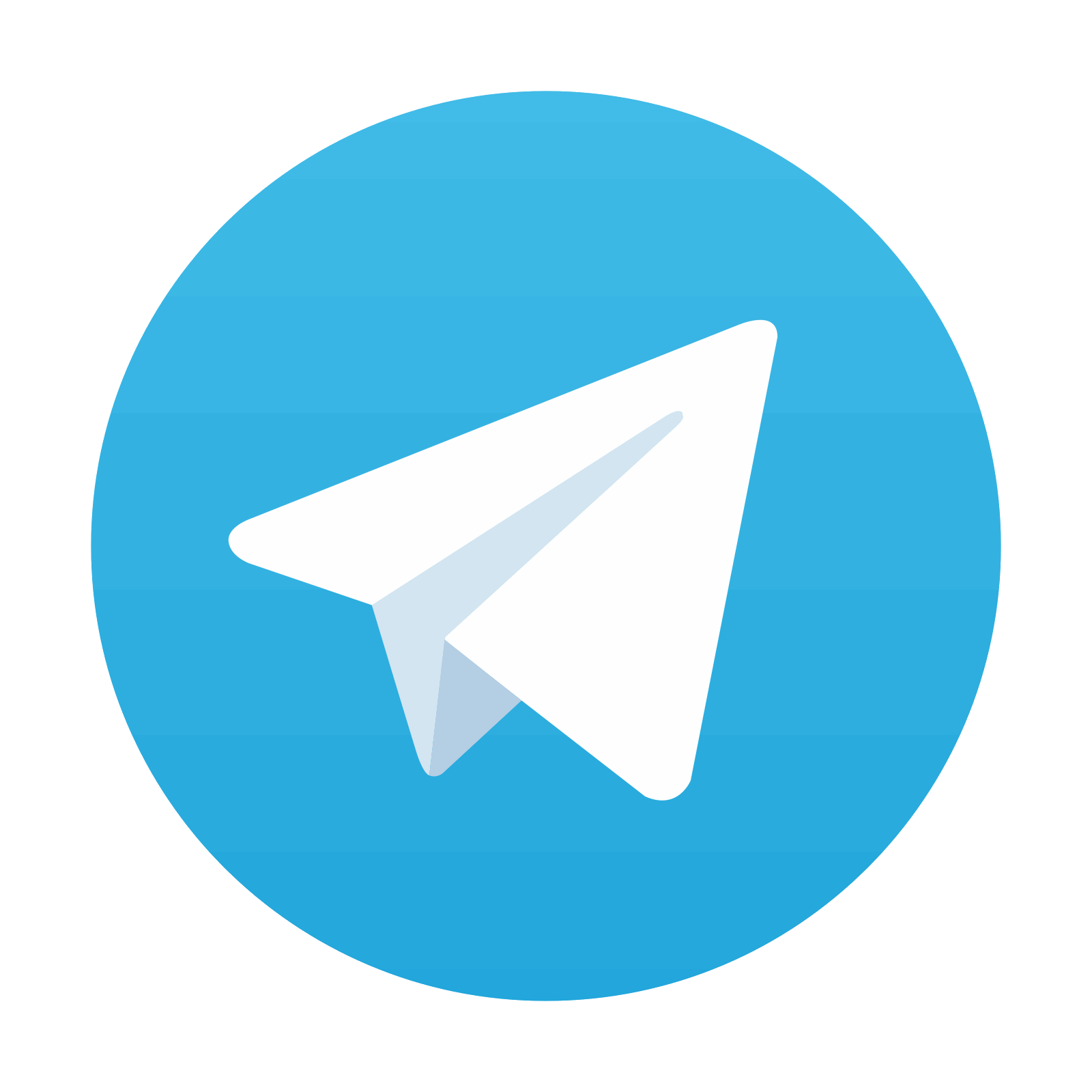
Stay updated, free articles. Join our Telegram channel

Full access? Get Clinical Tree
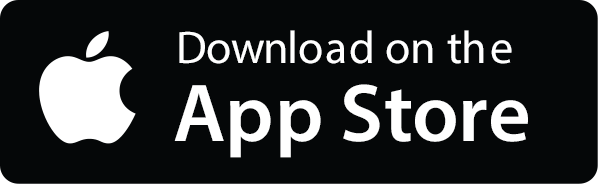
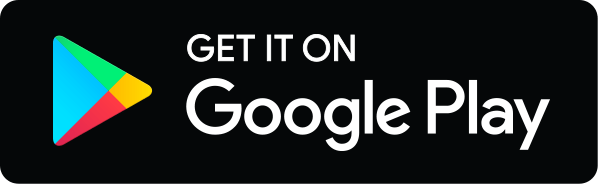
