Background
Contrast echocardiography (CE) provides closer agreement with magnetic resonance imaging (MRI) for left ventricular (LV) volumes and ejection fraction (EF) than noncontrast echocardiography. However, the feasibility and role of myocardial deformation analysis on contrast echocardiographic images have not been well established. The aim of this study was to assess the feasibility of deformation analysis on CE using a new software tool that provides simultaneous measurements for LV volumes and EF.
Methods
Data from 52 patients who were recruited for the Alberta Heart Failure Etiology and Analysis Research Team Study (34 men; mean age, 64 ± 9 years) and underwent CE and MRI were considered. Contrast bolus injections were administered for optimal endocardial definition. Offline LV volume analysis was performed by standard manual tracing. A single frame was traced manually for two-dimensional (2D) cardiac performance analysis (CPA), which automatically calculated LV volumes, EF, and global longitudinal strain (GLS). Volumes obtained with 2D CPA were compared with those measured with standard CE and MRI. GLS from noncontrast echocardiographic recordings was also calculated with 2D CPA and compared with CE-derived and MRI-derived GLS.
Results
Tracing of contrast echocardiographic images with 2D CPA was possible in 49 out of 52 patients, and measurements correlated well with standard CE and MRI (EF: r = 0.93, P < .001, and r = 0.85, P < .001, respectively). Mean GLS from noncontrast echocardiographic and contrast echocardiographic recordings was −13.4 ± 5.8 and −15.3 ± 4.64, respectively ( P = .056), and the latter correlated well with MRI-derived GLS ( r = 0.78 vs 0.81, respectively).
Conclusions
Simultaneous volumetric and deformation analysis on contrast echocardiographic recordings is feasible and reproducible. While volumes and EF obtained with the new software compare well with those obtained from standard CE and MRI, GLS from CE shows a good correlation with strain measured with MRI.
Left ventricular (LV) systolic function as assessed by volume and ejection fraction (EF) parameters is a widely validated and strong predictor of outcomes in cardiac patients. On the other hand, speckle-tracking imaging is emerging as a complementary technique for the assessment of LV function and has been shown to be particularly relevant both in patients with overt as well as subclinical ventricular dysfunction. However, factors such as poor acoustic view with impaired endocardial border identification may often limit proper assessment of LV function in terms of both volumetric and deformation analysis.
The advent of contrast echocardiography (CE) has significantly overcome the difficulties in the assessment of LV volumes and EF. Nonetheless, strain measurements of contrast recordings performed with Doppler tissue imaging were shown to be unreliable and as such have been discouraging.
Myocardial strain analysis of contrast images was shown to be feasible with speckle-tracking, but the variability between strain measurements of contrast and noncontrast recordings was considered a potential limitation to the technique.
In this study, we sought to test the feasibility of speckle-tracking analysis on contrast images by using a new software tool that provides simultaneous measurements for LV volumes, EF, and myocardial deformation parameters.
Methods
Data from 52 patients who were recruited for the Alberta Heart Failure Etiology and Analysis Research Team Study ( http://www.albertaheartresearch.ca ; mean age, 64 ± 9 years; 34 men) between September 2010 and April 2011 were considered for this pilot study. Study patients belong to one of four prespecified categories—(1) systolic heart failure (HF), (2) diastolic HF, (3) diastolic dysfunction, and (4) increased risk for diastolic HF (i.e., patients with known risk factors but no clinical symptoms of HF)—and, as part of the study protocol (unless contraindications are present), undergo both CE and cardiac magnetic resonance imaging (MRI). Exclusion criteria for enrollment in our study were atrial fibrillation or other arrhythmias and contraindication to contrast media administration.
Cardiac MRI Evaluation
All MRI examinations were performed using a 1.5-T Siemens Sonata scanner (Siemens Healthcare, Erlangen, Germany) and a five-element cardiac array for signal reception, as previously described. All image acquisitions were electrocardiographically gated and acquired during breath holds. End-diastolic volume, end-systolic volume (ESV), and EF measurements were derived from manual segmentation of short-axis cine images at end-systole and end-diastole (Argus; Siemens Healthcare), and EF was derived using Simpson’s rule. Additionally, the endocardial tracings in conjunction with apical and basal locations from two-chamber, three-chamber, and four-chamber long-axis views were used to generate an endocardial surface of the left ventricle at end-systole and end-diastole. Peak systolic global longitudinal strain (GLS) was calculated using the average of the change in length of the surface from end-diastole to end-systole divided by the lengths at end-diastole.
Echocardiographic Evaluation
After the acquisition of noncontrast echocardiographic images, 0.1 mL Definity (Lantheus Medical Imaging, North Billerica, MA) in slow bolus injections, followed by slow saline flush, was administered for optimal endocardial definition using an iE33 scanner (Philips Medical Systems, Andover, MA). We used standard settings with low to intermediate mechanical index (mean values ranging from 0.3 to 0.4) for contrast recordings, with images optimized to achieve dense and homogenous LV opacification and dark myocardium. The maximum frame rate to achieve LV opacification was used for this study (39 frames/sec). Although higher frames rates would be advantageous for speckle imaging, they cannot be realized with the current scanners and would probably impair LV opacification and endocardial definition. Offline LV volume and EF analysis for noncontrast and contrast images was performed using standard manual tracing of end-systolic and end-diastolic endocardial borders from apical two-chamber and four-chamber images (Xcelera; Philips Medical Systems), and results were obtained using Simpson’s biplane method. In addition, echocardiographic recordings were uploaded to the new software, the two-dimensional (2D) cardiac performance analysis (CPA) for speckle-tracking imaging (frame rate, 39 frames/sec; TomTec, Munich, Germany). Two-dimensional CPA has been developed for noncontrast echocardiography. This technique works very similar to Velocity Vector Imaging (Siemens Healthcare). Two-dimensional CPA tracks speckles mainly at the endocardium. This is different from speckle-tracking as implemented in many clinical scanners, and it also tracks speckles in the myocardium. The operator scrolled through the cardiac cycle and, irrespective of cardiac cycle, identified a single frame with good endocardial definition, which was usually during systole. A minimum of 10 border points, at the operator’s discretion, were depicted for manual tracking. Then tracing of the endocardial border throughout the cardiac cycle was automatically performed, and LV volumes and EF were computed. In addition, speckles were tracked in a frame-by-frame fashion, and traces depicting segmental longitudinal strain were computed automatically. After the automatic tracking throughout the cardiac cycle, recordings were reviewed to identify studies with inappropriate tracking. No corrections of the tracked contour were made. The tracking was regarded as unsuccessful if the tracked contour on end-diastolic or end-systolic frames was >5 mm away from the visually perceived border of the LV cavity in two or more of six segments in the four-chamber or two-chamber view.
Systolic GLS was obtained by averaging the segmental strain curves at maximum instantaneous peak. Similarly, noncontrast images from the same patients were also analyzed with the software and strain parameters were recorded.
LV volumes and EF derived from 2D CPA were compared with those obtained using MRI and the contrast images analyzed with Xcelera using Simpson’s biplane rule. Mean GLS from contrast echocardiographic recordings were compared with those obtained from noncontrast echocardiographic images. Two experienced physicians assessed the image quality for noncontrast recordings, with optimal quality defined as adequate visualization of all myocardial segments. In a subset analysis for GLS, only data from patients with optimal image quality on noncontrast echocardiographic recordings were included for comparison between contrast and noncontrast measurements. In another subset of 22 patients, in which MRI analysis had also been performed, GLS strain data from contrast and noncontrast echocardiographic recordings were correlated with those derived from MRI.
Reproducibility Analysis
All 2D CPA measurements were repeated in a subset of 20 patients by a second physician and by the same primary reader, who were blinded to data. Repeated measurements were performed ≥2 weeks after the first evaluation. As mentioned previously, the selection of the cardiac cycle for the manual border detection was left to the operator’s discretion. Interobserver and intraobserver variability was calculated as the absolute difference of the corresponding pair of repeated measurements and as a percentage of their means in each patient and then averaged over the total number of patients, and correlation was tested using intraclass correlation coefficients.
Data are presented as mean ± SD or percentages as appropriate. Student’s t tests were used for comparisons of continuous data. Linear regression analysis was used to correlate measurements among different techniques, with agreement assessed using Bland-Altman analysis and intraclass correlation coefficients, as described previously.
All measurements were analyzed using SPSS version 18 for Windows (SPSS, Inc, Chicago, IL). P values < .05 were considered statistically significant.
Results
Twenty-three patients included in our study had systolic HF, nine had diastolic HF, 11 had diastolic dysfunction, and nine were at increased risk for diastolic HF. Baseline characteristics of the patient population are presented in Table 1 . Of the patients undergoing echocardiography, 20 were qualified as having optimal image quality on noncontrast recordings. All patients received contrast agent for endocardial border delineation to obtain a quantitative EF. Forty-five patients underwent cardiac MRI, with seven patients not completing MRI evaluation because of either contraindications (three patients) or claustrophobia (four patients).
Variable | Patients | |||
---|---|---|---|---|
Systolic HF | Diastolic HF | Diastolic dysfunction | Risk for diastolic HF | |
( n = 23) | ( n = 9) | ( n = 11) | ( n = 9) | |
Men | 17 | 3 | 6 | 6 |
Age (y) | 59 ± 5 | 70 ± 5 | 60 ± 5 | 61 ± 5 |
EF (%) | 34 ± 4 | 52 ± 5 | 56 ± 4 | 60 ± 7 |
After contrast administration, image quality significantly improved in all patients. Manual tracing of contrast echocardiographic images using the 2D CPA software was possible in all 52 patients. Simultaneous processing for volumes and strain parameters with the new software was not successful in 3 patients, in whom visually detected deviation >0.5 cm of the computed tracking contour from the myocardial borders was found. Apical contrast destruction, contrast swirling, and myocardial opacification were found to impair the semiautomatic border detection in these patients ( Figure 1 ). None of these patients belonged to the group with good image quality, and they also could not be properly processed before contrast administration. Conversely, in 49 of 52 patients (94.2%), the tracking contour followed the myocardial movements with <0.5 cm deviation.

Mean volumes and EFs assessed using different techniques are presented in Table 2 . LV volumes assessed with MRI, standard CE, and 2D CPA were significantly higher than volumes measured with noncontrast echocardiography using Simpson’s biplane rule. Volumetric measurements with the new software correlated well for all parameters with standard CE and CMR: end-diastolic volume ( r = 0.96, P < .001, and r = 0.82, P < .001 respectively), ESV ( r = 0.94, P < .001, and r = 0.92, P < .001, respectively), and EF ( r = 0.93, P < .001, and r = 0.85, P < .001, respectively) ( Figure 2 ). Mean bias and 95% limits of agreement for EF measured with standard CE and MRI were −5.2 ± 5.6% and −5.2 ± 7.5%, respectively ( Figure 3 ).
Parameter | Technique | |||
---|---|---|---|---|
MRI | 2D CE | 2D CPA | Standard 2D echocardiography | |
EF (%) | 49 ± 15 | 48 ± 17 | 53 ± 15 | 47 ± 10 |
EDV (mL) | 186 ± 68 | 163 ± 59 | 176 ± 58 | 112 ± 44 |
ESV (mL) | 107 ± 72 | 92 ± 54 | 87 ± 54 | 64 ± 37 |


Mean GLS from noncontrast and contrast recordings of the whole patient population was −13.4 ± 5.8 and −15.3 ± 4.64, respectively, with a trend toward a statistically significant difference between the groups ( P = .056). Correlation ( r = 0.72, P < .01) and agreement (bias, −1.55; 95% limits of agreement, −9.4 and 6.35) for GLS between noncontrast and contrast recordings are shown in Figure 4 .

In the subset of patients with good image quality, average GLS did not significantly differ between noncontrast (−14.3 ± 4.3) and contrast (−14.5 ± 4.45) recordings ( P = .87), and there was a better correlation ( r = 0.89, P < .01) and closer agreement (mean bias, −0.39 ± 2.1; 95% limits of agreement, −4.5 and 3.76) between measurements ( Figure 5 ). On the other hand, there was a significant difference between average GLS for noncontrast (−13.2 ± 3) and contrast (−15.8 ± 4.5) recordings of patients with nonoptimal image quality at baseline ( P = .02), with looser agreement (mean bias, −1.99; 95% limits of agreement, −11.3 and 7.28) and correlation ( r = 0.6, P < .001).
In the subset of patients undergoing MRI strain analysis, GLS derived from contrast images correlated well with MRI-derived GLS ( r = 0.81, P < .01). A slightly worse correlation with MRI was found between GLS obtained from noncontrast recordings ( r = 0.78, P < .01; Figure 6 ). Finally, as presented in Table 3 , there was reasonable interobserver and intraobserver agreement for all 2D CPA measurements.
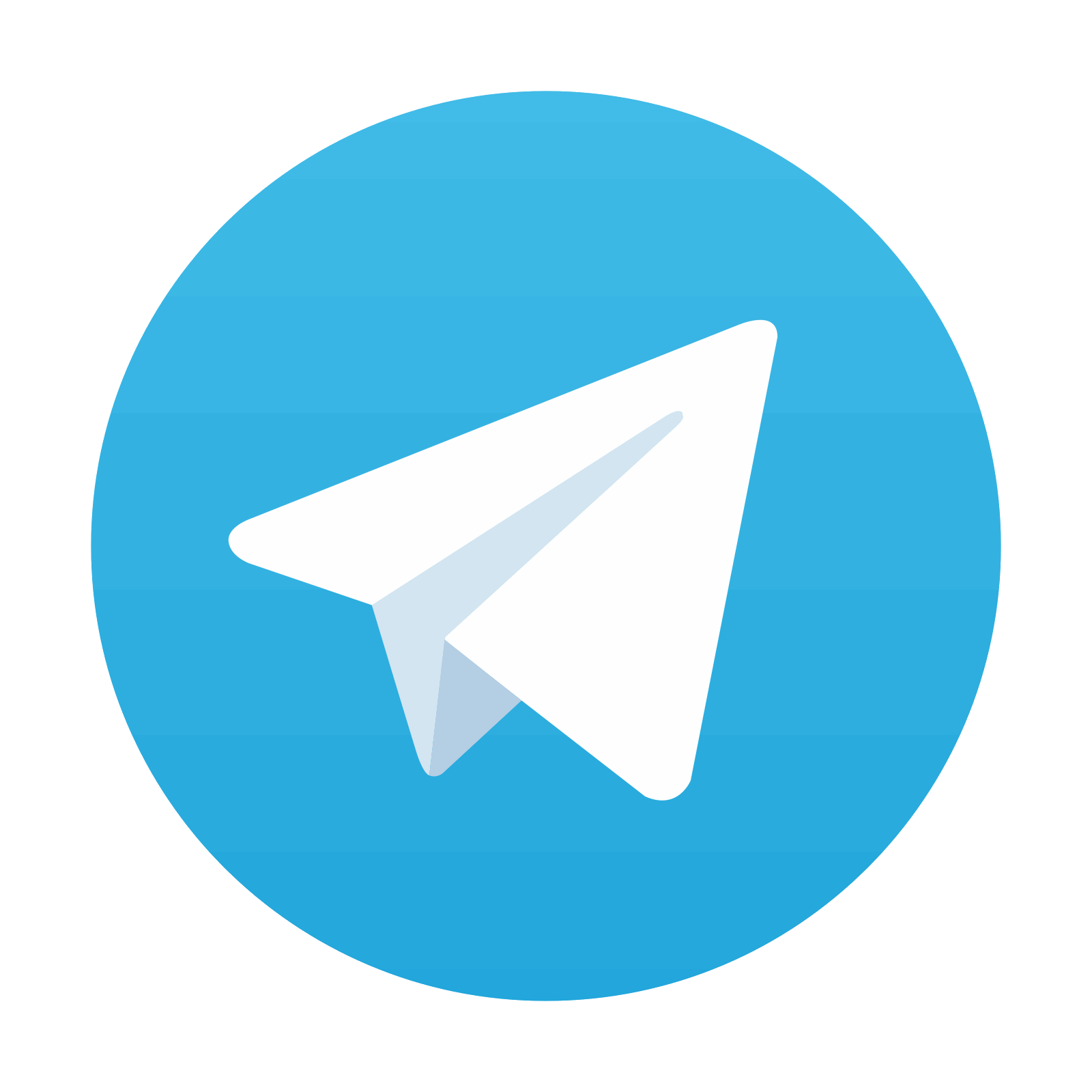
Stay updated, free articles. Join our Telegram channel

Full access? Get Clinical Tree
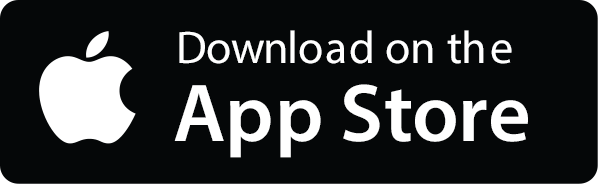
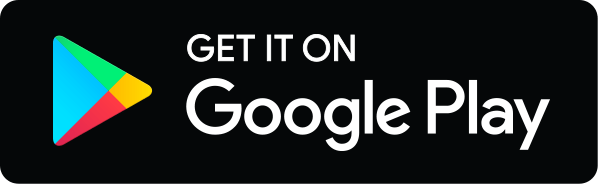
