The mature mammalian heart can be represented as a four-chamber blood-pumping organ consisting of contractile cardiomyocytes and supportive stromal cells surrounded by fibrous connective tissue. After a period of intense cardiomyocyte proliferation and heart growth in the embryo, cell expansion and turnover become undetectable in the postnatal phase. Traditionally, the mature heart was thought to contain only a discrete number of quiescent cells (1 to 2 × 10 10 cells/heart [average]), with little regenerative potential. The heart maintains an average heart rate of 70 beats/min throughout an individual’s life span. Noncardiomyocytes make up 75% of the total number of cells in the heart and only 25% of the total cell volume. The ratio of number of capillaries that provide oxygen and nutrients per cardiomyocyte is 1 : 1. In a setting of slow cell turnover and little regenerative potential, dysregulated or accelerated cell death under a pathophysiologic stress can rapidly lead to cell depletion, loss of function, and heart failure.
Although the overall cardiomyocyte population declines with age, proliferating cardiomyocytes have been recognized in normal adult hearts. , Their exact biologic significance remains uncertain. Evidence suggests that cardiomyocytes may not be quiescent terminally differentiated cells, but cells that respond to the modern cell biology concepts of proliferation, death, and ongoing turnover throughout adulthood.
Types of Cell Death
Necrosis and Apoptosis
Myocardial infarction causes cardiomyocyte death by ischemia that results from an imbalance between myocardial supply and demand of oxygen and nutrients. Necrosis, oncosis, and apoptosis are alternative mechanisms for demise. Strictly speaking, the term necrosis encompasses most terminal changes occurring in cell death, and oncosis is defined by cell swelling and karyolysis. This type of death is not governed by genetic regulation but is the consequence of biochemical insults and mechanical constraints that destroy essential cellular structures, mainly because of failure of membrane ionic pumps, resulting in cell swelling and rupture, inflammation, and tissue repair. Exudative inflammation follows as dying cells release toxic contents in tissues before being ingested by phagocytes. On the other hand, apoptosis is characterized by cell contraction and cell shrinkage and karyorrhexis. This type of death is genetically programmed and tightly regulated by a number of extra- and intracellular biochemical signaling pathways. Apoptotic cells are simply eliminated, without an inflammatory context and without compromising the survival of neighboring cells. There is strong interest in determining whether the prominent cardiac cell death seen after myocardial infarction (MI) occurs through regulated apoptotic reactions or indiscriminate necrosis. The former is believed to be an important mechanism; the latter is a key to the development of MI and its impact on subsequent cardiac remodeling and function.
Autophagy
The mechanisms for the degradation of mitochondria, other cytoplasmic organelles, and bulk proteins are under strict genetic control, referred to as autophagy or macroautophagy. Autophagy is characterized by the formation of double-membrane–lined vacuoles, known as autophagosomes, that merge with lysosomes to degrade their contents. Tagging with the ubiquitin peptide directs these cell constituents toward autophagosomes. The process is controlled by specific genes, such as the ATG gene family and beclin-1, which control the assembly and function of autophagosomes. Moderate and controlled autophagy ensures proper function and recycling of cell organelles, and promotes increased cardiomyocyte survival. Upregulation of autophagy by the overexpression of beclin-1 can be cardioprotective against ischemia/reperfusion (I/R) injury. On the other hand, the dysregulation of autophagy produces excessive ubiquitination of intracellular proteins or insufficient or inadequate lysosomal proteolytic activity. This leads to the absence of autophagosomes and accumulation of ubiquitinated proteins within the cell or to excessive destruction of essential organelles; both situations cause irreversible cell injury and death. Cardiomyocyte autophagy is activated in cardiac ischemia and further enhanced by reperfusion. Autophagosomes are found in apoptotic and necrotic cells of chronic ischemic hearts, and are seen as putative triggers for programmed cardiomyocyte death.
Cardiac Regeneration
Of the total cardiomyocyte population, 0.0014% are in a mitotic state in resting adult hearts, 0.015% in remodeling failing hearts, and up to 0.08% in the proliferative zones surrounding areas of recent infarcts. In a model of high cardiac cell turnover in transgenic mice expressing green fluorescent protein, up to 18.5% of cells adjacent to the infarct area and 7.5% of distal cells were mitotic in the first 3 months following coronary occlusion. Thus, I/R appears to be associated with some spontaneous cell renewal; the exact nature of these proliferating cells, however, is uncertain and they can be invasive inflammatory cells, cardiomyocytes, or stromal cells.
Hypoxic cardiomyocytes activate the hypoxia-inducible transcription factors (HIFs) that sense oxygen depletion and regulate emergency expression of antiapoptotic genes. Cardiac HIF-1α and HIF-2α strongly colocalize with markers of cardiomyocyte proliferation ; heterozygous transgenic mice partially deficient in HIF-1α, however, do not respond to ischemic preconditioning. Hence, cardiomyocyte HIF-1α is a key element for cell survival during ischemia, without necessarily protecting cardiac function completely.
It was demonstrated for the first time in 2003 that cardiomyocytes can originate from resident progenitor cell populations nested in specific zones of adult hearts, known as niches. These cells are now believed to possess significant proliferative capacity to mediate cardiomyocyte turnover. By contrast, bone marrow transplantation and other experimental studies have shown that relatively few progenitor cells have the potential to move from distal organs to repopulate injured or transplanted hearts. , Thus, cardiac proliferation may primarily consist of an expansion of resident cardiomyocyte progenitor cells . Whatever their sources and numbers, they are either insufficient or inappropriate in numbers to ensure spontaneous cardiomyocyte turnover and to compensate for the subsequent events of inflammation, apoptosis, tissue repair, and remodeling that result in an inert scar in place of contractile tissue.
Cardiac Death and Clinical Objectives
The current main drivers of treatment of MI are to open the artery and restore blood flow to prevent cardiac cell death, minimize infarct size, and maintain cardiac function. These measures are often ineffective or insufficient, fueling strong interest in novel cell therapies. Because the intrinsic proliferative capacity of mature adult cardiomyocytes does not allow sufficient or timely regeneration, alternative options are being tested, such as enhancing cardiomyocyte survival and/or replenishing contractile cells within the lesion. Cytoprotective approaches envisioned are further acceleration of reperfusion therapy, pharmacologic blockage of cell death, and artificial reintroduction of cells. Adding pharmacologic antiapoptotic therapy based on the knowledge of the timing, spatial distribution, and resolution of apoptotic reactions could help optimize therapeutic benefits.
Cardiomyocyte Death in the Ischemic and Reperfused Heart
Ischemia and Cardiac Death
Cardiac cell death occurs rapidly on MI in response to a number of stimuli, which include hypoxemia and energy depletion in the absence of aerobic reserve, reoxygenation, acidosis, oxidative stress, and cytokine stimulation. The schedule of events leading to death has been described in patients and in animal models. Diastolic and systolic dysfunction appear within 30 to 45 seconds of flow deprivation, followed by electrocardiographic changes; necrosis detected by sarcolemmal disruption appears within 30 to 40 minutes and cell death subsequently progresses exponentially, in a process initially described by Reimer and Jennings as “the wavefront phenomenon of myocardial death.” In the absence of reperfusion or significant collateral flow, necrosis involves almost all the area at risk after 6 hours of occlusion and severe ischemia ( Fig. 9-1 ).

Cardiomyocyte Death: Oncosis and Apoptosis
Oncosis is a form of cell death defined initially on histopathological bases and relevant to cardiomyocytes. It can be activated in three different ways: (1) damage to cell membranes, resulting in a loss of selective permeability, which contributes to the membrane attack complex (MAC) of the complement pathway; (2) membrane phospholipids degradation via dysregulated phospholipase activity or peroxidation by radical oxygen species (ROS); and (3) disruption of the respiratory chain machinery in mitochondria and a decrease in oxidative phosphorylation and the generation of adenosine triphosphate (ATP). , These events trigger sharp rises in intracellular Ca 2+ concentration, followed by uncontrolled influx of extracellular water and ions, gradual cell swelling, and rupture.
Apoptosis is identified by a number of unique modifications, including chromatin condensation and nuclear shrinkage, DNA fragmentation by endogenous nucleases, minor modifications of cytoplasmic organelles, plasma membrane blebs, and apoptotic bodies. In a normal state, apoptotic cells do not exceed 0.002% of the total cardiomyocytes population; following MI, it is as high as 0.08% to 0.25% in the infarct area for weeks. With the terminal deoxynucleotidyl transferase dUTP nick-end labeling (TUNEL) method, and internucleosomal fragmentation by agarose gel electrophoresis (200 base pair fragments), apoptosis is mainly detected in the border zone of histologically identified infarcts. Others have claimed that apoptosis is the major form of cardiomyocyte death, peaking as early as 4 to 5 hours after ischemia, with oncosis representing only 1 of 30 dead cells at this stage. However, some necrotic cardiomyocytes exhibiting DNA fragmentation do not show additional features of apoptosis, suggesting that true identification of apoptosis in MI could require not one but a combination of characteristics. Some cardiomyocytes associated with DNA nick-end labeling may actually reflect a state of DNA repair, , or apoptosis in stromal cells. The relative importance of apoptosis versus oncosis in MI remains unknown.
Border Zone Apoptosis
Inflammation triggers intense myofibroblast proliferation and angiogenesis in the infarct area, which results in a vascularized granulation tissue relatively rich in cells within 4 to 6 days after the infarction. During this phase of tissue repair, cardiac cells still undergo apoptosis at the edge of the histologically defined infarct area, as seen by caspase-3 activity, TUNEL labeling, and DNA laddering. This infarct border zone can account for up to 40% of the area at risk. High concentrations of thrombospondin-1 (TSP-1), a potent antiangiogenic agent and trigger of endothelial apoptosis, are seen within this zone, , suggesting that TSP-1 and apoptosis may serve as a barrier to the expansion of granulation tissue. In addition, TSP-1 facilitates the expression and activation of transforming growth factor beta 1 (TGF-β1), which exerts anti-inflammatory and antimitotic effects on multiple cells to contain the fibroproliferative reactions. Border zone apoptosis can thus be seen as cardioprotective.
Impact of Reperfusion
Whereas numerous cardioprotective methods have been described (see Chapter 25 for details), rapid restoration of blood flow is the ultimate requirement to confine ischemic cardiac death. Aggressive recanalization of coronary arteries is believed to represent the best approach for protecting cardiomyocytes. Fibrinolytic therapy and, whenever possible, primary angioplasty, are applied as promptly as possible. The window of opportunity for cardiomyocyte salvage lies within the first 3 hours after the coronary occlusion and may extend up to 12 hours. Despite its overall cardioprotective effects resulting in a reduction of mortality, rapid interventional recanalization of coronary vessels induces counterreactions. Reperfusion can accelerate exudative inflammation and death of surviving cardiomyocytes. , TUNEL-labeled apoptotic cardiac cells accounted for approximately 4% of dead cardiomyocytes and necrosis for 40%, in patients 30 minutes after I/R injury, and 6% and 12% after 2 to 4 hours of ischemia reperfusion, respectively.
Membrane phosphatidylserine (PS) externalization is considered one of the earliest markers of apoptosis. It is caused by a loss of membrane phospholipid symmetry, with PS accumulating on the outer surface of the membrane. Recombinant annexin V has a strong specific affinity for PS and is used as a marker of PS exposure. TUNEL-positive nuclei cardiomyocytes with intact plasma membranes are also seen in the I/R area complicating the interpretation of quantitative data. Late recanalization in the subacute stages between 4 days and 2 weeks after MI is associated with less cardiac apoptosis and remodeling than recanalization during the acute phase, providing an experimental basis for delayed revascularization.
Cardiac Death and Acute-Phase Inflammation
Inflammatory Cell Invasion
Myocardial necrosis is associated with exudative inflammation and tissue invasion by neutrophils and monocytes-macrophages. Ischemia induces the release of interleukin-1 (IL-1), interferon-γ (IFN-γ), IL-8, and tumor necrosis factor-α (TNF-α); the latter two are potent chemoattractants for neutrophils. Chemokines are a family of highly basic small proteins with a conserved tridimensional structure. IL-8 and lipopolysaccharide-induced CXC chemokine (LIX) are potent chemoattractive chemokines of the cysteine-X–cysteine ligand (CXCL) subgroup, and appear to mediate neutrophil invasion. Other chemokines of the cysteine-cysteine ligand (CCL) subgroup, such as macrophage chemoattractant protein-1 (MCP-1), MCP-3, and macrophage inflammatory protein-1α and -1β (MIP-1α and MIP-1β) are upregulated without influencing infarct size and angiogenesis but with delayed inflammatory cell recruitment and tissue repair, resulting in less remodeling. Genetic deletion of the main MCP-1 receptor, CCR2, causes a partially similar phenotype of delayed inflammatory cell invasion and reduced remodeling. Both CC and CXC play a major role in the rapid recruitment of leukocytes after MI. Activation of the complement cascade was also observed during acute cardiac injury. , The complement cascade is activated through the classic, alternative, and lectin pathways, and dying cardiomyocytes release complement-activating moieties. The potent anaphylatoxin C5 is specifically activated to recruit neutrophils within the first hour of ischemia and activates terminal complement pathways. Reperfusion triggers further increases in IL-8 and TNF-α complement activation and excessive inflammatory reactions. In the rabbit model, specific inhibition of activated protein C1 or the anaphylatoxins C3a and C5a by the soluble complement receptor type 1 results in a strong reduction in exudative inflammation. The complement MAC triggers oncosis in multiple cell types, including cardiomyocytes and endothelial cells, leading to local vascular injury. Despite all these cues, clinical trials with a C5-neutralizing antibody in patients with acute MI has failed to demonstrate improvements in infarct size or cardiac function.
Inflammation and Cell Death
Acute exudative inflammation generates a strong burst of ROS generation, increases nitric oxide (NO) release and the secretion of pro- and anti-inflammatory cytokines that profoundly modify the ischemic environment. These moieties also influence cardiomyocyte function and survival.
Reactive Oxygen Species
Superoxide secreted by neutrophils and from other sources and other ROS are highly reactive biochemical moieties that react and modify lipids, proteins, and DNA to cause cell damage and death. At rest, the toxic reactive species are neutralized by enzymatic reactions involving superoxide dismutases (SODs), catalase, or glutathione peroxidase. These enzymes, however, are overwhelmed by the ROS burst generated in MI. ROS activate the Na + /H + exchanger isoform 1 (NHE1), which mediates cytochrome C release into the cytosol, activation of caspase-3, and DNA degradation. NHE1 is phosphorylated and activated by p90 ribosomal S6 kinase (RSK). Transgenic mice with cardiac-specific overexpression of dominant negative p90 RSK displayed a 40% reduction in apoptosis on I/R injury. I/R and H 2 O 2 also decreased the expression of antiapoptotic proteins and sensitized cardiomyocytes to Fas-mediated apoptosis. Transgenic mice overexpressing superoxide dismutase (SOD) 1 or manganese SOD have smaller infarct sizes than littermates. The combined administration of recombinant SOD and catalase reduces infarct size in I/R injury in dogs, when administered immediately prior to ischemia or within minutes after reperfusion, situations that are rarely encountered in clinical practice. Inhibition of the Na + /H + exchanger with cariporide failed to benefit patients experiencing acute MI or undergoing coronary artery bypass grafting (CABG).
Toll-like receptors (TLRs) are a family of 12 cell surface effectors in innate immunity that mediate the recognition of molecular patterns associated with pathology. TLRs are activated by ROS, extracellular matrix fragments, microbacterial components, including lipopolysaccharides, and many other moieties from damaged tissues. TLR-4 is strongly upregulated in infarcted mouse hearts and human cardiomyopathic hearts. Cardiomyocyte apoptosis is downregulated and infarct area is halved after I/R injury in transgenic mice lacking TLR-4. In contrast, TLR-2–deficient mice displayed similar infarct sizes and inflammatory cell invasion after MI, but reduced long-term remodeling. In vitro, the activation of TLR-2 by ROS and of TLR-4 by lipopolysaccharide reduced death in cultured cardiomyocytes via adaptor protein MyD88 and IL-1 receptor–associated kinase. Hence, ROS, TLR-2, and TLR-4 may have diverse effects on cardiomyocytes. The nature of TLRs’ contribution to cell death during MI remains to be clarified.
Nitric Oxide
Nitric oxide synthases (NOSs) form a group of three enzymes, NOS1 (neuronal NOS), NOS2 (inducible NOS), and NOS3 (endothelial NOS), which catalyze the conversion of l -arginine to l -citrulline and nitric oxide (NO). NO is a multipotent mediator that can promote death or survival, depending on its concentration and mechanism of production. NOS2 increases significantly in 48-hour-old infarcts coinciding with inflammatory cell invasion, and persists for up to 14 days. In vitro, the combination of neutrophil and macrophage cytokines TNF-α, IL-1β, and IFN-γ triggers cardiomyocyte NOS2 expression and the generation of large amounts of NO. Selective NOS2 inhibition in infarcted hearts decreases inflammation and improves recovery, supporting the concept that excess NO produced by inflammatory cells is noxious for cardiomyocytes and tissue injury. NO produced by NOS2 activity further downregulates the expression of the antiapoptotic protein, stimulates Bax, and induces apoptosis in MI. Excessive NO triggers mitochondrial permeability transition (MPT) pore opening and cytochrome C release and initiates caspase-activation. NO can react with ROS to form peroxinitrite, which reacts with proteins, lipids, and nucleic acids, modifies the redox (reduction-oxidation) potential of the intracellular environment and promotes apoptosis. More specifically, NO and peroxinitrite inhibit SOD activity and enhance superoxide accumulation and cell damage. On the other hand, NO produced in normal conditions via basal NOS1 and NOS2 activity may be protective against cardiomyocyte apoptosis and inflammatory invasion. NO also upregulates Bcl-2 expression and blocks caspase-3 activation. NOS3 is expressed in cardiomyocytes and cardiac endothelium, where it mediates large-vessel vasodilation, inflammatory cell recruitment, and angiogenesis. NOS3-deficient mice display larger infarcts after MI and I/R. , This is consistent with the direct and indirect roles of NO in cardiomyocyte survival and angiogenesis after MI, in delayed cardioprotection after ischemia- and anesthetic-induced preconditioning, and increased inflammation and larger infarct size after I/R in NOS2-deficient mice. The ultimate influence of NO on cardiac death may thus depend on the local levels of ROS, as well as the timing and intensity of its release.
Proinflammatory Cytokines
Proinflammatory cytokines secreted by neutrophils and macrophages (TNF-α, IFN-γ, IL-1) contribute to cell death in ischemic hearts. IL-1 and TNF-α are early inflammatory cytokines released after MI. TNF-α is upregulated in human cardiac infarcts, with levels reaching peak values after 5 to 7 days, which correlates with increased caspase-8 activity and cardiomyocyte apoptosis. Cardiac death contributes to complement cascade activation, ROS generation, and local TNF-α release, which upregulates the secretion of multiple cytokines. Cardiomyocytes are insensitive to physiologic levels of TNF-α, but high concentrations enhance the cardiomyocyte expression of proapoptotic Fas ligand (FasL) and Bim and accelerated Fas-mediated apoptosis. TNF-α can also stimulate the apoptosis of endothelial cells and has deleterious effects on the vasculature. TNF-α–deficient mice show suppressed inflammation after MI, reduced cardiomyocyte death, and long-term cardiac dysfunction. Direct administration or forced adenoviral expression of soluble recombinant TNF-α receptor (TNFR) inhibits TNF-α signals and reduced cardiomyocyte apoptosis and cardiac dysfunction, supporting an early role for TNF-α in ischemic cardiac cell death. Cardiac reperfusion further stimulates TNF-α expression in association with a depressed Bcl-2/Bax ratio, increased cytosolic caspase-8 and caspase-3 activity, and cardiomyocyte apoptosis. In contrast, other investigators have reported that TNF-α could mediate cardiomyocyte survival. Gene therapy studies and overexpression of soluble TNFR blocked TNF-α signaling and promoted cardiac rupture in infarcted mouse hearts. TNFR-1/TNFR-2 double-receptor knockout mice had a larger infarct and more cardiomyocyte apoptosis after MI. TNFR-1 signals may be deleterious, mediating cardiac dysfunction, whereas TNFR-2 signals may be cardioprotective. Thus, TNF-α appears to be a key regulator in ischemic cardiac death, with opposite biologic effects. Inhibition of IL-1 signaling with an IL-1 receptor antagonist (IL-1ra) blocks the effects of IL-1α and IL-1β, reduces caspase-9 activity, and protects cardiomyocytes from apoptosis after MI, but fails to modulate infarct size. Viral delivery of the IL-1ra gene was successful in reperfused mouse hearts in reducing infarct size by 40% and apoptotic cardiomyocytes by 50%, and blocking the upregulation of Bax, Bak, and caspase-3, suggesting an important role of IL-1 in the apoptosis that follows I/R.
TNF-α, IL-1β, TLR, the complement cascade, and ROS are all known to activate nuclear factor kappa B (NF-κB) in multiple cell types on cardiac injury. This nodal transcription factor upregulates inducible chemokines and cytokines synthesis in infarcted hearts, and primes innate immune responses and exudative inflammation. However, NF-κB activation in leukocytes during the resolution phase of inflammation upregulates anti-inflammatory gene expression and apoptosis. Transgenic mice deficient for p50, a key subunit of activated NF-κB, are partly protected against MI injury. NF-κB can also upregulate inhibitor of apoptosis protein (IAP) and Bcl gene expression in certain cell types. Cardiac-restricted expression of a mutated IκB protein in transgenic mice prevented nuclear translocation of NF-κB, enhanced myocyte apoptosis, and increased infarct size after MI, suggesting that NF-κB directly contributes to cardiomyocyte survival. NF-kB, like TNF-α, can trigger cytoprotective and apoptotic pathways and lead to contrasting findings in the heart. Both IL-1R and TNF-α pathways induce the long pentraxin PTX3 transcript in ischemic hearts , and in serum after I/R injury. PTX3-deficient mice show decreased capillary density and increased apoptotic cardiomyocytes after I/R, associated with increased complement cascade activation. PTX3 may thus be a cardioprotective mediator of TNF-α and IL-1 signaling. Other soluble TNF-α family proteins, including TRAIL and CD40L, have been implicated in the development of MI. Enhanced expression of TRAIL was observed in circulating monocytes in MI patients, and human cardiomyocytes express the death domain–containing TRAIL receptor-1 and -2, suggesting that the TRAIL system may participate in ischemic cardiac death. CD40L is also increased in the circulation of MI patients, but the relative contributions of TRAIL and CD40L in cardiac death after MI remain unclear. Cytokines of the IL-6 family, such as IL-6, cardiotrophin-1 (CT-1), and leukemia inhibitory factor (LIF) are induced after MI and have profound effects on cardiac myocytes by protecting them from apoptosis. For example, CT-1, originally isolated as a promoter of hypertrophy, was subsequently found to reduce cardiomyocyte apoptosis in I/R injury in rats. Experimental administration of CT-1 resulted in decreased infarct size, and forced expression of LIF prevented cardiomyocyte death and induced angiogenesis. However, gene deletion of CT-1 or IL-6 does not modulate infarct size in mice after I/R injury, suggesting that alternative endogenous signals may compensate for their protective role. ,
Hematopoietic Factors
Hematopoietic growth factors such as granulocyte colony-stimulating factor (G-CSF) and monocyte colony-stimulating factor (M-CSF) are expressed in ischemic hearts. The precise function of these growth factors is believed to promote inflammatory cell differentiation and function but remains mostly unknown. However, G-CSF can act directly on specific cardiomyocyte receptors to promote their survival. G-CSF induced antiapoptotic Bcl-2 and Bcl-xL (B-cell lymphoma protein X, long isoform) expression and protected cardiomyocytes against ROS-induced apoptosis in vitro and I/R injury in mice. G-CSF also reduced apoptosis of endothelial cells and increased vascularization in infarcted hearts. This is supported by the finding that treatment with G-CSF, stem cell factor (SCF), and other growth factors promotes mobilization of endogenous bone marrow progenitor cells, improves function, and attenuates left ventricular remodeling following MI. M-CSF is stimulated in healing canine infarcts and its expression is associated with macrophage accumulation and proliferation. M-CSF does not directly reduce infarct area after MI, but stimulates vascular endothelial growth factor (VEGF) production, infarct vascularization, and reduced ultimate remodeling after I/R.
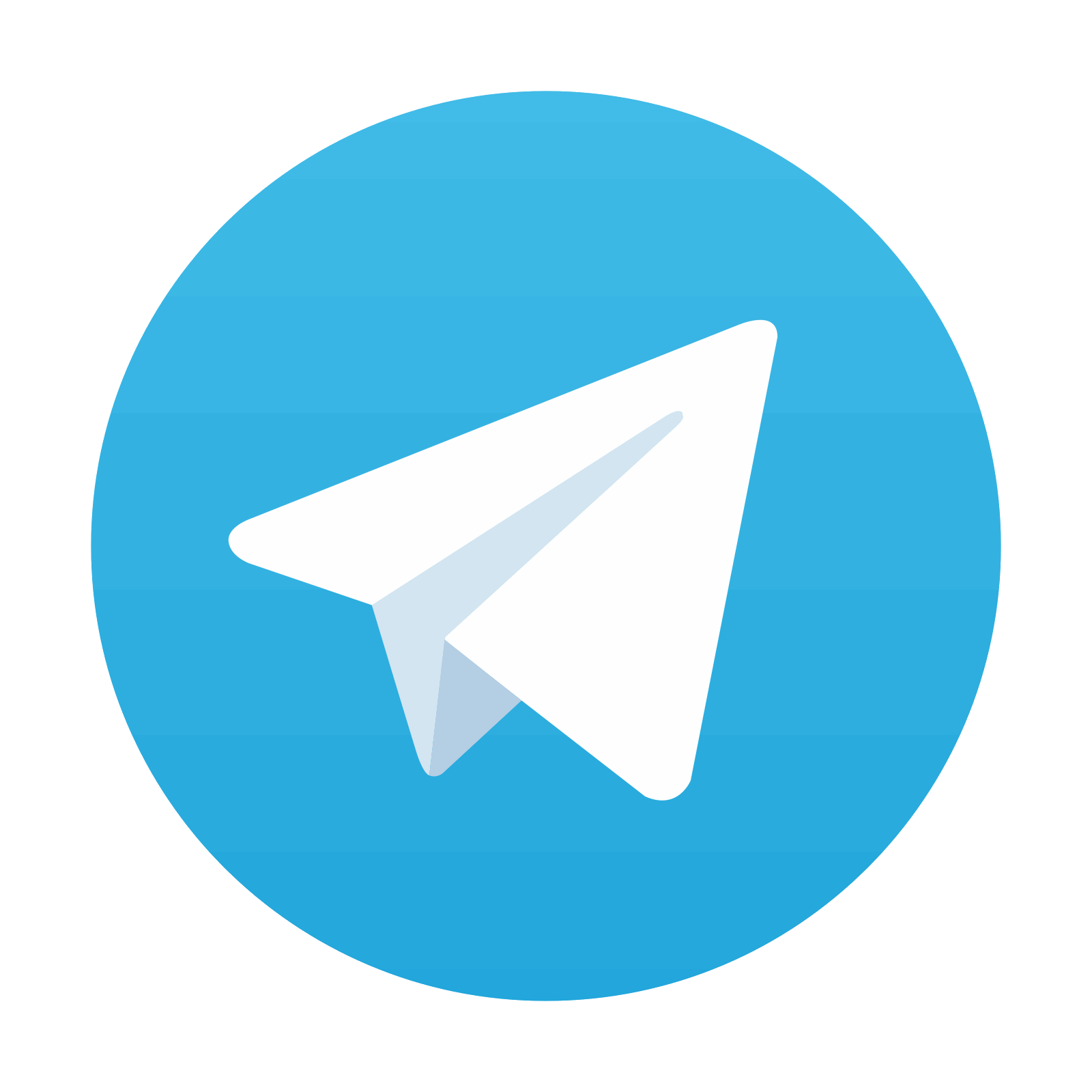
Stay updated, free articles. Join our Telegram channel

Full access? Get Clinical Tree
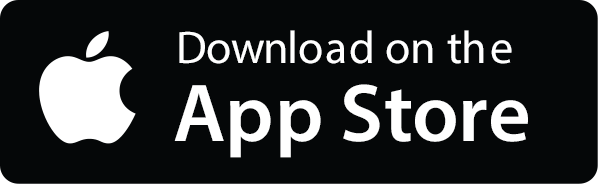
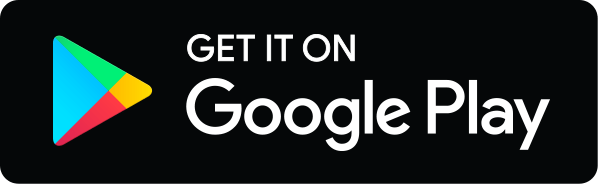