CHAPTER | ||
27 | Multielectrode Epicardial Mapping | |
Jakub Sroubek, MD; Elad Anter, MD |
INTRODUCTION
The rapid emergence of high-resolution mapping technologies, including multielectrode catheters, has derived from both an intellectual necessity to gain better insights into mechanisms and a clinical need to expedite mapping time. The reduction in electrode size, the closer interelectrode spacing, and a design favoring acquisition of simultaneous electrogram in contact with tissue have resulted in increased mapping resolution and speed.1–7 In particular, these catheters have been shown to identify surviving subendocardial myocardial bundles that could not be recognized with standard ablation catheters.
The basic principles underlying epicardial multielectrode mapping parallel the tenets of multielectrode endocardial mapping. In other words, just like endocardial maps, multiple electrograms can be collected simultaneously at each beat to construct high-density voltage and activation maps (during sinus rhythm, paced rhythm, or arrhythmia) that may be useful for mapping activation or a tachycardia to identify its arrhythmogenic substrate. In this regard, the increased mapping density allows to calculate endocardial conduction velocity that can be used to identify areas of slow conduction, susceptible for reentry.8 However, the anatomic differences between the endocardium and epicardium deserve special attention in respect to multielectrode mapping catheters.
First, the pericardial space is contained between the parietal and visceral pericardial layers. This narrow space (in the absence of fluid) allows excellent contact of linear catheters with the epicardial surface of the heart as compared to the endocardium where the proximal electrode is often in less contact. Furthermore, as the contact angle is increased from 0 degrees (parallel) to 90 degrees (perpendicular), the bipolar signal intensity reduces and the electrogram resembles a unipolar potential. In the empty pericardial space, the contact angle of both electrodes is ~0 degrees, producing a true bipolar electrogram with relatively higher amplitude and fidelity to identify near-field low-amplitude potentials. However, this advantage may be offset by the relative abundance of adipose tissue on the epicardial layer of the heart, which may result in more profound voltage amplitude reduction of multielectrode mapping catheters that have smaller electrodes compared to ablation catheters. This fundamental knowledge in electrogram recording and the variables affecting signal amplitude and shape can be used to choose an appropriate catheter depending on the underlaying substrate and mapping needs.
Second, catheter design is critical for epicardial mapping. While Decapolar and Duo-Decapolar catheter designs are the preferred design due to the abovementioned discussion, PentaRay (PentaRay™, Biosense Webster, Irvine, CA) and basket catheters (Orion™, Boston Scientific, Marlborough, MA) have not been optimized for this purpose. The PentaRay splines tend to cluster together such that electrodes often touch one another, while the basket catheter can only be used in a collapsed fashion, limiting its advantages compared to endocardial mapping. Furthermore, the stiff design of the basket and the sharp edges of the spines are not optimal for mapping in the pericardial space. On the other hand, the high-resolution rectangular Advisor mapping catheter (HD Grid, Abbott, St. Paul, MN) seems particularly well-suited for the pericardium. The latter has multiple small, closely-spaced electrodes and is believed to improve the validity of 3D mapping. In addition to rapid collection of data points, this catheter allows for bipole recording both along and across its splines to account for directionality.
HISTORICAL PERSPECTIVES
The first modern reports of in vivo epicardial maps in humans (aside from cases done in the setting of open-heart surgery) come from Sosa et al.9,10 The group used a standard 8-Fr introducer catheter via a posterior subxiphoid approach and then generated point-by-point maps using either using a standard 7-Fr steerable catheter (Webster-Mansfield, Watertown, MA) or Marinr multi-curve deflectable ablation catheter (Medtronic, Minneapolis, MN). With these linear quadripolar catheters, they were able to sample electrograms from the entire ventricular epicardial surface without having to expand the pericardial sac in any way. Their technique forms the basis of most subsequent epicardial mapping ventures. Marchlinski and colleagues11
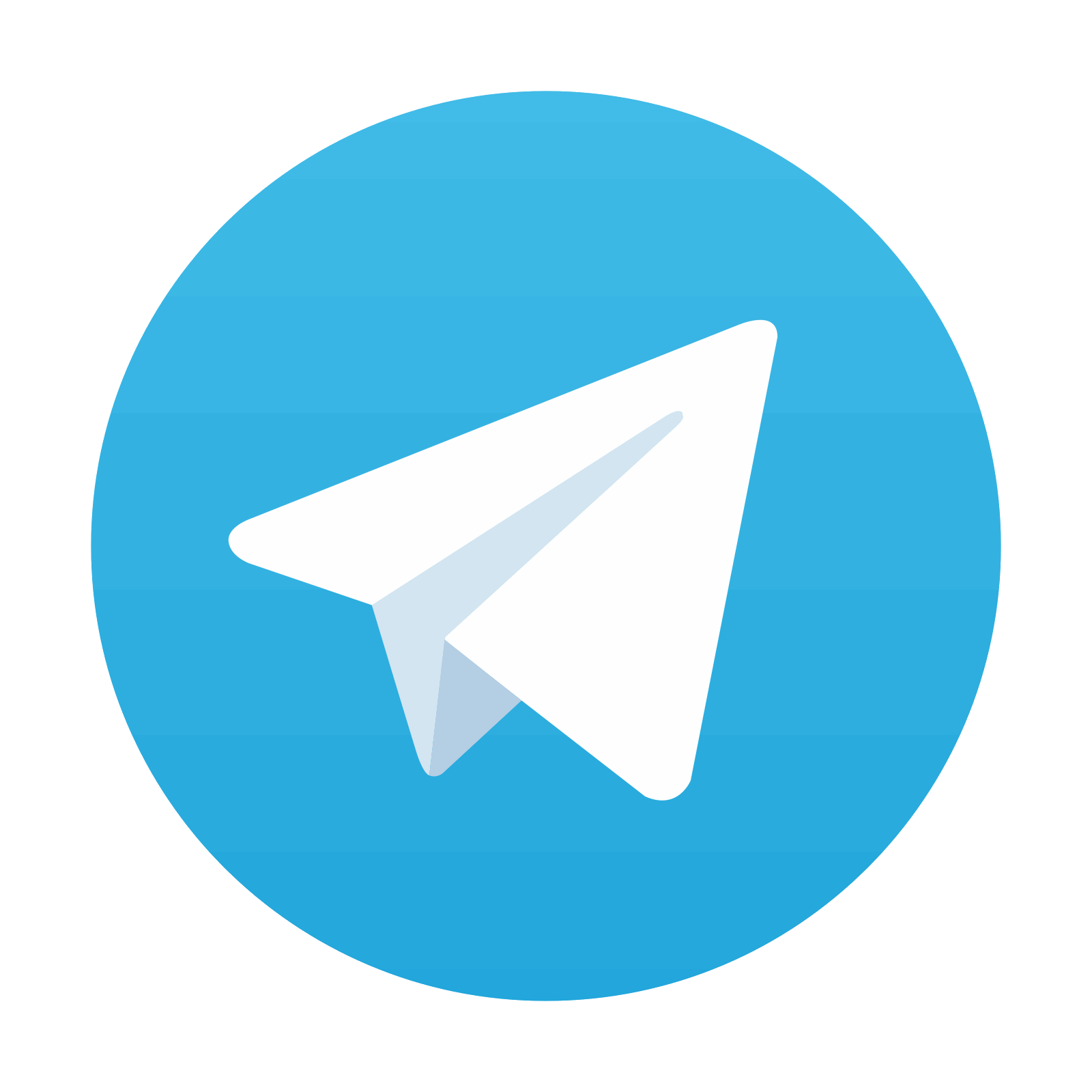
Stay updated, free articles. Join our Telegram channel

Full access? Get Clinical Tree
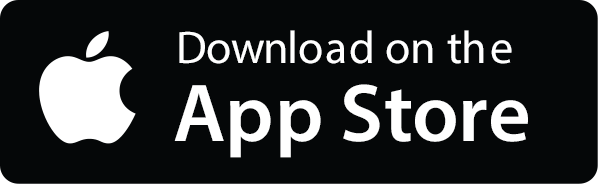
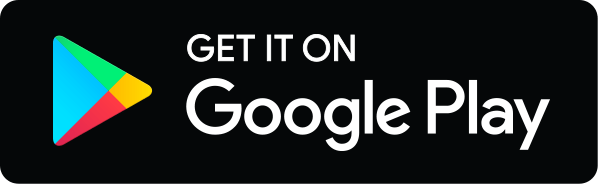