CHAPTER | ||
41 | Alternate Irrigation Solutions for Radiofrequency Ablation and Alternative Energy Sources | |
Pierre Qian, MBBS, PhD; William Sauer, MD |
INTRODUCTION
Catheter ablation in the epicardial environment introduces technical challenges that may limit ablation safety and efficacy. While radiofrequency (RF) ablation has become standard for endocardial ablation, the use of catheters designed to target tissue exposed to actively circulating blood flow can be problematic in the epicardium. The epicardial location of coronary arteries often limits the ability to deliver RF near these structures. In addition, the apposition of the pericardium in close proximity to an RF-emitting electrode can often result in pericarditis or phrenic nerve injury. Therefore, the development of alternative approaches using RF and non-RF energy sources has been pursued by several investigators for improving the safety and efficacy of epicardial ablation.
STANDARD RF ABLATION EXTERNALLY COOLED WITH LOW-IONIC IRRIGANTS
Solid-tip RF ablation catheters are ineffective in the epicardial space because there is minimal cooling that occurs without the presence of active blood flow. Therefore, externally irrigated catheters are frequently used to deliver RF. As discussed in Chapter 40, the accumulation of standard saline irrigant has been shown to lead to reduced lesion size and thus removal of the fluid from the pericardial space is typically performed. One reason for less effective lesions observed when saline is allowed to accumulate in the pericardial space is from the loss of RF current to a low-impedance environment. RF electrical current will follow the path of least resistance and therefore will be shunted away from tissue targeted for ablation when a catheter tip is surrounded by a low-impedance fluid.1 However, if a high-impedance fluid with low-ionic tonicity, such as dextrose in water (D5W) or half-normal saline (HNS), surrounds an electrode, the RF will be preferentially shunted to the lower-impedance tissue targeted for ablation.
Figure 41.1 shows the basic physical principles of how the use of low-ionic irrigant can lead to improved efficiency of RF delivery to tissue. Using this basic principle, our lab evaluated the effect of different irrigants used for cooling an active RF electrode, including HNS and D5W in an ex vivo setting.1 The results from controlled experiments using viable bovine myocardium revealed larger lesions when low-ionic irrigant was used to cool an iridium-platinum electrode delivering between 30 and 50 W of RF energy. Not surprisingly, a larger number of steam pops were observed in the HNS group, though this did not achieve statistical significance. The lesion set created using D5W irrigation and 50 W for 30 seconds with the catheter tip oriented in the perpendicular position had a very high incidence of steam pops, and 50 of 80 lesions exhibited cavitation.
Using a porcine in vivo model, externally irrigated epicardial ablation was performed at 50 W for 30 seconds using D5W versus standard NS irrigation. D5W ablation produced larger lesions compared to standard NS irrigated ablation, 157.1 ± 50 mL versus 56.9 ± 24.2 mL (P value = 0.009).1
Figure 41.1 Low-ionic fluids can facilitate epicardial ablation while sparing injury to the pericardium. During radiofrequency (RF) epicardial ablation, the blood pool acts as the predominant low-resistance pathway, an RF “ground “plane. During standard irrigated RF ablation, current loss to the accumulating normal saline (NS) irrigant fluid in the pericardial space is detrimental to myocardial ablation (Panel A); this effect is minimized and lesion size is increased if continual aspiration of pericardial fluid is performed (Panel B). In contrast, the use of low-ionic catheter irrigation fluid during RF ablation such as HNS instead of NS acts as a relative electrical insulator, shunting current towards the myocardial tissue and increasing lesion size while also displacing the pericardium, potentially reducing pericardial injury (Panel C).
In a separate study specifically evaluating the environmental impedance surrounding an RF electrode, the effect of a low-ionic solution enveloping the RF catheter tip appeared to be larger than what is observed in an endocardial model.2 Using an ex vivo model and an in vivo thigh preparation model, we demonstrated a significantly larger lesion associated exclusively with the reduced ionic content of the fluid surrounding a catheter after controlling for force, power, and duration of RF applications. The clinical implication given by these results was that HNS should be used in the epicardial space to prevent RF current shunting that will occur to a greater extent when lower-impedance standard saline irrigant is used. Interestingly, a small number of steam pops were observed with high-power RF applications, but this slightly higher incidence did not achieve statistical significance in the ex vivo model. There were no steam pops observed in the porcine thigh prep using an HNS-circulating bath despite the use of high power. The results of this study were further corroborated in a separate study evaluating the use of HNS in an ex vivo model designed to replicate bipolar ablation with an RF electrode in the epicardium with a return electrode placed in the endocardium.3
Given the strength of these ex vivo and in vivo animal studies, a group of electrophysiologists from 13 different centers used this novel strategy for ablation of ventricular arrhythmias that were resistant to standard RF ablation using NS as an external irrigant.4 The use of HNS was evaluated as a bail-out strategy when standard ablation failed to eliminate a targeted ventricular arrhythmia earlier in the same ablation session. The reasons for ablation failure were entirely due to lack of lesion depth using standard methods of ablation. The location of the arrhythmias targeted tended to be in places that are often the most challenging: the left ventricular summit, the base of the left ventricular papillary muscles, and intramyocardial scar in patients with nonischemic cardiomyopathy.
Of note, there were 28 patients who underwent epicardial RF ablation using HNS in this series of patients. Remarkably, of these, there was only one case of mild pericarditis observed. This low incidence of pericarditis may be due to another potential benefit of leaving fluid in the pericardial space. As low-ionic irrigant fluid accumulates, the pericardium is spared direct contact with the RF emitting electrode and thus may avoid injury that it might otherwise endure when fluid is actively removed, as can be appreciated in Figure 41.1.
Although this small case series is a step forward for understanding the safety and efficacy of the use of HNS, more data is needed. A randomized study evaluating HNS vs. NS is planned for ventricular arrhythmias. This study will also add to our understanding of this ablation strategy for epicardial tissue. In addition to the use of HNS or 5% dextrose solutions to enhance epicardial ablation, there is also an emerging body of literature evaluating alternative energy sources for epicardial ablation.
ALTERNATIVE ENERGY SOURCES FOR EPICARDIAL ABLATION
The types of alternative energy sources available for epicardial ablation are listed in Table 41.1, along with a brief summary of their pros and cons.
Cryoablation
Cryoablation causes tissue injury mediated by formation of extracellular and intracellular ice crystals with disruption of cell and organelle membranes as well as microvascular injury to produce sharply demarcated lesions with necrotic cores and eventual fibrotic replacement with minimal extracellular connective tissue architecture changes.5,6 Cryoablation may offer certain theoretical advantages in safety compared to RF ablation in percutaneous epicardial ablation due to better catheter stability, from cryoadhesion useful at sites close to coronary vessels and the phrenic nerve; potentially a low rate of permanent phrenic nerve palsy if phrenic nerve injury is acutely encountered as suggested by STOP-AF;7 low thrombogenicity when applied near coronary arteries; and ability to nonlethally ‘cryomap’ at −30° C to avoid unnecessary ablation. Cryoablation lesion dimensions correlate with nadir catheter tip temperature, catheter tip size and contact area, coolant flow rate, number of freeze-thaw cycles, and ablation duration.8–11 Percutaneous epicardial application may result in much larger lesion volumes compared to endocardial ablations due to the absence of convective warming from the blood pool.10 Epicardial ablation in closed-chest models have demonstrated the potential for transmural atrial ablation using 30-mm but not 6-mm tipped ablation catheters12 and ventricular ablations up to a depth of 4.7 ± 2.2 mm, 5.0 ± 2.3 mm, and 6.0 ± 1.2 mm, using 6-, 8-, and 30-mm tipped catheters respectively.10,12,13 Ventricular ablation lesion dimensions are significantly attenuated by epicardial fat > 2 mm and large epicardial vessels which provide convective warming.10 Cryoablation directly over an epicardial coronary vessel causes acute interruption of coronary flow due to freezing that resumes on thawing without acute histological vascular injury or thrombosis; however, neointimal hyperplasia with moderate focal coronary artery stenosis may be observed 6 months postprocedure with a tendency to spare larger coronary arteries.10,12,14,15 Clinically significant coronary injury to the left anterior descending following surgical ablation of LV summit VT after surgical cryoablation requiring percutaneous intervention has been described.16
Irreversible Electroporation
When cell membranes are exposed to sufficiently strong electrical fields for short periods of time (nanoseconds to milliseconds), transient nanometer-sized pores form within lipid bilayers large enough to permit the unregulated passage of macromolecules, a phenomenon termed electroporation. Depending on electric field strength, the pulse duration, frequency, and number, as well as cellular and tissue properties, this perturbation may lead to cell death via apoptosis (irreversible electroporation) or recovery (reversible electroporation).17,18 Reversible electroporation has been widely used to introduce substances otherwise impermeable to cells, notably chemotherapeutic agents and genetic material. Irreversible electroporation has gained recent interest in tumor and cardiac ablation. By limiting peak voltage density and tailoring voltage curves and pulse duration, electrical arcing may be avoided and thermal and electrolytic effects controlled to potentially facilitate ablation efficacy while preventing deleterious outcomes originally associated with direct-current catheter ablation.19–24
Electroporation produces controllable depths of myocardial tissue injury and a tendency to preserve tissue architecture with a low risk of coronary artery injury, pulmonary vein stenosis, phrenic nerve palsy, and esophageal injury in animal models.12,14,25–35 Risk of ventricular fibrillation induction and unwanted skeletal muscle contractions from electrical stimulation will likely require QRS gating and general anesthesia with paralytic agents. By pulsing high-voltage electrical fields with periodicity in the nano- to microsecond range, more homogenous lesions might be achievable in tissues with conductive heterogeneities while also avoiding skeletal muscular contractions common with a high-energy single pulse.36,37 Promising early clinical results has been seen using basket and linear electrode arrays for pulmonary vein isolation in both endocardial and surgical epicardial applications.32,38 Percutaneous epicardial ventricular electroporation using a different circular electrode array and a single cathodal defibrillator shock up to 200 J produced homogenous fibrosis at 3 months postablation and no significant vascular injury even if directly applied over a coronary artery while encompassing the perivascular myocardium within the zone of injury.39 Lesions were created with width up to approximately 22 mm and mean depth of 11.9 ± 1.5 mm in a dose dependent fashion, though ablations over coronaries and epicardial fat appeared relatively attenuated with a median lesion depth of 6.4 ± 2 mm.40,41 Further studies will better establish the safety and efficacy of this emerging modality for cardiac ablation.
Table 41.1 Alternative Energy Sources for Percutaneous Epicardial Ablation
Laser Ablation
Laser (light amplification by stimulated emissions of radiation) is a phase-coherent emission of light at a single wavelength. Clinical applications of lasers have typically used wavelengths in the red to infrared spectrum emitted using a gas such as argon or carbon dioxide, solid-state media such as neodynmium:yttrium-aluminum-garnet (Nd:YAG), or semiconductor diodes. Shorter wavelengths are absorbed by blood and myocardium with very little transmitted and scattered useful for creating focal superficial ablation or cutting but predisposing to coagulum and charring, whereas longer wavelengths promote greater transmission and scatter through tissue enabling absorption and heating at deeper layers to generate larger volume lesions.42 The largest clinical experience of epicardial laser ablation dates to the era of surgical treatment of VT using either a Nd:YAG laser operating at 1064 nm at 15–20 W.43 More recently, an open-irrigated 8-Fr tip diode laser catheter operating at 1064 nm at 15–20 W for 10–30 seconds has been shown to have efficacy in transmural ventricular endocardial ablations without significant incident of surface overheating in vitro and in vivo.44,45 A laser balloon system for pulmonary vein isolation using a 980 nm diode laser at up to 12 W has also gained FDA approval and demonstrated comparable overall efficacy and safety to radiofrequency ablation with a lower incidence of PV stenosis (0% vs. 2.9%), albeit a higher rate of phrenic nerve palsy (3.5% vs. 0.6%).46,47
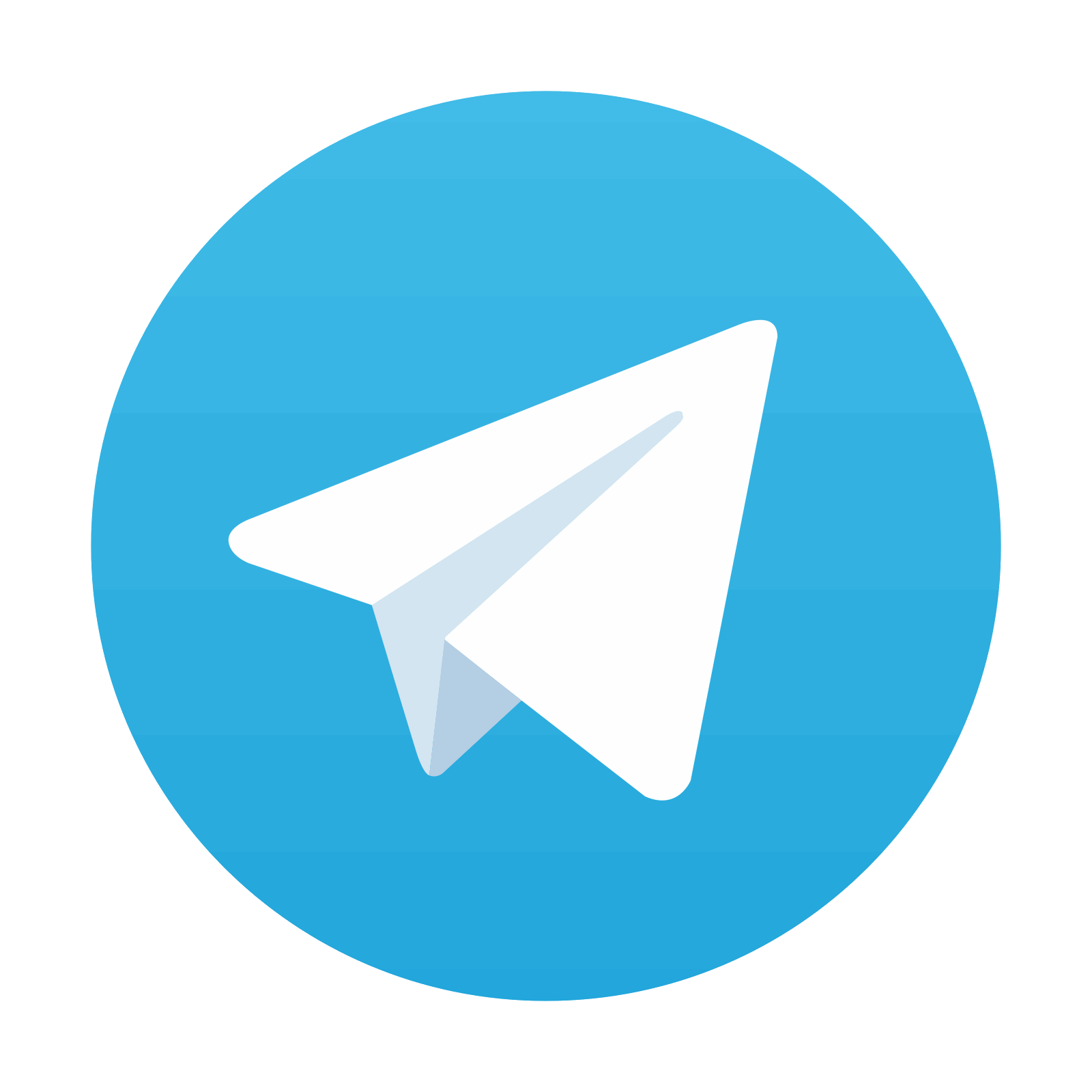
Stay updated, free articles. Join our Telegram channel

Full access? Get Clinical Tree
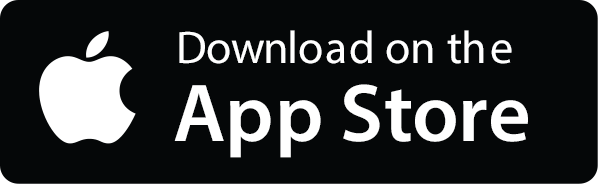
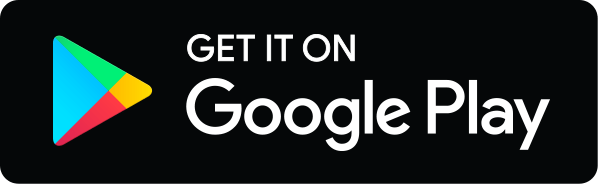