Patient radiation exposure in invasive cardiology is considerable. We aimed to investigate, in a multicenter field study, the long-term efficacy of an educational 90-minute workshop in cardiac invasive techniques with reduced irradiation. Before and at a median period of 2.5 months and 2.0 years after the minicourse (periods I, II, and III, respectively) at 5 German cardiac centers, 18 interventionalists documented various radiation parameters for 10 coronary angiographies. The median patient dose area product (DAP) for periods I, II, and III amounted to 26.6, 12.2, and 9.6 Gy × cm 2 , respectively. The short-term and long-term effects were related to shorter median fluoroscopy times (180, 138, and 114 seconds), fewer radiographic frames (745, 553, and 417) because of fewer (11, 11, and 10) and shorter (64, 52, and 44 frames/run) runs, consistent collimation, and restriction to an adequate image quality; both radiographic DAP/frame (27.7, 17.3, and 18.4 mGy × cm 2 ) and fluoroscopic DAP/second (26.6, 12.9, and 14.9 mGy × cm 2 ) decreased significantly. Multivariate analysis over time indicated increasing efficacy of the minicourse itself (−55% and −64%) and minor influence of interventionist experience (−4% and −3% per 1,000 coronary angiographies, performed lifelong until the minicourse and until period III). In conclusion, autonomous self-surveillance of various dose parameters and feedback on individual radiation safety efforts supported the efficacy of a 90-minute course program toward long-lasting and ongoing patient dose reduction.
Highlights
- •
Patient radiation exposure in invasive cardiology remains high. However, all existing radiation safety concepts have lacked consistent documentation of relevant dose parameters that characterize the underlying various individual radiation-reducing efforts by single cardiologists in clinical routine. Until now, there has been no multicenter long-term validation of an educational radiation-reducing program in clinical routine.
- •
This shortcoming is resolved by the presented educational “Encourage Less-Irradiation Cardiac Interventional Techniques” long-term approach: consistent collimation and realization of adequate image resolution, radiographic acquisition, and fluoroscopy time proved to be within the reach of every interventionalist, and if available, encouraged radiation-reducing technical settings—that is, preselectable pulse rates and detector entrance doses—that were evidently implemented over the years. A comprehensive, pseudonymized feedback for patient exposure results achieved at baseline and follow-up evaluations supported the significant efficacy of our minicourse and was accessible to each interventionalist by use of her or his individual code number.
- •
The interactive 90-minute minicourse presented here, designated “Encourage Less-Irradiation Cardiac Interventional Techniques”, enabled significant short-term (−54.6%) and—without further training—long-term (−64.1%) patient dose reduction from the marker intervention of coronary angiography. It consequently represents the first validation of the long-term efficacy of such a course in a multicenter field study (18 operators at 5 heart centers) in clinical routine. Multivariate linear regression analysis indicated—over the years—increasing efficacy of the minicourse itself and a minor and slightly decreasing influence of interventionists’ experience. Interventional workload after the minicourse did not influence long-term dose area product results.
- •
Nevertheless, extremely great differences among the cardiologists remained after the program. Our evaluation moreover illustrates both median values for dose parameters, realizable benchmarks, and borderstones for best practice. Because—over the course of a few coronary angiographies—the program qualifies operators to enable reliable self-monitoring and iterative radiation-saving improvements; it implemented a new culture of individual autonomy in radiation safety according to the “plan-do-check-act” principles of sustained quality management.
- •
In conclusion, autonomous self-surveillance of various dose parameters and feedback on individual radiation safety efforts supported the efficacy of a 90-minute course program toward long-lasting and ongoing patient dose reduction.
Since the early 1980s, the widespread use of radiation-emitting medical imaging and therapeutic action—over and above the average background radiation level in the United States (∼3.2 mSv/year)—has contributed to a sixfold increase (from ∼0.5 to ∼3.0 mSv/capita) in the annual average medical effective dose (ED). Cardiovascular tests, associated with the greatest radiation exposure and taken as part of this trend, include nuclear imaging, computed tomography, and percutaneous coronary interventions. Despite comprehensive state-of-the-art guidelines and training concepts on safe x-ray application, patient’s ED levels in invasive cardiology vary greatly —within 0.5 to 20 mSv for coronary angiographies (CA) and 2 to 57 mSv for percutaneous coronary interventions —and erythema, epilation, and ulceration may result. Chronically exposed cardiologists are susceptible to cellular redox imbalance, cell apoptosis, spinal pain, cataracts, and cancer —for example, brain tumors. Recently, the training program “Encourage Lower-Irradiation Cardiac Interventional Techniques” for CA in a multicenter field study has been validated as successful and effective, and in a single-center survey has indicated lasting efficacy. The presented multicenter 2-year follow-up investigation analyzes both the program’s long-term efficacy as well as influencing or confounding factors such as operator experience (in terms of CAs performed lifelong), patient’s body mass index (BMI), gender, and age.
Methods
Air kinetic energy released in matter at the interventional reference point (K A,R ; unit: Gray [Gy]) is the dose to air in the entrance plane of the patient and is independent of collimation. Skin dose includes backscatter in the upper skin layers and represents the most appropriate quantity for deterministic skin hazards. Dose area product (DAP; unit: Gy × cm 2 ) is the product of mean K A,R and the irradiated skin area. ED (with Sievert [Sv] as unit) is the sum of all weighted equivalent doses of exposed organs in the body and characterizes future stochastic cancer risks. DAP-to-ED conversion factors have been calculated at ∼0.20 mSv/Gy × cm 2 for the adult thoracic region.
Our study program was voluntary and received approval by the local institutional ethics committee. All patients and interventionalists were encoded. In accordance with German national radiation safety regulations, each operator had completed a theoretical 40-hour radiation safety course, an 8-hour special course in fluoroscopic guided interventions, and yearly 1-hour refresher courses. From 2003 to 2010, 18 cardiologists at 5 German cardiac centers before and at a median period of 2.5 months (interquartile range 1.9 to 3.4) and 2.0 years (1.9 to 3.1) after our minicourse performed 10 consecutive elective CAs, each by femoral access. Bypass grafts or significant valve diseases were excluded. Each interventionalist used the same equipment throughout the program; for example, traditional image-intensifier catheterization systems (11 operators; centers 1 to 4) or an advanced flat-panel detector system (operators 3, 6, 7, 9, 10, 13, 16; center 5; Table 1 ). Documentation included DAP, radiographic (DAP R ) and fluoroscopic (DAP F ) fractions, fluoroscopy time, the number of radiographic frames and runs, and—as parameters of dose intensity—DAP R /frame and DAP F /s ( Table 2 ). The 90-minute workshop within the centers addressed the following dose-reduction principles: (1) optimized time on beam, (2) consistent collimation, (3) copper filtering, (4) adequate pulse rates and detector entrance dose levels, (5) less irradiating angulations and adequate magnification, (6) full inspiration during radiography, (7) long source-to-skin and short patient-to-detector distances, and (8) sufficiently rested operators. Each of these steps toward improved radiation safety was discussed and illustrated by education videos. Before the minicourse and after short-term evaluation, all interventionists received anonymized feedback on their individual results, but participated in no additional training.
N | Years in the Cathlab | CA ∗ Until MC | CA ∗ Since MC | CA ∗ at Long-term Evaluation | Years Since MC | Center (1–5) | Technology | Company | Model |
---|---|---|---|---|---|---|---|---|---|
1 | 10-20 | 6000 | 2500 | 8500 | 3.5 | 1 | II | Philips | Integris H 5000 |
2 | 3-5 | 1200 | 1000 | 2200 | 2.5 | 2 | II | Philips | Integris H 5000 |
3 | 4 | 1000 | 550 | 1550 | 1,8 | 5 | FP | Siemens | Axiom Artis dFC |
4 | 5-10 | 3000 | 1500 | 4500 | 1.9 | 4 | II | Philips | Integris H 5000 |
5 | >20 | 15000 | 1000 | 16000 | 2.0 | 4 | II | Philips | Integris H 5000 |
6 | 6 | 2000 | 900 | 2900 | 1.9 | 5 | FP | Siemens | Axiom Artis dFC |
7 | 6 | 1800 | 600 | 2400 | 1.7 | 5 | FP | Siemens | Axiom Artis dFC |
8 | 10-20 | 10000 | 1000 | 11000 | 1.9 | 4 | II | Philips | Integris H 5000 |
9 | 1 | 420 | 1150 | 1570 | 3.8 | 5 | FP | Siemens | Axiom Artis dFC |
10 | 15 | 4200 | 250 | 4450 | 1.7 | 5 | FP | Siemens | Axiom Artis dFC |
11 | 5-10 | 3500 | 1500 | 5000 | 2.0 | 4 | II | Philips | Integris H 5000 |
12 | 10-20 | 2800 | 1200 | 4000 | 3.6 | 1 | II | Philips | Integris H 5000 |
13 | 9 | 8500 | 700 | 9200 | 1.9 | 5 | FP | Siemens | Axiom Artis dFC |
14 | 5-10 | 4500 | 1000 | 5500 | 1.9 | 4 | II | Philips | Integris H 5000 |
15 | 1-2 | 1200 | 2400 | 3600 | 3.1 | 3 | II | Philips | Integris H 3000 |
16 | 2 | 230 | 270 | 500 | 1.7 | 5 | FP | Siemens | Axiom Artis dFC |
17 | 3 | 1400 | 1800 | 3200 | 3.1 | 3 | II | Philips | Integris H 3000 |
18 | < 1 | 700 | 1600 | 2300 | 3.1 | 3 | II | Philips | Integris H 3000 |
Before Minicourse (B) | Short-Term Level (S) | Long-Term Level (L) | p Value (S vs. B) | p Value (L vs. S) | p Value (L vs. B) | |
---|---|---|---|---|---|---|
Operators | 18 | 18 | 18 | |||
Patients | 180 | 180 | 180 | |||
Centers | 5 | 5 | 5 | |||
Patient BMI (kg/m 2 ) | 27.7 (25.2–29.9) | 27.3 (24.9–30.5) | 27.6 (24.8–30.1) | 0.83 | 0.93 | 0.72 |
Patient age (yrs) | 64.6 (55.5–70.3) | 65.3 (57.3–71.1) | 66.5 (58.2–72.3) | 0.59 | 0.41 | 0.19 |
Patient sex, female (%) | 29.4 | 33.9 | 42.8 | 0.43 ∗ | 0.10 ∗ | 0.02 ∗ |
DAP (Gy × cm 2 ) | 26.6 (17.4–39.3) | 12.2 (6.8–19.1) | 9.6 (5.4–14.6) | < 0.001 | < 0.005 | < 0.001 |
DAP R (Gy × cm 2 ) | 21.7 (14.4–28.5) | 9.6 (5.2–14.4) | 7.6 (4.4–11.7) | < 0.001 | < 0.01 | < 0.001 |
DAP F (Gy × cm 2 ) | 5.1 (2.7–10.0) | 1.9 (1.0–4.7) | 1.7 (0.9–2.7) | < 0.001 | < 0.05 | < 0.001 |
DAP R /frame (mGy × cm 2 ) | 27.7 (20.8–37.9) | 17.3 (12.2–25.2) | 18.4 (12.3–25.0) | < 0.001 | 0.85 | < 0.001 |
DAP F /s (mGy × cm 2 /s) | 26.6 (15.7–42.3) | 12.9 (7.8–22.6) | 14.9 (8.7–24.0) | < 0.001 | 0.41 | < 0.001 |
Radiographic frames | 745 (572–908) | 553 (383–719) | 417 (323–572) | < 0.001 | < 0.001 | < 0.001 |
Radiographic runs | 11 (10–13) | 11 (9–13) | 10.0 (8–12) | < 0.05 | < 0.01 | < 0.001 |
Frames/run | 63.5 (53.5–75.0) | 51.5 (41.1–60.4) | 43.7 (35.4–52.2) | < 0.001 | < 0.001 | < 0.001 |
Fluoroscopy time (s) | 180 (114–338) | 138 (96–270) | 114 (78–174) | < 0.05 | < 0.001 | < 0.001 |
Values were expressed as median (interquartile range). Three groups (periods [p] I, II, and III) of non-normally distributed variables were analyzed by the 2-tailed Kruskal-Wallis test (significance level p <0.05) and by paired comparisons (period II vs I, III vs II, and III vs I) of results by the Mann-Whitney U test, including Bonferroni adjustment (p <0.05/3) and with use of SAS technology (version 9.1; SAS, Cary, North Carolina). Categorical data were compared by Fischer’s exact test. We performed multivariate linear regression analysis to rule out biasing effects of patient’s age, gender, and BMI, and of operator experience until the minicourse, until period III, and within the 2-year period after the minicourse. All parameters were coded dichotomously for short-term and long-term analyses. Normal distribution of residuals was tested using the Shapiro-Wilk test (p <0.1). Because the dependent original variables of regression models did not fulfill the normality assumption ( Table 3 ), we used log (ln) transformation to assure satisfactory statistical conditions for multivariate analysis. The relative variations of dose parameters, multivariately adjusted for the respective influencing variables, were obtained by exponential retransformation of the log regression coefficients ( Table 4 ).
Determinants Short-term Results (p II vs I) | Constant | BMI | Age (per Decade) | Sex (♂ = 1, ♀ =0) | Minicourse Participation | Experience † (per 1,000 CA) | ||||||
---|---|---|---|---|---|---|---|---|---|---|---|---|
p Value | c b | p Value | c a | p Value | c s | p Value | c p | p Value | c e | p Value | ||
Ln(DAP) (Gy × cm 2 ) | 0.94 | < 0.002 | 0.07 | < 0.001 | 0.08 | < 0.005 | 0.19 | < 0.006 | –0.79 | < 0.001 | –0.04 | < 0.001 |
Ln(DAP R ) ∗ (Gy × cm 2 ) | 0.67 | < 0.020 | 0.07 | < 0.001 | 0.06 | < 0.020 | 0.20 | < 0.002 | –0.81 | < 0.001 | –0.02 | < 0.004 |
Ln(DAP F ) (Gy × cm 2 ) | –1.04 | < 0.040 | 0.07 | < 0.001 | 0.13 | < 0.007 | 0.19 | 0.093 | –0.86 | < 0.001 | –0.09 | < 0.001 |
Ln(DAP R /frame) ∗ (mGy × cm 2 ) | 1.08 | < 0.001 | 0.07 | < 0.001 | –0.04 | < 0.030 | 0.20 | < 0.001 | –0.48 | < 0.001 | –0.02 | < 0.001 |
Ln(DAP F /s) (mGy × cm 2 /s) | 1.05 | < 0.002 | 0.07 | < 0.001 | 0.02 | 0.595 | 0.22 | < 0.005 | –0.67 | < 0.001 | –0.04 | < 0.001 |
Ln(Radiographic frames) ∗ ( n ) | 6.50 | < 0.001 | 0.00 | 0.754 | 0.02 | 0.236 | 0.00 | 0.961 | –0.33 | < 0.007 | 0.00 | 0.529 |
Ln(Radiographic runs) ( n ) | 2.44 | < 0.001 | 0.00 | 0.753 | 0.01 | 0.228 | –0.01 | 0.763 | –0.07 | < 0.001 | –0.02 | < 0.001 |
Ln(Frames/run) ∗ ( n ) | 4.06 | < 0.001 | 0.00 | 0.874 | 0.01 | 0.556 | 0.01 | 0.756 | –0.26 | < 0.001 | –0.01 | < 0.004 |
Ln(Fluoroscopy time) ∗ (s) | 4.82 | < 0.001 | 0.00 | 0.889 | 0.11 | < 0.002 | –0.03 | 0.732 | –0.20 | < 0.010 | –0.05 | < 0.001 |
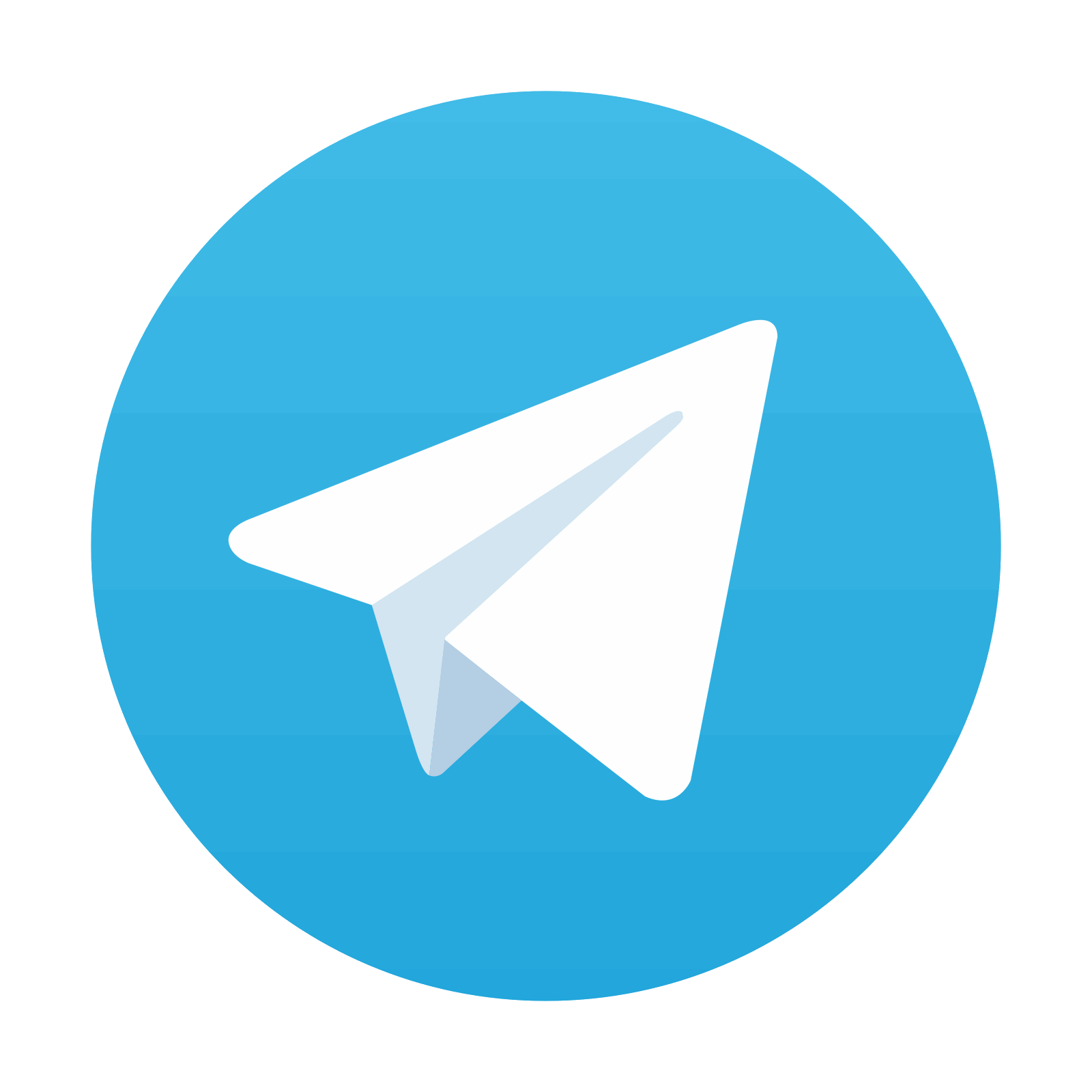
Stay updated, free articles. Join our Telegram channel

Full access? Get Clinical Tree
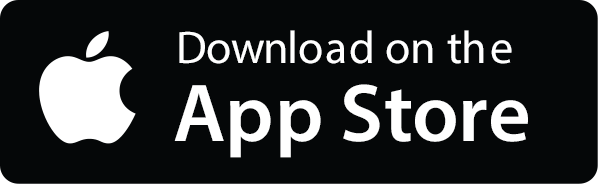
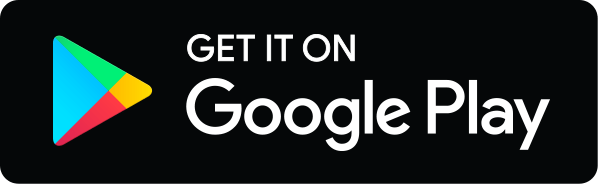
