Mouse Models of Lung Cancer
Ambra Pozzi
Lung cancer has increased in incidence throughout the 20th century and is now the most common cancer in the Western World. Compared to other cancers, the pathogenesis of lung cancer remains highly elusive because of its aggressive biologic nature and considerable heterogeneity. Most patients die of progressive metastatic diseases despite aggressive local and systemic therapies. To better understand the different steps in lung cancer progression and, most importantly, to devise more effective lung cancer therapies, there is considerable need for improved experimental models of lung cancer.
Currently, several animal species are widely used for experimental lung cancer research, including dogs, primates, hamsters, and mice.1,2,3 Among the different animal species, mice have become the preferred model to study lung cancer development and progression because of their relative cost-effectiveness, the ease of genetic manipulation, and the large number of genetically altered mice available for experimentation. Primary lung tumors in mice have morphologic, histogenic, and molecular features similar to human lung adenocarcinoma, particularly the bronchioloalveolar carcinoma subtype. Because of this, and because of the genetic homology between man and mouse, this model system is receiving intense research attention.
Three major different models are commonly used to induce lung cancer in mice, namely chemically and/or carcinogen-induced lung cancer, orthotopic models of lung cancer, and genetic models of lung cancer. In addition, spontaneous lung cancer develops in approximately 3% of mice and has a strain-dependent incidence in inbred mice (Fig. 13.1).4 Mice that develop spontaneous lung tumors also respond to chemicals and carcinogens, thus making them an ideal system to study chemically and/or carcinogen-induced lung cancer. Each of the mouse models available allows the analysis of different aspects of the disease, such as carcinogenesis, initiation, promotion, metastasis, as well as host-tumor interaction and angiogenesis. For example, the chemically induced lung cancer models allow the study of tumor initiation and promotion; the orthotopic model allows the analysis of primary as well as metastatic lung cancers, whereas the genetic model allows the identification of genes involved in lung cancer development and progression.
Despite the fact that these models reflect the histopathology and the steps involved in lung cancer progression, each of them has limitations. These include lack of metastasis (genetic-and chemically induced lung cancer), the development of tumors only late in their course of development (chemically induced lung cancer), and the development of only one subset of lung cancer, namely adenoma and/or adenocarcinoma. For these reasons, more than one model is used when studying the etiology, pathogenesis, and progression of lung cancer.
In this chapter, we will describe the main features of the available mouse models of lung cancer, and how these models can be used for translational research. We will mainly point out practical benefits, such as their application for identifying therapeutic strategies for the treatment and prevention of lung cancer.
CHEMICALLY INDUCED LUNG CANCER
Cigarette smoking represents the prominent cause of lung cancer. More than 20 lung carcinogens have been identified in cigarette smoke, and they can act as initiators and/or promoters of lung cancer by accelerating tumor onset and increasing tumor multiplicity.5 Although 85% of lung cancers are thought to be as a result of cigarette smoking, individuals exposed to asbestos, arsenic, nickel, radiation, and those with pulmonary fibrosis are also at increased risk.6 For this reason, chemically and carcinogen-induced animal models of lung cancer have been developed. They are used not only to identify possible molecules and/or environmental factors able to induce lung cancer but also to study the early stage of carcinogenesis and cancer progression. Chemically and/or carcinogen-induced lung tumors have been described in various species, including dogs, cats, ferrets, and mice.6 The susceptibility of mice to develop chemically and/or carcinogen-induced lung cancer is strain dependent. Mouse strains have been categorized into sensitive, intermediate, and resistant,7 based on the time of occurrence of lung tumors after
chemical exposure, and on the number of tumors. The A/J mouse strain belongs to the sensitive group with at least 20 times higher susceptibility than resistant strains like C57BL/6J and C3H/HeJ or intermediate strains like BALB/c. The propensity of these sensitive strains to develop lung tumors strongly correlates with a polymorphism in the second intron of the K- ras gene.8 The polymorphism is a 37-nucleotide-intronic sequence that is tandemly duplicated in resistant strains and is present as a single copy in sensitive strains. This polymorphism seems to confer different nuclear protein-binding abilities that may influence gene expression.9 In addition to the K- ras gene, the pulmonary adenoma susceptibility 1 (Pas1) locus on chromosome 6 has been implicated in the development of lung cancer in the A/J strain.10 Based on the fact that both the Pas1 and K- ras genes map on chromosome 6,11 it has been proposed that the Pas1 gene could be identical to the K- ras oncogene, and natural and/or chemically induced point mutation in Pas1 gene could lead to lung tumors similarly to what observed with the K- ras gene.8 When A/J mice are exposed to urethane, mutations in either one of these polymorphic loci occur, resulting in the development of benign lung adenoma within few months from the exposure.12 Some of these tumors can progress to adenocarcinomas with histopathology similar to that seen in humans.13
chemical exposure, and on the number of tumors. The A/J mouse strain belongs to the sensitive group with at least 20 times higher susceptibility than resistant strains like C57BL/6J and C3H/HeJ or intermediate strains like BALB/c. The propensity of these sensitive strains to develop lung tumors strongly correlates with a polymorphism in the second intron of the K- ras gene.8 The polymorphism is a 37-nucleotide-intronic sequence that is tandemly duplicated in resistant strains and is present as a single copy in sensitive strains. This polymorphism seems to confer different nuclear protein-binding abilities that may influence gene expression.9 In addition to the K- ras gene, the pulmonary adenoma susceptibility 1 (Pas1) locus on chromosome 6 has been implicated in the development of lung cancer in the A/J strain.10 Based on the fact that both the Pas1 and K- ras genes map on chromosome 6,11 it has been proposed that the Pas1 gene could be identical to the K- ras oncogene, and natural and/or chemically induced point mutation in Pas1 gene could lead to lung tumors similarly to what observed with the K- ras gene.8 When A/J mice are exposed to urethane, mutations in either one of these polymorphic loci occur, resulting in the development of benign lung adenoma within few months from the exposure.12 Some of these tumors can progress to adenocarcinomas with histopathology similar to that seen in humans.13
More recently, Stathopoulos and colleagues14 have elegantly shown that epithelial nuclear factor (NF)-κB activation facilitates urethane-induced lung carcinogenesis. In this context, mouse strains susceptible to lung tumor formation (i.e., FVB, BALB/c) exhibited early NF-κB activation and inflammation in the lungs after urethane treatment. In contrast, the resistant strain C57B6 failed to activate NF-κB or induce lung inflammation. Interestingly, selective NF-κB inhibition resulted in increased apoptosis of airway epithelial cells after urethane exposure, highly suggesting that NF-κB signaling in airway epithelium is integral to chemically induced tumorigenesis.14
Another common example of carcinogen-induced lung neoplasia is the classical two-step initiation/promotion model. Injection of the initiator 3-methylcholanthrene, followed by various exposures to the promoter butylated hydroxytoluene, has been shown to induce adenocarcinoma in BALB/c mice within 16 weeks from the exposure to the initiator. The first stage in this two-stage carcinogenesis procedure (initiation) induces an irreversible lesion in the DNA of a single cell, while the second stage (promotion) initiates cell clonally expands.
Mice are also used to assess carcinogenic activity of various chemicals, including benzopyrenes, metals, nitrosamines, and polyaromatic hydrocarbons.12 Biochemical effects of lung chemicals can be detected within hours or days of their administration. In strains susceptible to lung tumorigenesis, exposure to chemicals leads to rapid formation of hyperplastic foci in the bronchioles and alveoli. All or some of these foci then evolve into microscopic adenomas, and few months later, some of these adenomas display the nuclear atypia and invasiveness of adenocarcinomas in situ.15 In contrast, exposure of chemicals in resistant strains only leads to the development of few adenomas and/or papillary tumors within several months from the original exposure.
Given the high incidence of cigarette-induced lung cancer, many animal models have been used to determine the effect of cigarette smoke on lung cancer incidence, formation, and progression. 3 Despite the vast research conducted, it is debatable whether the measured response to cigarette smoke in animal species for assessing carcinogenic potential in humans reflects the strong epidemiological evidence in human smokers. In a recent review article, Coggins3 points out some of the pitfalls related to exposure of animal models to cigarette smoke. In this context, the cigarettes used in many studies were unfiltered and had very high yields; thus being very different from the cigarettes commonly smoked today. In addition, whereas some
studies used nose-only smoking machines, others used wholebody exposures. Moreover, whereas certain animal models were exposed to single cigarettes, others were exposed to rotating carousels. Finally, when dogs were used for studies, invasive tracheotomy technique was used to facilitate smoke breathing. As results, often lung necrosis and/or inflammation with no apparent neoplasm were evident. Thus, these studies not only do not entirely mimic the exposure of smoke as observed in humans, but also do not recapitulate the events of lung cancer initiation/formation. Lastly, studies performed in rats and mice exposure for lifetime to cigarette smoke suggested that, although both species developed alveolar epithelial hyperplasia, alveolar adenomas, and alveolar carcinomas, the incidence of all three were more evident in the rats. Thus, mice might not represent the most suitable model for smoke-mediated lung cancer.16
studies used nose-only smoking machines, others used wholebody exposures. Moreover, whereas certain animal models were exposed to single cigarettes, others were exposed to rotating carousels. Finally, when dogs were used for studies, invasive tracheotomy technique was used to facilitate smoke breathing. As results, often lung necrosis and/or inflammation with no apparent neoplasm were evident. Thus, these studies not only do not entirely mimic the exposure of smoke as observed in humans, but also do not recapitulate the events of lung cancer initiation/formation. Lastly, studies performed in rats and mice exposure for lifetime to cigarette smoke suggested that, although both species developed alveolar epithelial hyperplasia, alveolar adenomas, and alveolar carcinomas, the incidence of all three were more evident in the rats. Thus, mice might not represent the most suitable model for smoke-mediated lung cancer.16
Mice, however, have been successfully used to study susceptibility to lung cancer following exposure to environmental agents such as radiation and viruses.17,18 Although these two nonchemical models have the advantage that they are not strain dependent, they present the major disadvantage that tumors develop in various organs, beside the lungs, making the analysis of primary versus potential metastatic lung cancer more difficult to evaluate.
The observation that sensitive mice are more susceptible to chemically induced lung cancer became the basis for quantitative carcinogenicity bioassay12 and screening systems for chemopreventive agents.19 Cancer chemoprevention can be defined as the use of agents able to prevent, inhibit, or reverse the process of carcinogenesis.20 Various anti-inflammatory drugs such as indomethacin or aspirin have been used to lower chemically induced lung tumor development and multiplicity,21 and specific cyclooxygenase-2 inhibitors have been used to reduce the growth of adenocarcinoma after treatment with carcinogens.15 In addition, pretreatment of mice with drugs able to inhibit DNA methylation showed chemopreventive efficacy in primary mouse lung tumors induced by nitrosamines (see Chapter 7).22 Finally, the effect of natural products can be tested for their preventive effect on carcinogen-induced lung cancer. Kohno and colleagues23 identified chemopreventive factors (i.e., beta-cryptoxanthin and hesperidin) in commercial mandarin juice, able to suppress lung cancer initiated with nitrosamines in A/J mice. In addition, pretreatment with perillyl alcohol, a naturally occurring monoterpene found in lavender, cherries, and mint, 1 week before lung tumor initiation with nitrosamines, significantly reduced tumor incidence and tumor multiplicity, strongly suggesting that this monoterpene is an effective chemopreventive compound in mouse lung tumor bioassay.24
In summary, chemically and/or carcinogen-induced lung cancer models offer the major advantage that the induction of lung tumors is highly reproducible, and they can also be used to screen potential carcinogens as well as to identify chemopreventive agents. There are, however, disadvantages of these models. In particular, they are time consuming, strain dependent, lead primarily to the development of non-small cell lung carcinoma (NSCLC), allow the detection of lung tumors at late stage of progression, and lead to the development of tumors with low metastatic potential. Moreover, for study related to cigarette-smoke-induced lung cancer, mice might not represent the best animal models available. Finally, administration of chemicals or carcinogens can yield to various different tumor cell types, many of which might not be directly relevant to human lung cancer.
ORTHOTOPIC MODEL OF LUNG CANCER
Orthotopic models of cancers consist in the injection of tumor cell suspension as well as in the implantation of fresh tumor tissues directly into the appropriate organ of origin. Human tumors and/or human cancer cells can be orthotopically implanted in various organs, including stomach, colon, pancreas, prostate, mammary gland, bladder, and lung.25 The availability of immunodeficient mouse strains such as the nude mice, the Rag2-/- mice, and the severe combined immunodeficient (SCID) mice facilitated the establishment of human orthotopic models of cancer because of the inefficiency of these mice to reject human cells. The injection of tumor cells in the organ of origin clearly allows a better understanding of the role of the microenvironment in the development of primary tumors. In addition, a major advantage of this model is that it allows studying and recapitulating of the entire process of tumor progression consisting of local tumor growth, vascular and lymphatic invasion at the local site, flow in the vessels and lymphatic, extravasation at the metastatic organs, and seeding and growth at relevant metastatic sites. The availability of both human NSCLC and small cell lung carcinoma (SCLC) cells make the orthotopic model an attractive assay to study lung cancer growth and development as, at present, there is only one genetic mouse model of primary SCLC available (see discussion later). In addition, based on the observation that the microenvironment profoundly affects the phenotype and progression of many tumor types,26 the injection of lung cancer cells directly into the organ of origin may recapitulate the events of lung cancer growth and progression similar to those observed in humans. Several orthotopic injection routes have been developed for lung cancer, including intrabronchial,27,28,29 intrathoracic,30 intrapleural or intravenous,31 and direct injection of tumor cells32,33 as well as implantation of fragments of subcutaneously growing tumor tissues34 into the lung parenchyma of recipient mice. Of these models, the direct injection of tumor cells into the lung parenchyma represents an exceptionally rapid procedure with limited trauma to the mice and reduced intrapleural leakage of tumor cells. In addition, this method had been successfully used to produce a solitary tumor nodule in the lung followed by metastasis to the mediastinal lymph nodes. It is well recognized that the orthotopically growing tumors will grow and metastasize to organs similarly to the human situation. Thus, the presence of tumors in the contralateral noninjected lung, as well as presence of metastases in lymph nodes, liver, brain, and bones can be used to evaluate the metastatic efficiency of different lung cancer cell lines.33
One of the major issues related to cancer is the detection of micrometastases (single cell or clusters of fewer than 10 cells). To improve the visualization of tumors in different organs, tumor cells can be stably labeled with different fluorescent or bioluminescent markers such as green fluorescent protein (GFP)35 and luciferase.36 Single GFP-labeled tumor cells can be detected in freshly isolated organs with a fluorescence microscope,37 and the number of tumors growing on the surface and/or within the organs can be evaluated, and quantitative measurements can be obtained using computer software imaging programs.33 Retroviral delivery of GFP has been successfully used not only to label primary human tumors, but also to analyze regional and distant metastases.38 A significant advantage of using GFP- or luciferase-expressing cells is that tumors can be visualized and measured externally in live mice. Hoffman37 implanted highly metastatic human cell line expressing GFP into the left lungs of nude mice and then followed primary and metastatic growth in real time by analyzing the mice under fluorescence light. Similarly, Rosol and colleagues 39 injected into the left ventricle luciferase-labeled metastatic cells and followed in real time their localization in the lungs by using in vivo bioluminescent imaging. More recently, Acuff and colleagues29 have used luciferase-labeled human NSCLC cells to determine in real time the contribution of host-derived matrix metalloproteinases to the survival and the early establishment of tumors in the lung. Finally, noninvasive in real-time imaging has been recently employed to determine the effect of systemic delivery of capsid-modified adenoviruses in an orthotopic model of advanced lung cancer.40 Thus, the use of labeled cells clearly facilitates in vivo imaging as well as longitudinal studies as the same mouse can be monitored over time (Fig. 13.2).
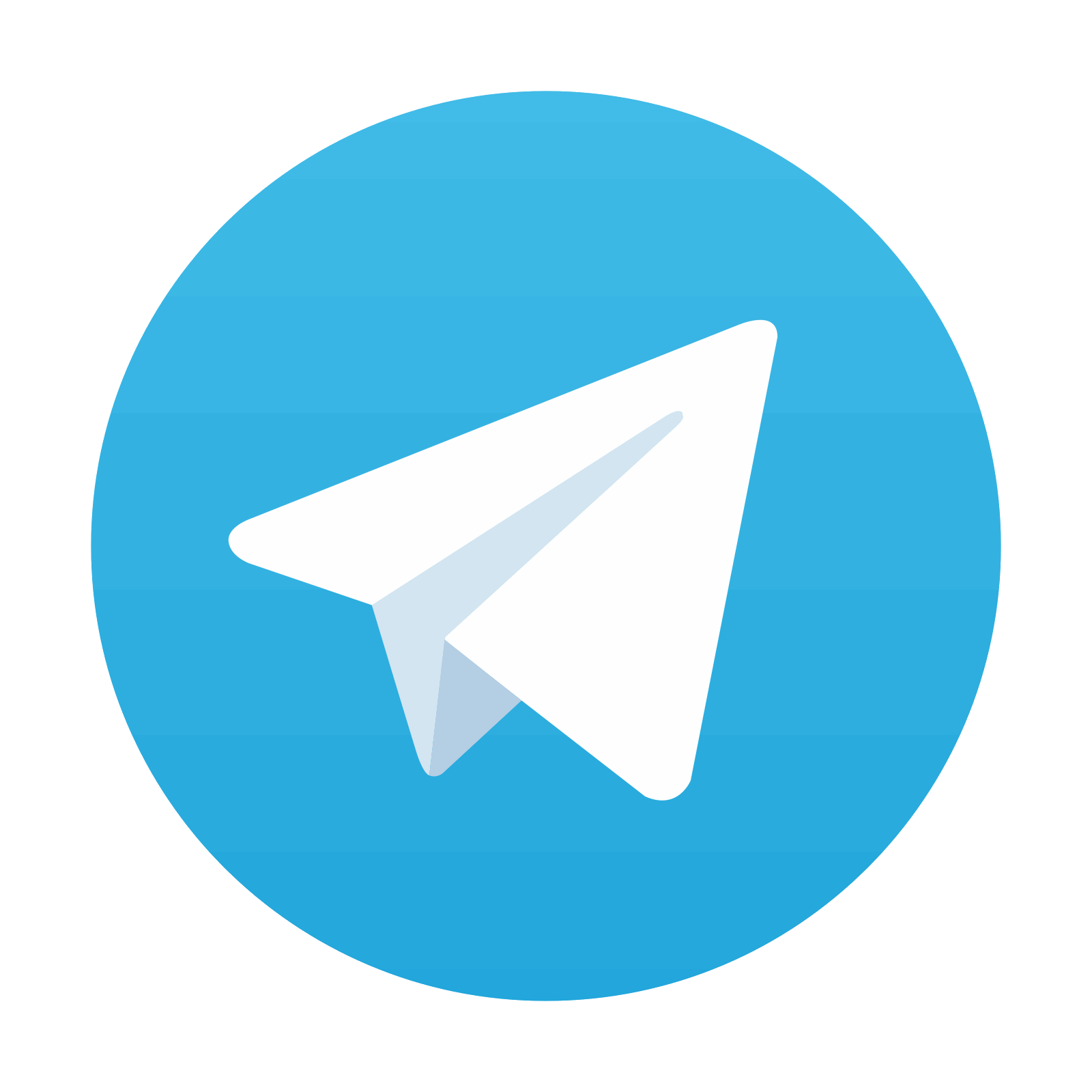
Stay updated, free articles. Join our Telegram channel

Full access? Get Clinical Tree
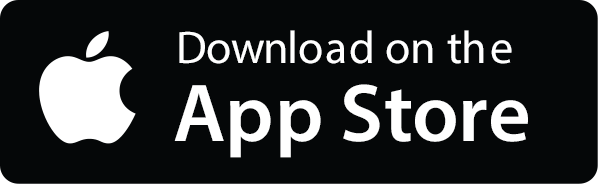
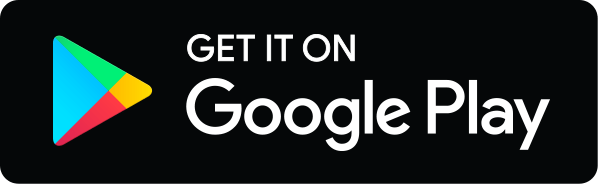