Abstract
This chapter describes the therapeutic strategies for cystic fibrosis which are based on targeting cystic fibrosis transmembrane conductance regulator (CFTR), either at the gene or protein level. We provide updates on small molecule CFTR modulators and gene therapy, focusing on clinical development and evaluation. The field has seen significant progress over recent years, particularly with the CFTR potentiator, ivacaftor, in patients with class III mutations. Increased understanding of the abnormalities in the structure and function of CFTR protein will help optimize the approaches required for normalizing function and, in doing so, aid the rational design of clinical trials—both in terms of the development of more efficacious drugs and the selection of appropriate patient populations. While progress with gene therapy remains some way behind, potential benefits (including being mutation agnostic and a nonsystemic route of delivery) remain significant. It may be that future optimal approaches will harness the benefits of more than one of these approaches and lead to considerable synergy. The ultimate goal for molecular and advanced therapies in cystic fibrosis is to find drugs or combinations of drugs capable of restoring CFTR function, applicable to patients with any genetic mutation.
Keywords
cystic fibrosis, CFTR modulators, gene therapy, clinical trials, outcome measures
Introduction
The impressive improvement in life expectancy in cystic fibrosis (CF) over recent decades has been achieved by treatments that are aimed at the downstream consequences of absent or dysfunctional CF transmembrane conductance regulator (CFTR) activity (including aggressive treatment of infection, airway clearance and nutrition) rather than by targeting CFTR directly. In contrast, novel approaches using CFTR modulators or gene therapy aim to target the root cause of the disease, replacing/repairing the CFTR gene or addressing the underlying defects responsible for defective CFTR channel function, intracellular processing, or production. Ultimately, the aim is to fully correct the defective CFTR and restore normal function. There is already some evidence that CFTR modulation can affect the disease trajectory in CF.
This chapter describes the therapeutic strategies for CF which are based on targeting CFTR, either at the gene or protein level. We will provide updates on small molecule CFTR modulators and gene therapy, focusing on clinical development and evaluation. The challenge for the field is to find drugs or combinations of drugs capable of restoring CFTR function, applicable to patients with any genetic mutation.
Small Molecule Cystic Fibrosis Transmembrane Conductance Regulator Modulators
The focus of this section is on CFTR modulators licensed for clinical use or in clinical trials at the time of writing. The wave of CFTR modulators currently in the initial stages of development or under evaluation in clinical trials will inevitably mean that for the reader to remain up to date, information in this chapter will need to be reviewed in conjunction with new evidence as it becomes available. Table 53.1 lists the drugs targeting CFTR, which are at clinical stages of testing. Clinical trials are registered on the US National Institutes of Health ClinicalTrials.gov website ( https://clinicaltrials.gov/ ). The US Cystic Fibrosis Foundation also has an interactive drug development pipeline on its website that takes the reader to an overview of evidence to date from clinical trials and trials currently in progress ( https://www.cff.org/Trials/Pipeline ).
Class of Drug | Agents | Current Status |
---|---|---|
Gene replacement therapy | pGM169/GL67A (UK CF Gene Therapy Consortium) | Primary end point met in phase IIb clinical trial (59) |
mRNA repair | QR-010 (ProQR Therapeutics) | Trials of nasal delivery and nebulization underway |
Read-through agents | Ataluren (PTC124; PTC Therapeutics) | Recent announcement of 2nd phase III trial failure http://ir.ptcbio.com/releasedetail.cfm?releaseid=1015471 |
Amplifier | PTI-428 (Proteostasis Therapeutics Inc) | Early-phase trials of oral agent underway |
Correctors (+/−potentiator) | Lumacaftor/ivacaftor (Orkambi, Vertex) | Licensed for F508del homozygous patients |
Tezacaftor (VX661)/ivacaftor (Kalydeco; Vertex) | Phase III program in F508del homozygous and heterozygous patients underway | |
Next-generation triple component drugs (Vertex) | Early-phase trials underway with several agents administered with 661/ivacaftor | |
Riociguat (Bayer) | Phase II trial underway | |
GLPG2222 and GLPG2737 (Galapagos) | Early-phase trials underway | |
FDL169 (Flatley Discovery Labs) | Phase II trial to commence 2017 | |
Potentiator | Ivacaftor (Kalydeco; Vertex) | Licensed for patients with gating mutations aged 2 years and older and R117H A component of all Vertex’s combination drugs |
GLPG1837 (Galapagos) | Phase II trial data awaited | |
QBW251 (Novartis) | Phase II study results awaited | |
GLPG2451 (Galapagos) | Early-phase trials as mono and in combination with GLPG2222 | |
GLPG3067 (Galapagos) | In phase I trial | |
Deuterated ivacaftor (CTP-656, Concert) | In phase II trial of patients with gating mutations | |
Stabilizer | Cavosonstat (N91115; Nivalis Therapeutics) | Recent announcement of failed phase II trial http://ir.nivalis.com/press-releases/detail/60 |
ENaC inhibitor | VX-371 (Vertex) | In phase II trial in patients on Orkambi (also being tested in primary ciliary dyskinesia [PCD]) |
Identifying Candidate Drugs
Drug development typically starts with identification of a therapeutic target, which may be site-, cell-, or receptor-specific. Candidate compounds are then investigated for their ability to modulate this target. These can be either selectively or randomly chosen; the latter clearly requires high-throughput screening techniques—automated assays that allow high numbers of potential compounds to be screened quickly. “Lead” compounds showing potential undergo further preclinical assessment (further information at http://www.cftr.info/drug-research-development/pre-clinical-development/pre-clinical-validation-of-cftr-therapies/ ). Optimization to improve selectivity of the desired target, pharmacokinetics, and preclinical toxicology follows. The agents that are considered promising at the end of this process may proceed to clinical trials. Both traditional and high-throughput screening processes have been used to identify potential compounds that target defective or absent CFTR. Understanding functional manifestations at the protein/molecular level of a particular disease-causing mutation in CF allows the use of assays in high-throughput screening that can identify any impact on CFTR function (e.g., assays measuring anion conductance across cell membranes act as a “readout” for CFTR function).
Impact of Cystic Fibrosis Transmembrane Conductance Regulator Mutations on Protein Expression, Maturation, or Function
A basic understanding of CFTR structure and function is important to understand the rationale for CFTR modulators. CFTR is a 1480–amino acid protein belonging to the adenine nucleotide binding cassette (ABC) transporter superfamily, expressed at the apical membrane of specialized epithelial cells. It has multiple functions, but its role as a chloride and bicarbonate transporter is best characterized. A detailed description of the consequences of absent or dysfunctional CFTR in CF is described in Chapter 49 .
More than 2000 mutations in the CFTR gene have been identified ( http://cftr2.org ), although the number determined as definitely disease causing is currently less than 200. The single residue deletion of phenylalanine at codon 508 (Phe508del) is the commonest mutation globally, present in almost 90% of CF alleles. Of the remaining mutations, 159 occur at a prevalence >0.01% in the CF population. Six main classes of CFTR mutation have been defined, categorized according to effects on CFTR production, intracellular processing, and function ( Fig. 53.1 ).

In class I mutations, a premature termination codon prevents full-length CFTR protein from being produced; these are present in approximately 10% of CF patients worldwide, although they are very much more common in certain populations such as the Ashkenazi Jewish population. Class II mutations (Phe508del being the commonest) result in CFTR being misfolded within the epithelial cell. As a result the protein cannot be transported to its site of action in the cell membrane, instead being prematurely degraded by the ubiquitin-proteasome system. In class III mutations, CFTR is produced and sited within the cell membrane, but there is infrequent opening (“gating”) of the chloride ion channel; these mutations are present in approximately 5% of CF patients worldwide, with the commonest by far being Gly551Asp (G551D). Class IV mutations (e.g., Arg117His) result in reduced ion conductance. Class V are splicing mutations, which result in reduced protein expression, and class VI mutations impact the turnover of CFTR at the cell membrane due to a reduced half-life. Despite these distinctions, it is important to recognize that the functional impact of the mutation may traverse several classes; for example, Phe508del (predominantly considered to be a class II mutation) also impacts the function of any CFTR that does reach the cell membrane (such as abnormal gating and reduced half-life).
Targeting Cystic Fibrosis Transmembrane Conductance Regulator Protein With Small Molecule Drugs
The small molecule drugs that target CFTR dysfunction are commonly referred to according to their mechanism of action. They include ribosomal read-through agents, “potentiators,” and “correctors.” Agents capable of “reading-through” a prematurely placed stop codon aim to produce a full-length functional protein. Potentiators are aptly named as they potentiate CFTR function but rely on appropriate placement within the cell membrane in the first instance. Correctors aim to rectify protein misfolding and thereby facilitate trafficking to the cell membrane.
Therapeutic candidates using one or more of these approaches have been evaluated in clinical trials, and a small number have now progressed through licensing to the clinic. They tend to be administered via the enteral route (possible due to their low molecular weight), which means multiorgan effects may be achieved ( Fig. 53.2 ), and once commenced, the duration of treatment is potentially lifelong.

Read-Through Agents
Stop codons are nucleotide triplets in messenger RNA (mRNA) that serve a key role in signaling the end of protein coding sequences (e.g., UAG, UAA, UGA). Premature stop codons are those that occur within the normal coding sequence due to a mutation. This means that the message to create the protein of interest is incomplete; thus only truncated protein is formed. Molecules that allow the read-through of premature stop codons, thus enabling the production of full-length protein, are being evaluated for several diseases including class I mutation CF.
The aminoglycoside gentamicin was initially shown to possess such capabilities, but concerns over the adverse safety profile of this group of agents (renal toxicity and sensorineural deafness) led to a search for other potential candidates. The one that has been most extensively investigated is the oral small molecule drug ataluren (Translarna, formerly PTC124. PTC Therapeutics; http://www.ptcbio.com/en/pipeline/ataluren-translarna/ ). This was identified by a high-throughput screening process involving more than 800,000 compounds. In CFTR knockout mice expressing human G542X mutant CFTR, ataluren increased CFTR expression at the apical surface of epithelial cells and restored cyclic adenosine monophosphate (cAMP)-stimulated chloride currents to approximately 25% of wild type. Ataluren has been evaluated in both adults and children older than 6 years with at least one CFTR nonsense mutation. Changes toward normal in nasal potential difference (nPD) measurements (a bioelectrical test of CFTR function) were observed in most adults and in approximately half of children in early phase trials. An increased proportion of airway epithelial cells expressing CFTR detected by immunofluorescence was also seen. These phase II trials involved CF patients from Israel (adults) and Europe (children). The results were in contrast to the lack of efficacy seen in a phase II trial of PTC124 in the United States. The phase III randomized controlled trial (RCT) that followed involved 238 patients with a nonsense CFTR mutation. Patients were randomized to receive ataluren in a 3 times daily dosing regimen (10 mg/kg morning and midday; 20 mg/kg evening) or placebo for 48 weeks. Although neither the primary outcome of forced expiratory volume in one second (FEV 1 ) nor the secondary outcome of a reduction in pulmonary exacerbations were met, on post hoc analysis these outcomes were statistically significant for patients who were not receiving concurrent inhaled tobramycin as part of their routine CF management. This has led to a further phase III trial (NCT02139306) specifically for patients not on inhaled aminoglycosides, as it has been suggested that the lack of response in patients receiving aminoglycosides may be due to competition with ataluren at the ribosomal binding site; at the time of writing, the results of this second trial have not been published, but a sponsor’s press release describes it as having failed to achieve its outcomes ( http://ir.ptcbio.com/releasedetail.cfm?releaseid=1015471 ). Drugs such as ataluren targeting premature stop codons have the potential for broader applicability than being limited to a single disease. For example, ataluren has been granted a conditional marketing authorization by the European Medicines Agency, based on the results of a phase IIb study in boys with Duchenne muscular dystrophy in conjunction with an unmet need for disease-modifying therapies in this condition.
Potentiators
The clinical trials of ivacaftor (Kalydeco, VX-770 Vertex Pharmaceuticals; http://www.kalydeco.com/how-kalydeco-works ) undertaken to date have represented an important milestone in the proof of concept for drugs aiming to target the underlying molecular defect in CF. Identified through high-throughput screening, ivacaftor potentiated CFTR in human bronchial epithelial cells from compound heterozygotes with the commonest class III mutation Gly551Asp/Phe508del in vitro. It was then evaluated in patients with at least one copy of this mutation; early-phase studies demonstrated evidence of functional improvement in CFTR with nPD and sweat chloride measurements. Phase III placebo controlled studies followed with impressive results. Efficacy was demonstrated on a regimen of ivacaftor 150 mg twice daily in adults and adolescents (STRIVE), and children aged 6–11 years (ENVISION). In both trials a significant improvement in lung function was seen on active treatment with absolute improvements in FEV 1 of 10.6% and 12.5%, respectively. The improvement in lung function was evident by week two of treatment with ivacaftor and was sustained through 48 weeks. In parallel, there were also significant reductions in sweat chloride concentrations, and improvements in quality of life and weight. The pulmonary exacerbation frequency was significantly reduced in adults receiving ivacaftor.
A key question for any therapy which can potentially affect the natural history of a disease course is whether efficacy can be demonstrated in patients with mild disease (i.e., before irreversible changes within the airway occur). The use of more sensitive outcome measures has helped to move this area forward in patients with normal lung function as assessed by FEV 1 , the historically favored primary outcome. In patients with mild CF lung disease (FEV 1 ≥ 90% predicted) aged 6 years and older with the Gly551Asp mutation, a clinical trial in patients demonstrated significant improvements in lung clearance index (LCI). LCI has also been used to demonstrate efficacy in those with advanced disease (FEV 1 < 40% predicted).
Following the clinical trial that demonstrated that improvement in LCI was observed in those with preexisting normal FEV 1 , it became important to consider if the CFTR modulator ivacaftor could be given in the early years of life because CFTR modulation therapy might have the greatest potential to prevent organ damage at that stage. Outcome measures for lung efficacy are a challenge in this population, and therefore a clinical trial in preschool children was primarily undertaken using outcomes for safety and pharmacodynamics (sweat chloride). This open label study (KIWI) involved ivacaftor (50 mg or 75 mg twice daily) in preschool children with any CFTR gating mutation and found it was well-tolerated and safe, although an elevation in liver function tests (transaminases) warrants close monitoring in the clinic. A notable finding in the KIWI study was the effects of ivacaftor on growth and pancreatic function. Increases in fecal elastase and decreases in serum immunoreactive trypsinogen on treatment were suggestive of partial improvement of exocrine pancreatic function. As a systemically administered therapy, small molecule CFTR modulators have the potential to affect nonpulmonary disease manifestations. At the time of writing, efficacy outcomes (LCI and computed tomography [CT] scans) are being further explored in a trial in 3–5 year olds (NCT02742519) and a trial in infants less than 24 months of age (NCT02725567) is assessing safety, pharmacokinetic, and pharmacodynamic outcomes.
Although initially restricted to patients with the commonest mutation in the class III group, in vitro evidence of efficacy in other CFTR gating and residual function mutations led to the investigation of ivacaftor in non-Gly551Asp gating mutations and the Arg117His (R117H) class IV mutation. Efficacy was successfully demonstrated in non-Gly551Asp gating mutations in the KONNECTION study, although for patients with Arg117His (KONDUCT), efficacy was limited to patients older than 18 year. The authors suggested that ivacaftor may therefore be beneficial for patients with the Arg117His mutation who have established CF disease. The lack of effect in children may reflect the inclusion of this mutation in many CF newborn screen testing algorithms and its known phenotypic variability with milder variants being detected and thus skewing the population included in such clinical trials.
Key to the success of CFTR modulation is in the ability to alter the disease trajectory in CF. There is already some evidence that ivacaftor can achieve this, and as the proportion of patients for whom this drug becomes part of routine CF care increases (in terms of mutation class and younger age), long-term pharmacovigilance will be eagerly awaited. The open label extension study of patients enrolled in the STRIVE and ENVISION trials (PERSIST; NCT01117012) reported absolute change in FEV 1 at week 96 (i.e., total duration of ivacaftor 144 weeks) of 9.4% and 10.3% in adults/adolescents and children, respectively, in those receiving ivacaftor in the original phase III trials. A longitudinal study of ivacaftor in the postapproval setting reported improvements in several clinically relevant markers, including lung function, body mass index, mucociliary clearance, hospitalization rate, and Pseudomonas aeruginosa burden.
The successes of the ivacaftor clinical trials have sparked heightened interest in the search for more efficacious CFTR potentiators. Several companies are conducting early-phase clinical trials at the time of writing. This includes Galapagos NV with GLPG1837 in patients with the Gly551Asp mutation (NCT02707562) and the S1251N gating mutation (NCT02690519), as well as two other molecules. Novartis Pharmaceuticals have undertaken a trial of their CFTR potentiator, QBW251, in patients with CF (NCT02190604).
Concert Pharmaceuticals, Inc. have conducted a phase I trial of deuterated ivacaftor. Their strategy is based on selectively replacing hydrogen atoms with deuterium (a heavy isotope of hydrogen), with the aim of stabilizing carbon bonds within a compound. Their deuterium-modified ivacaftor (CTP-656) had a superior pharmacokinetic profile to standard ivacaftor (Kalydeco) in healthy volunteers.
As a single agent therapy, the patients benefiting from potentiator therapies are limited to a minority of the CF population with class III and IV mutations. In patients with the class II mutation Phe508del, the in vitro data suggesting small potentiating effects of ivacaftor in bronchial epithelial cells from Phe508del homozygotes did not translate into clinically detectable benefit. Strategies for tackling Phe508del are discussed in the next section.
Correctors
Drugs that target class II mutations in CF (e.g., Phe508del) in which a misfolding of CFTR leads to failure of its trafficking to the apical cell membrane are referred to as correctors. Because this class of mutations represents the greatest proportion of mutations within the CF population, an effective CFTR modulator in this group would have a profound impact. The furthest developed drug in this class is the oral small molecule drug lumacaftor (VX-809, Vertex Pharmaceuticals). In vitro studies demonstrated that in cell culture of bronchial epithelial cells homozygous for the Phe508del mutation, lumacaftor increased chloride secretion to approximately 14% of wild-type CFTR, through its mechanism of action on the processing of CFTR in the endoplasmic reticulum. Functional evidence of efficacy as assessed by sweat chloride was demonstrated in adult CF patients homozygous for the Phe508del mutation, although these were small in magnitude and were not associated with significant clinical changes. The single drug approach may be limited by the complexity required to restore CFTR function in patients with Phe508del.
There are several other drugs being investigated that may have a novel mechanism of action in modulating CFTR. The nitric oxide pathway may be a possible CFTR corrector strategy (either via the direct or indirect pathways). Examples in early-phase clinical trials include N91115 (Nivalis Therapeutics, Inc.), which the company describes as a first-in-class CFTR “stabilizer.” N91115 is an S-nitrosoglutathione reductase inhibitor. The aim is to stabilize CFTR both from within the cell and on the apical surface of the airway epithelial cells. Following a phase Ib clinical trial investigating N91115 monotherapy in adults homozygous for the phe508del mutation (NCT02227888), a phase II trial of safety and efficacy in patients receiving lumacaftor/ivacaftor (i.e., as a triple approach) (NCT02589236) has recently completed. Most recently, Nivalis Therapeutics have commenced a further phase II trial of N91115 in adult patients heterozygous for F508del-CFTR and a gating mutation receiving ivacaftor treatment (NCT02724527); results have not been published in a peer-reviewed journal, but at the time of writing, the company’s press release indicates a safe but ineffective drug ( http://ir.nivalis.com/press-releases/detail/60 ). Also involving the nitric oxide pathway, the drug riociguat (BAY63-2521, Adempas, Bayer), with existing approval for the treatment of chronic thromboembolic pulmonary hypertension, has been found to have CFTR corrector function and is currently being investigated in a phase II study (NCT02170025) assessing safety, tolerability, and efficacy of the orally administered drug in adult patients homozygous for phe508del. Phosphodiesterase inhibitors including sildenafil, vardenafil, and tadalafil may also prove useful in CF caused by class II mutations. Using an inhaled preparation, these drugs have been shown to induce changes toward normality in nPD measurements in p.Phe508del mice, but it has been suggested that high doses may preclude this as a therapeutic approach in humans.
Combination Therapy
Given the lack of clinical efficacy of lumacaftor alone in patients homozygous for Phe508del, the addition of a potentiator to maximize the function of any CFTR reaching the cell surface is an attractive option. Most research to date has been with the combination of ivacaftor and lumacaftor, the potentiator and corrector described in previous sections. In vitro studies showed that addition of ivacaftor to lumacaftor-exposed Phe508del homozygous cells further increased chloride transport to 25% of wild type, in keeping with prior observations of defective gating in addition to defective processing of Phe508del CFTR.
Subsequently, two large phase III RCTs (TRAFFIC and TRANSPORT) studied patients aged 12 years and older homozygous for Phe508del. They showed relatively small but significant improvement in lung function of the magnitude of 3%–4% predicted FEV 1 , on a regimen of lumacaftor (either 400 mg twice daily or 600 mg daily) and ivacaftor 250 mg twice daily. More impressive was the reduction in the rate of pulmonary exacerbations (30%–39% reduction). Although the lumacaftor-ivacaftor was generally well tolerated, trial discontinuation was higher in the active arm, and the adverse events occurring more frequently in the active treatment group tended to be respiratory in nature (e.g., bronchospasm and dyspnea). The combination therapy lumacaftor-ivacaftor has now been licensed by the US Food and Drug Administration (FDA) (lumacaftor/ivacaftor, Orkambi, Vertex Pharmaceuticals, Inc.), and is available clinically in several countries, although reimbursement is not approved worldwide.
The magnitude of the improvement in lung function with ivacaftor and lumacaftor in Phe508del homozygotes was lower than that observed with ivacaftor in class III mutations. In vitro assays on Phe508del human bronchial epithelial cells treated with lumacaftor/ivacaftor have shown that ivacaftor may be destabilizing CFTR that has been transported to the cell membrane by lumacaftor, and this may help to explain the relatively limited efficacy observed with this combination of drugs in vivo in clinical trials. This destabilization has been shown to cause a reduction of CFTR on the apical cell membrane and a reduced half-life resulting from increased turnover.
As described for CFTR modulation with ivacaftor, one of the key aims is to be able to instigate therapy before irreversible changes resulting from CF lung disease occur. Therefore the tolerability, safety profile, and efficacy in children and those with mild disease are important. A phase III trial in younger children (6–11 years) to assess the impact of Orkambi in earlier disease (NCT02514473), using LCI as the primary outcome, is in progress.
Lumacaftor is the only CFTR corrector to have progressed to licensing (as the combination formulation); however, other candidates are being investigated. The corrector VX-661 in combination with ivacaftor (Vertex Pharmaceutical, Inc.) showed promising results in early-phase trials and is currently undergoing evaluation in a number of large, phase III clinical trials in Phe508del homozygotes (NCT02347657) and Phe508del heterozygotes with a second mutation (1) which has been shown to be responsive to ivacaftor (NCT02412111), (2) with residual function (NCT02392234), and (3) likely unresponsive to VX661 or ivacaftor (NCT02516410). Following participation in these trials, patients may elect to roll over into a longer, open-label trial (NCT02565914). Interestingly the compound VX-661 does not appear to destabilize ivacaftor in the same way reported for the lumacaftor/ivacaftor combination and thus may be suited to the combination therapy approach.
Other companies are also developing drugs for a triple therapy approach with existing combinations of CFTR modulation. Proteostasis Therapeutics, Inc. have developed PTI-428 as a CFTR amplifier, which aims to increase an immature form of CFTR protein to provide additional substrate for other CFTR modulators. A safety and pharmacokinetic study involving adult patients with CF is being undertaken at the time of writing (NCT02718495). Flatley Discovery Labs have a combination of modulators from which encouraging in vitro data have recently been reported.
Autophagy, a major mechanism used by cells in protein turnover within the cytoplasm, has been shown to be abnormal in CF cells with the F508del mutation related to depletion of the essential autophagy-related protein Beclin 1 (BECN1). A phase II trial of two proteostasis regulators (cysteamine and epigallocatechin gallate [EGCG]) for the autophagy-dependent rescue of CFTR reported improvements in sweat chloride, inflammatory markers, and FEV 1 in patients with Phe508del or other class II homozygotes or compound heterozygotes. The authors reported that patients in this trial whose primary nasal brushed cells did not respond to cysteamine plus EGCG in vitro also exhibited deficient therapeutic responses in vivo.
Other Noncystic Fibrosis Transmembrane Conductance Regulator–Based Approaches
Small molecule drugs targeting the epithelial sodium channel ENaC are emerging as an active field of research. For example, Vertex Pharmaceuticals, Inc. have, at the time of writing, commenced a phase II clinical trial of a triple therapy approach with VX-371 (P-1037) in combination with Orkambi (NCT02709109) in patients aged 12 years and older homozygous for the F508del CFTR mutation.
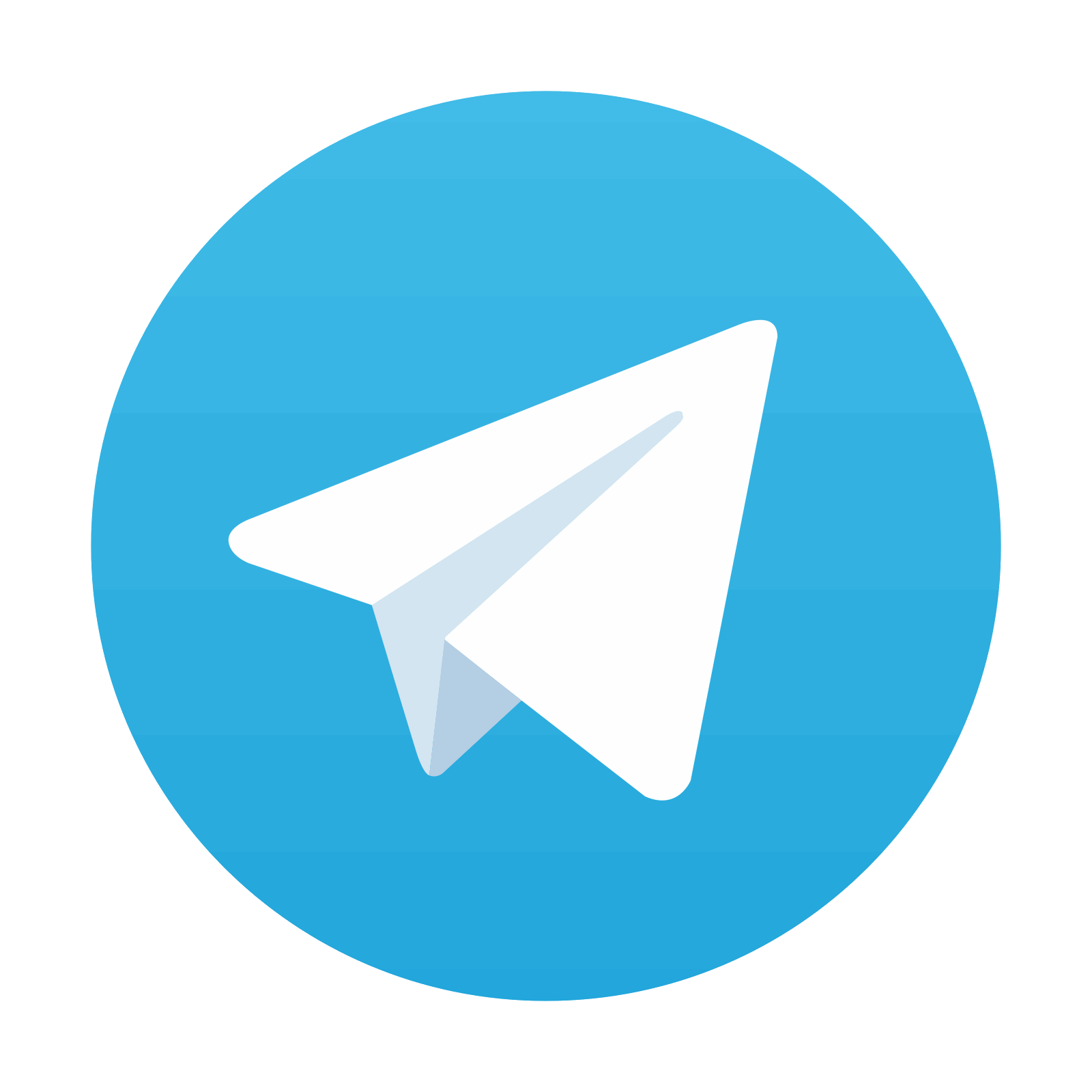
Stay updated, free articles. Join our Telegram channel

Full access? Get Clinical Tree
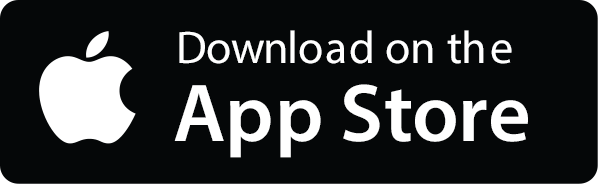
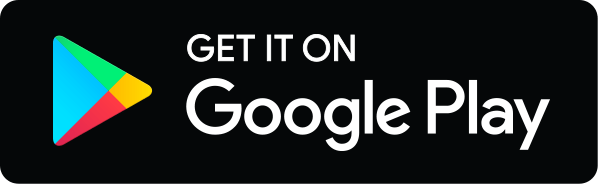