Histologic observations have taught us much regarding the pathophysiology of the acute coronary syndromes. Pathoanatomic examination of atheromas that provoke fatal thrombosis reveals much about the mechanisms of this extreme form of an acute coronary syndrome. Although previously controversial, the role of thrombosis in producing the acute coronary syndromes has now gained wide acceptance.
Four distinct microanatomic mechanisms can precipitate the acute coronary syndromes. , Plaque rupture, the most common and perhaps the best understood, causes two thirds to three quarters of fatal acute myocardial infarctions ( Fig. 7-1A ). Superficial erosion without a frank rupture in the plaque’s fibrous cap underlies some 20% of fatal acute coronary thrombi (see Fig. 7-1B), and erosions around calcium nodules account for a few acute coronary thromboses. Another mechanism of rapid plaque expansion, intraplaque hemorrhage, may also play a role in precipitating some cases of acute coronary syndromes.

Beyond these structural microanatomic substrates, which usually involve a disruption of the plaque, functional changes can also influence the thrombotic potential and stability of clots. A balance between procoagulant and anticoagulant factors prevails at any particular moment in the vascular compartment ( Fig. 7-2 ). Similarly, profibrinolytic and antifibrinolytic factors may regulate the stability of clots. In addition to fluctuations in the blood compartment in the determinants of thrombosis, local regulation at the level of the arterial wall in these regulatory pathways may determine the consequences of any given plaque disruption.

Recognition has increased that inflammation is a fundamental and common theme in the structural and functional pathways to thrombosis. Furthermore, molecules and cells involved in both the innate and adaptive arms of the immune response may participate in many of the processes that precipitate the acute coronary syndromes. These new insights aid in understanding the pathophysiology of the acute coronary syndromes; they have prognostic and therapeutic implications as well. This chapter describes recent advances in the pathophysiology of the acute coronary syndromes, emphasizing the practical clinical import of these new concepts.
Mechanisms of Plaque Disruption
Plaque Rupture
Many human coronary atheromas contain a lipid-rich central core. In addition to extracellular lipid debris, the lipid core contains foamy macrophages, many of which have surfaces studded with the potent procoagulant, tissue factor. A collagenous fibrous cap overlies the typical lipid core and serves as a barrier between the blood compartment, which contains proteins of the coagulation cascade, and thrombogenic material in the lipid core.
In plaque rupture, a thinned and friable fibrous cap fractures. The contact between blood and tissue factor in the lipid core unleashes the clotting cascade. Collagen uncovered during plaque disruption can promote platelet adherence and activation. Resistance of the fibrous cap to rupture depends largely on the integrity of the interstitial collagen in the extracellular matrix. Collagen fibrils lend biomechanical strength to the fibrous cap. In general, interstitial collagen has considerable stability and turns over slowly, if at all. Moreover, until recently, most regarded the atheroma as a metabolically inactive graveyard for excess cholesterol stuck in the artery wall. It is now understood that the atheroma teems with living cells whose functions and exchanged messages may dictate the clinical consequences of the atheromatous lesion. Inflammation has also emerged as a unifying concept in the pathogenesis of atherosclerosis and its complications.
Therefore, in the early 1990s, I hypothesized that the collagenous skeleton of the plaque depends on a dynamic balance between ongoing collagen synthesis and degradation. The arterial smooth muscle cell synthesizes the great majority of the collagen in this structure, and expression of interstitial collagen genes by smooth muscle cells depends on the inflammatory milieu. Interferon-γ (IFN-γ), a cytokine produced in plaques, almost halts procollagen gene expression by cultured smooth muscle cells. Atherosclerotic plaques contain IFN-γ. When activated, the T lymphocyte produces high levels of IFN-γ.
Other cells found in the atheroma can also elaborate IFN-γ under certain circumstances. For example, smooth muscle cells and macrophages exposed to a combination of cytokines found in the plaque (interleukin [IL]-12 and IL-18) can secrete IFN-γ. These observations led to the hypothesis that T cells may locally inhibit collagen synthesis in regions of the atherosclerotic plaque. T lymphocytes localize in atherosclerotic plaques, particularly in the shoulder region, where tears in the plaque often cause rupture. Indeed, computational analysis of the biomechanical forces impinging on a plaque reveal maxima in circumferential stresses at the shoulders of plaques. Rekhter and colleagues have painstakingly enumerated cell types in various regions of the plaque and simultaneously assessed rates of interstitial collagen gene expression by in situ hybridization. They found a highly significant coincidence of T cells within regions of lower levels of interstitial collagen gene expression. Van der Wal and associates have carefully enumerated cell types at sites of actual disruptions of human coronary plaques that caused fatal thrombosis, and have found abundant T cells in the vicinity of the site of plaque disruption.
Taken together, these results indicate that IFN-γ derived from T cells in the shoulder region of inflamed plaques can impede the synthesis of new collagen by smooth muscle cells. Inhibition of interstitial collagen synthesis would interfere with the ability of the smooth muscle cell to repair and maintain the all-important fibrous cap. Experiments in mice rendered scorbutic by genetic engineering have shown that vitamin C (ascorbic acid) deficiency impairs the collagenous structure of the plaque. Because vitamin C promotes the cross-linking of collagen chains, this finding supports a role for collagen protein production in determining the structure of the plaque’s fibrous cap.
Intact collagen fibrils resist degradation by all but a few enzymes, known as interstitial collagenases. Humans produce three interstitial collagenases. These three enzymes belong to a broader family of proteinases that are dependent on zinc atoms and are thus known as matrix metalloproteinases (MMPs). In 1991, Henney and coworkers detected messenger RNA encoding one MMP-stromelysin, or MMP-3, in human atherosclerotic plaques. Importantly, human atheromata also overexpress interstitial collagenases. , The interstitial collagenases (MMP-1, -8, and -13) localize with macrophage foam cells and smooth muscle–derived foam cells in regions of human atherosclerotic plaques at sites particularly prone to rupture, as shown by biomechanical and pathologic studies. , Observations made on experimental atheroma in rabbits have shown the importance of the macrophage as a source of interstitial collagenase. , MMP-8 degrades type I collagen more efficiently than MMP-1 or MMP-13. Type I collagen accounts for the bulk of collagen in the atheroma. Therefore, MMP-8 has a substrate specificity that may render it one of the most important proteinases in degrading collagen in the atheroma. In other studies, Sukhova and colleagues and Liu and associates established overexpression of nonmetalloenzymes, including cathepsins S, K, and L, in the atherosclerotic plaque. Although chiefly implicated in elastolysis, cathepsin S may exhibit interstitial collagenase activity as well.
The overexpression of messenger RNA and protein corresponding to interstitial collagenases does not necessarily translate into increased enzyme activity. Proteinase cascades important in biologic control undergo regulation at several levels. MMPs, first synthesized as inactive precursors, require activation to attain their enzymatic function ( Fig. 7-3 ). Moreover, widely distributed endogenous inhibitors can limit the action of any activated form of the MMPs. Four members of the tissue inhibitor of MMP (TIMP) family exist, and vascular lesions can contain all four. Also, human arteries express tissue factor pathway inhibitor 2 (TIMP-2), which also inhibits collagenases. This homologue of TIMP-2 actually inhibits tissue factor poorly but blocks the action of collagenases as potently as TIMPs. Even if active molecules of the interstitial collagenase were to exist in the plaque, they could not digest collagen unless they overpowered these endogenous inhibitors. Unfortunately, none of the available immunologic reagents can distinguish the inactive zymogen form of the interstitial collagenases from their active counterparts.

Thus, the mere presence of immunoreactive interstitial collagenases in plaques does not imply that they actively participate in collagenolysis in situ. To prove this point, our laboratory assessed collagen degradation directly in situ using an antibody that selectively recognizes collagen that has undergone cleavage by interstitial collagenase. This study established that active collagen breakdown occurred in advanced human atheromas and, further, colocalized MMP-1 and MMP-13 within regions of collagenolysis in situ. These observations all suggest that enhanced breakdown of interstitial collagen caused by the action of MMPs may contribute to thinning and weakening of the fibrous cap and, hence, to the pathogenesis of plaque rupture. Therapies that limit the acute coronary syndromes may act in part by stabilizing plaques by interfering with collagen breakdown and increasing collagen synthesis. In rabbits with dietary or endogenous hyperlipidemia, lowering of cholesterol by dietary or pharmacologic means can reduce the expression of interstitial collagenase and promote collagen accumulation within plaques.
Recent studies in mice have provided strong support for the concept that proteinases that can degrade the extracellular matrix can contribute to the collagen composition of plaques and their tendency to cause thrombosis. Mice that bear a mutation that renders their interstitial collagen resistant to MMP collagenases in the context of genetically determined susceptibility to atherosclerosis have increased collagen accumulation in the atherosclerotic intima. Atherosclerosis-susceptible mice altered genetically to lack a major murine interstitial collagenase, MMP-13, similarly show augmented collagen content in their atherosclerotic plaques. Mice manipulated to lack another enzyme known as MMP-14 in cells derived from bone marrow also accumulate lesional collagen. MMP-14 appears to act by proteolytically processing the zymogen form of the interstitial collagenase MMP-13 to its active form, rather than by attacking collagen directly.
Proteinases not notable for their interstitial collagenase activity may also promote thrombosis in experimental atherosclerotic plaques in mice. Atherosclerosis-susceptible mice infected with a virus that directs the expression of an activated form of the gelatinase MMP-9 have shown evidence of plaque disruption. Mice that bear compound mutations in apolipoprotein E and Niemann-Pick C protein demonstrate thrombotic complications of atherosclerotic plaques as well. The proteinase cathepsin K may contribute to this thrombotic phenotype.
Recent evidence also provides insight into the links among adaptive immunity, inflammation, and thrombotic complications of atheroma. Mice genetically altered to augment T cell activity (because of T-cell–directed expression of transforming growth factor beta [TGF-β] receptor 2) show highly inflamed plaques, reduced collagen integrity, and evidence of thrombosis. , These mice have decreased levels of lysyl oxidase, an enzyme important in collagen cross linking. They also demonstrate augmented expression of two matrix-degrading proteinases, MMP-13 and cathepsin S.
Given the principal role of the smooth muscle cell in collagen synthesis in the artery wall, the level of collagen in a plaque may depend on the number of smooth muscle cells. The regulation of collagen gene expression in smooth muscle cells varies with the prevailing mediator milieu. For example, platelet-derived growth factor and TGF-β, protein mediators released from platelets at sites of thrombosis but also produced by many other cells, augment interstitial collagen gene expression in human smooth muscle cells. Thus smooth muscle cells, when present, promote plaque stability. Histopathologic observations have shown an inverse correlation between smooth muscle cells and plaque ruptures—plaques that have ruptured have few smooth muscle cells. Hence, I proposed that the smooth muscle cell was the “guardian of the integrity of the plaque’s fibrous cap.” It is now recognized that smooth muscle cells in the plaque can die. Cell death may cause smooth muscle cells in atherosclerotic plaques to drop out, eventually making a plaque more vulnerable.
Smooth muscle cells within the plaque can die by several mechanisms, including apoptosis, or programmed cell death. Smooth muscle cells exposed to proinflammatory mediators found in the atheroma can die by apoptosis. Notably, a combination of proinflammatory cytokines can promote smooth muscle cell apoptosis. The members of the surface-based cell death signaling dyad, Fas/Fas ligand, also operate in plaques. These observations strengthen the link between inflammation and impaired stability of the fibrous cap in human atheroma.
Superficial Erosion
Another form of physical disruption of the atherosclerotic plaque contributes to many coronary thromboses. Superficial erosion, rather than a frank fissure, in the fibrous cap accounts for approximately one quarter to one third of fatal coronary thrombi. Superficial erosion appears more commonly than fibrous cap fracture in younger persons, women, and those with hypertriglyceridemia or diabetes. The molecular pathways underlying superficial erosion have received less attention than those regulating the friability of the fibrous cap. Not all experts agree on the degree to which inflammation participates in superficial erosion. Virmani and coworkers have emphasized the bland, noninflammatory nature of sites of superficial erosion in human coronary arteries. The Amsterdam group has consistently found evidence for the coexistence of T cells and macrophages at sites of plaque disruption caused by superficial erosion and rupture of the cap.
Although the underlying mechanisms remain speculative, some mechanistic commonality may exist between these two most common forms of plaque disruption. In superficial erosion, endothelial cells may detach because the connections that tether them to the underlying basement membrane loosen. Type IV collagen, the major type of collagen in the subendothelial basement membrane, may also undergo proteolytic degradation. In contrast with the fibrillar collagens that make up the plaque’s fibrous cap, type IV collagen in the basement membrane does not form fibrils. Type IV collagen also can undergo catabolism by MMPs. However, instead of the interstitial collagenases, this nonfibrillar type of collagen undergoes degradation by MMP-2, a form of gelatinase. Like the other MMPs, MMP-2 requires activation from a zymogen precursor to cleave its substrate, type IV collagen. The cell surface–bound proteinase known as membrane-type MMP, or MT-MMP (MMP-14), participates in the activation of MMP-2. Proinflammatory cytokines and oxidized low-density lipoprotein can augment the expression by endothelial cells of the MT-1–MMP/MMP-14 that can activate MMP-2. Moreover, reactive oxygen species released from vascular cells and infiltrating inflammatory cells in inflamed plaques can activate MMPs directly. Thus, active degradation of the subendothelial basement membrane caused by the proteinases regulated by inflammation may promote endothelial desquamation. Such a scenario could provide one mechanism for superficial erosion as a source of coronary thrombi.
In addition, endothelial cells may detach because they die. As in the case of smooth muscle cells, endothelial cells can undergo death by apoptosis. Some inflammatory mediators encountered by endothelial cells may promote resistance to apoptosis, partially by inducing the antiapoptotic pathway involving nuclear factor kappa B (NF-κB). However, other proinflammatory stimuli may sensitize endothelial cells to programmed cell death. For example, hypochlorous acid, the product of the enzyme myeloperoxidase, can provoke endothelial cell apoptosis. Macrophages in the human atheroma contain myeloperoxidase, and substantial evidence supports local generation of hypochlorous acid within atherosclerotic plaques. Thus, increased endothelial cell death, regulated in part by inflammatory mediators, may contribute to the pathogenesis of superficial erosion.
Erosion Caused by Calcium Nodules
Erosion caused by mineral collections, niduses of calcification, provides another route to coronary thrombosis. This mechanism accounts for only 4% to 7% of fatal coronary thromboses. Calcification in atherosclerotic plaques depends on tightly regulated biochemical processes. , Proteins such as osteopontin and bone morphogenetic proteins may regulate the accretion of calcium mineral in atheroma. Just as the level of collagen in the plaque depends on the balance of synthesis and degradation, the level of calcium mineral depends on a similar balance. Bone morphogenetic protein likely enhances bony metaplasia in atherosclerotic plaques. On the other hand, macrophages may break down deposits of calcium. Indeed, macrophages in human atherosclerotic plaques can express some of the enzymes implicated in bone resorption by osteoclasts, including cathepsins S and K. Observations in mice with mutations that affect the number and function of macrophages have illustrated in vivo the importance of the catabolism of calcified tissue in atheroma. Specifically, mice lacking macrophage colony-stimulating factor, and thus unable to produce mature macrophages, show increased levels of calcium in experimentally produced atheroma. These observations show how the degree of calcification in atheroma depends in part on inflammatory signaling within the lesion.
Recent computational data have suggested that microscopic deposits of calcium mineral, well below the threshold of in vivo imaging, can augment circumferential stress and thus promote plaque rupture. , These findings have clinical significance because individuals with low levels of conary arterial calcium detectable by various imaging modalities might nonetheless have mechanically jeopardized plaques because of these microscopic foci of calcification. Specks of calcium as small as 10 µm in diameter can adversely affect the biomechanical properties of plaques. These microscopic calcified nodules might represent the remains of apoptotic cells within the atheroma.
Plaque Hemorrhage
Intraplaque hemorrhage with rapid lesion expansion may also precipitate the acute coronary syndromes. Many earlier pathologic studies viewed intraplaque hemorrhage as a major pathway to coronary thrombosis. However, later pathologic studies involving painstaking serial sectioning identified ruptures of the plaque’s fibrous cap as the primary lesion, with blood collection within the atheroma often secondary.
The amount of microvessels in the plaque may influence its biology in several ways. In the same way that growth of tumors depends on the formation of new blood vessels, the progression of atheroma may depend in part on neovascularization. Experiments with inhibitors of angiogenesis have shown attenuated lesion formation in mice. Atherosclerotic lesions express a number of angiogenic factors. For example, acidic fibroblast growth factor correlates with microvessel formation in plaques and colocalizes with inflammatory cells. Vascular endothelial growth factor, basic fibroblast growth factor, and other angiogenic proteins may promote plaque neovascularization.
Although intraplaque hemorrhage probably causes very few acute coronary syndromes by itself, the phenomenon may yet have important consequences for plaque evolution. Thrombosis in situ caused by ruptured friable neovessels in the plaque would lead to local thrombin generation. Thrombin potently stimulates smooth muscle cell migration, replication, and collagen synthesis. In this manner, intraplaque hemorrhage might promote the evolution of fibrous atherosclerotic plaques. In addition, iron derived from hemosiderin in extravasated blood within the plaque may promote oxidative processes. Notably, the production of reactive oxygen species by the Fenton reaction requires transition metal cations, such as iron. The presence of microvessels within plaques and the potential role of intraplaque hemorrhage in lesion expansion and growth sound a cautionary note in the context of angiogenic therapy for myocardial ischemia. Administration of angiogenic growth factors in the regions of atherosclerotic plaques might actually enhance plaque neovascularization and favor intraplaque hemorrhage, with its attendant adverse consequences for plaque formation. If angiogenic therapy has a similar effect on the atheroma, plaque growth and instability could result from increased nourishment of ischemic myocardium.
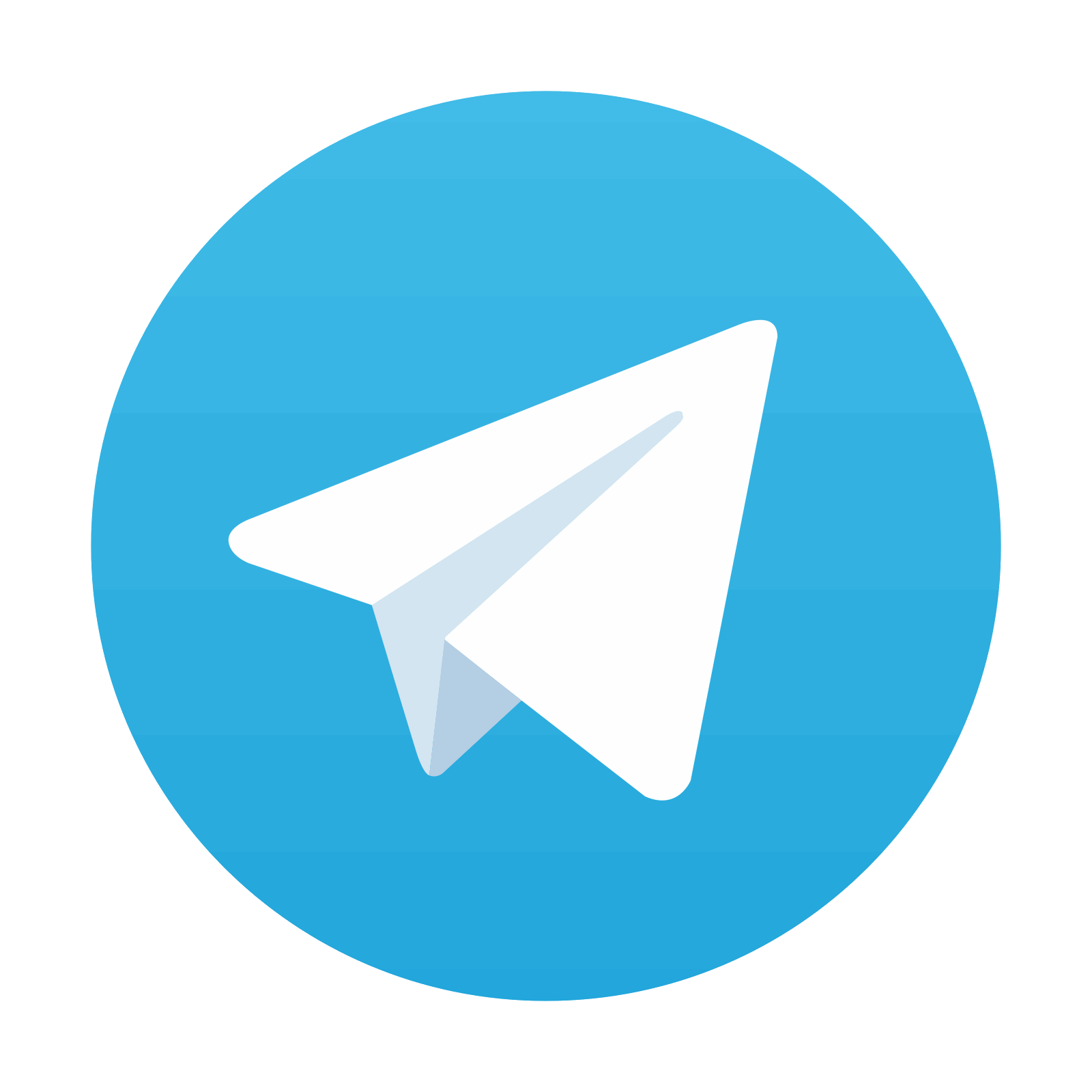
Stay updated, free articles. Join our Telegram channel

Full access? Get Clinical Tree
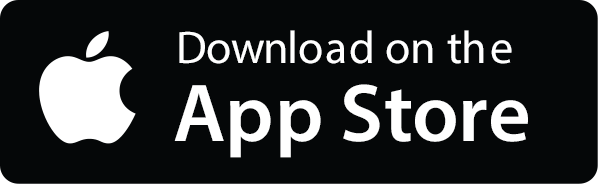
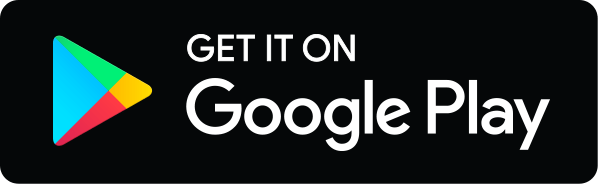