Accreditation Statement:
The American Society of Echocardiography is accredited by the Accreditation Council for Continuing Medical Education to provide continuing medical education for physicians.
The American Society of Echocardiography designates this educational activity for a maximum of 1 AMA PRA Category 1 Credits ™. Physicians should only claim credit commensurate with the extent of their participation in the activity.
ARDMS and CCI recognize ASE’s certificates and have agreed to honor the credit hours toward their registry requirements for sonographers.
The American Society of Echocardiography is committed to ensuring that its educational mission and all sponsored educational programs are not influenced by the special interests of any corporation or individual, and its mandate is to retain only those authors whose financial interests can be effectively resolved to maintain the goals and educational integrity of the activity. While a monetary or professional affiliation with a corporation does not necessarily influence an author’s presentation, the Essential Areas and policies of the ACCME require that any relationships that could possibly conflict with the educational value of the activity be resolved prior to publication and disclosed to the audience. Disclosures of faculty and commercial support relationships, if any, have been indicated.
Target Audience:
This activity is designed for all cardiovascular physicians and cardiac sonographers with a primary interest and knowledge base in the field of echocardiography: in addition, residents, researchers, clinicians, intensivists, and other medical professionals with a specific interest in cardiac ultrasound will find this activity beneficial.
Target Audience:
This activity is designed for all cardiovascular physicians and cardiac sonographers with a primary interest and knowledge base in the field of echocardiography: in addition, residents, researchers, clinicians, intensivists, and other medical professionals with a specific interest in cardiac ultrasound will find this activity beneficial.
Objectives:
Upon completing the reading of this article, the participants will better be able to:
- 1.
Name the basic principles of ultrasound molecular imaging.
- 2.
Describe the physical factors that may influence adhesion of ultrasound microbubbles.
- 3.
Identify the most pertinent clinical applications where imaging with ultrasound could have a major impact in diagnosis and patient care.
- 4.
Recognize the possible targets of ultrasound molecular imaging in the following clinical settings: acute atherothrombotic disease, atherogenesis, high-risk markers of atherothrombosis, neovascularization.
- 5.
List areas of future research for ultrasound molecular imaging.
The Evolution of Imaging
Most would agree that the modern age of using imaging technology to visualize internal anatomy began with Wilhelm Röntgen’s accidental observation in 1895 that a phosphorous screen could be activated when placed in the vicinity of a Crook’s tube, the first generation of cold cathode tubes (electrodes placed in a glass vacuum tube). His discovery that the emitted “particles” could pass through cardboard were soon followed by the finding that these particle were impeded by the more “dense” internal features of the body, leading to the use of x-ray imaging to examine the skeleton ( Figure 1 ). It should be duly noted that the primary malady apparent in the left panel of Figure 1 is matrimony, evidenced by the wedding ring on the hand of Röntgen’s wife, who succumbed to marital trust and participated as a test subject before the potentially harmful effects of radiation exposure were recognized. In the ensuing 15 years, there has been a proliferation of imaging methods that are capable of probing deeper into human pathology. We are reminded almost every year at the Edler Lecture of the American Society of Echocardiography Scientific Sessions about the pioneering work of those who have applied ultrasound engineering to view internal structures. Over the past 4 decades, improvements in both ultrasound imaging technology and our understanding of the pathophysiology revealed by ultrasound have greatly expanded the diagnostic role for echocardiography.
A relatively recent development for all medical imaging modalities, including ultrasound, has been the creation of noninvasive methods to assess biologic processes at the molecular level with targeted or “smart” imaging probes. Rather than providing a comprehensive review of all the progress that has been made in contrast-enhanced ultrasound molecular imaging, in this review article, I instead focus on (1) the basic principles of ultrasound molecular imaging and (2) the most pertinent clinical applications in which imaging vascular phenotype with ultrasound in particular could have a major impact in diagnosis and patient care.
Molecular Imaging Technology: Some Basic Considerations
In a broad sense, “molecular imaging” describes an array of techniques that have been developed to evaluate protein expression, metabolic status, intracellular trafficking, gene transcription, and enzyme activity in cells, tissues, or whole biologic systems. Approaches include optical techniques (microscopy, bioluminescence, fluorescence) as well as traditional noninvasive medical imaging techniques such as magnetic resonance imaging, radionuclide imaging, and ultrasound. When considering the different medical imaging techniques that can be used in humans, there are important differentiating features in terms of (1) detector performance, such as sensitivity and spatial and temporal resolution; (2) properties of the imaging probes; and (3) practical issues, such as expense, availability, and the speed of acquisition.
Molecular imaging with contrast ultrasound relies on the selective targeting and retention of microbubbles or acoustically active particles at sites of disease. Targeting has been accomplished by one of two general approaches. The least specific approach has been to take advantage of the innate ability of conventional shell components of microbubbles (eg, albumin, lipids) to bind at disease sites. This attribute can sometimes be amplified by mild chemical modification of the shell components. For example, the addition of phosphatidylserine (or other lipids with negative charge at physiologic pH) into the shells of lipid microbubbles has been used to amplify complement-mediated attachment to activated leukocytes. This property provides a mechanism to detect severe acute inflammatory processes that occur during myocardial infarction, chronic ischemic left ventricular dysfunction, and transplant rejection ( Figure 2 ).
A more specific targeting approach has been to attach ligands (antibodies, small peptides, or glycoproteins) that recognize specific disease-related molecules to the surface of ultrasound contrast agents. This surface conjugation is almost always performed using a bifunctional molecule that has a shell anchor component and a molecular spacer component that projects the ligand away from the shell surface, which is analogous to placing a lure at the end of a fishing line ( Figure 3 ). The resulting surface density of targeting ligands generally ranges from several hundred to several thousand per square micrometer of shell surface area.
The firm attachment of a multivalent particle contrast agent (ie, a particle bearing multiple binding moieties) to a disease target can be thought of as a form of cellular mimicry. In other words, ligand-directed contrast agents are often designed to emulate circulating platelets or leukocytes, which possess long adhesion molecules on their surface that mediate their attachment to disease sites. Accordingly, the attachment of ultrasound contrast agents is influenced by many of the same factors that govern cellular adhesion to disease sites ( Figure 4 ). Important factors include ligand and target molecule density, bond affinity, and shear forces that oppose firm attachment. The intravascular confinement of most ultrasound contrast agents is also an important consideration. With few exceptions, ultrasound molecular imaging probes have been used to identify events that occur within the vascular compartment. Although this constraint limits the potential targets, there are still many important molecular markers of ischemic injury, inflammation, angiogenesis, and thrombosis that are accessible to ultrasound contrast agents.
A common thread in this review is that adhesion molecules are a useful target for imaging inflammatory responses that occur during ischemia, reperfusion injury, atherosclerosis, and angiogenesis. The cellular inflammatory response, which involves leukocyte infiltration of tissue, relies on a carefully coordinated set of events ( Figure 5 ). First, leukocytes are recruited to the vessel wall and roll along the endothelium. This process is largely mediated by interaction between selectins (lectin-like adhesion molecules) on endothelial cells or leukocytes with glycoprotein counter-receptors. Rolling of leukocytes exposes them to cytokines (activating peptides) and also slows down the leukocytes sufficiently to allow their firm attachment and arrest mediated by the interaction between integrins, which are activated by local cytokines, with their counter-receptors (such as intercellular cell adhesion molecule–1 [ICAM-1] and vascular cell adhesion molecule–1 [VCAM-1]). Leukocytes can then transmigrate into tissue according to chemoattractant signals, although this is not an obligatory event after leukocyte adhesion.
In acute coronary syndromes (ACS), ischemia-related hypoxia or the oxygen free radicals formed during reperfusion injury result in the rapid expression and upregulation of most of the key adhesion molecules, mediated by a host of secondary intermediates (proinflammatory cytokines, complement activation, histamine, prostaglandins, etc). These responses are involved in the clearance of necrotic tissue and structural remodeling. They also provide a target for imaging ACS. Many of these same adhesion molecules are upregulated by oxidized lipid accumulation in the vessel wall early in atherosclerosis or by leukocyte infiltration itself as part of a self-perpetuating inflammatory process late in atherosclerosis. Because of the pathophysiologic or adaptive role of adhesion molecules in multiple cardiovascular disorders, and because the binding domains for these large molecules protrude well into the lumen, they have been a popular target for ultrasound molecular imaging probes.
Another consideration is whether the signal from targeted contrast agents is affected by their attachment to cells. Microbubbles undergo volumetric oscillation in an acoustic field because they are both highly compressible and smaller in diameter than the ultrasound wavelength. Signals are produced from either stable microbubble oscillation, also known as “noninertial” cavitation, or by “transient” inertial cavitation, which is produced by acoustic pressures that are sufficient to induce microbubble disruption and collapse. Irrespective of the method of signal generation, an important issue in molecular imaging is whether physical interactions caused by cell-bubble adhesion dampen the microbubble signal, similar to placing a finger gently on a ringing bell or a vibrating guitar string. In initial in vitro studies, microbubbles that had been phagocytosed (internalized) by leukocytes produced a lower amplitude signal compared with free microbubbles, thereby confirming a damping effect from being sequestered in the intracellular milieu. Subsequent studies have demonstrated that attachment of microbubbles to a cell surface where there is asymmetric interaction with a deformable membrane does not substantially affect bubble signal irrespective of frequency or the acoustic pressure ( Figure 6 ).
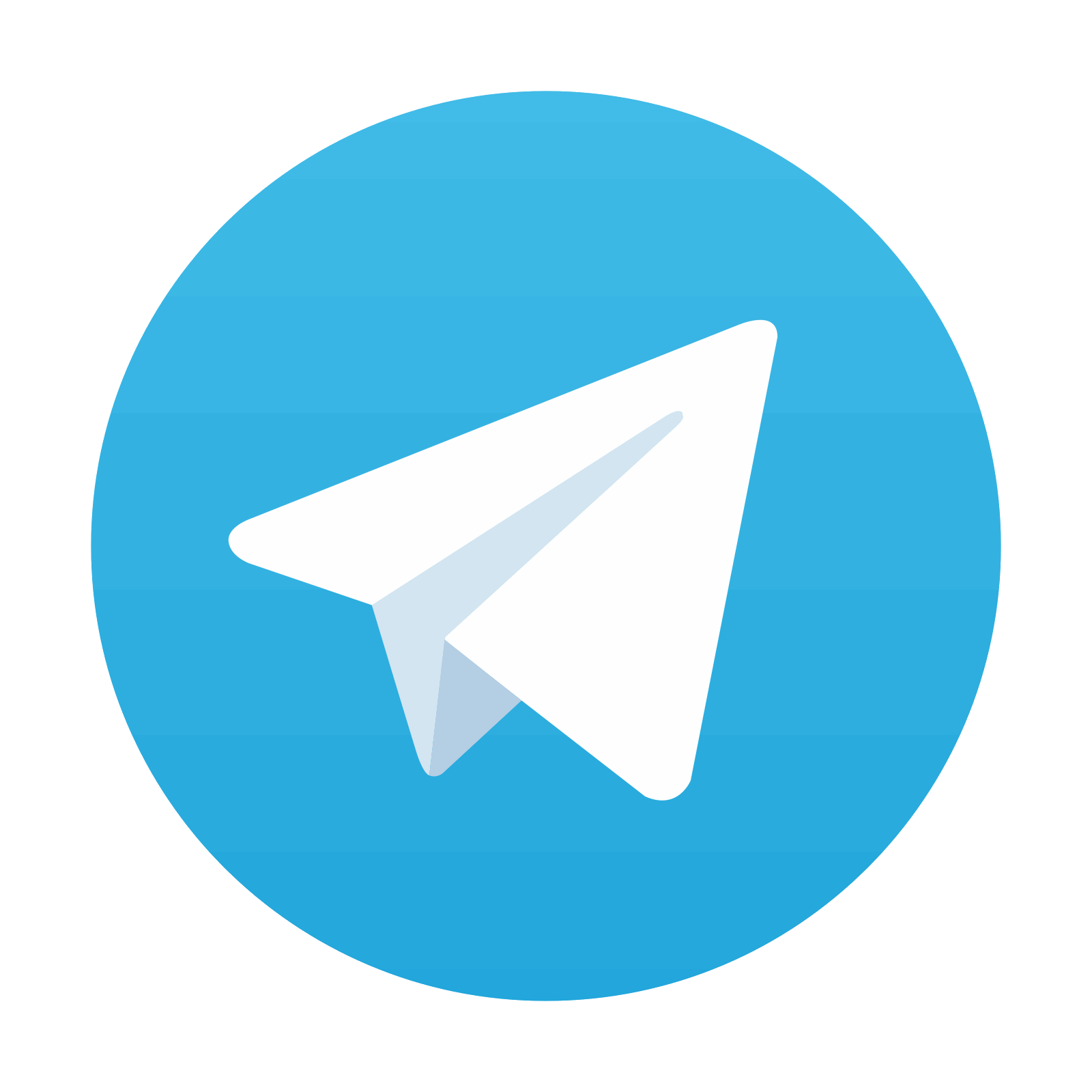
Stay updated, free articles. Join our Telegram channel

Full access? Get Clinical Tree
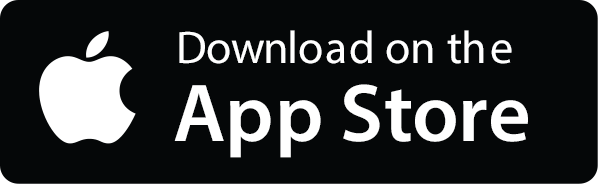
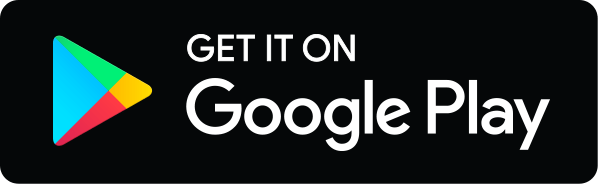
