Molecular Genetics
Qing Wang
Meredith Bond
Robert C. Elston
Xian-Li Tian
Overview
Finding the genes for a disease is considered a breakthrough discovery because it defines the genetic pathway and molecular basis underlying the pathogenesis of the disease and raises hopes that knowledge of the disease-causing genes and their functions may soon lead to accurate genetic testing, effective therapy, and prevention or cure of the disease. Identification and characterization of disease-causing genes are the central themes in the field of human molecular genetics. The process usually starts with identification of the chromosomal location (disease locus) of a disease gene by gene mapping or linkage studies or by cytogenetic analysis. Genes located within that chromosomal region can then be identified or cloned and become candidate genes for the disease. Mutation analysis of the candidate genes is then performed. Identification of disease-causing mutations in one of the genes implicates that gene in the disorder. Functional characterization of the mutations using transgenic or knockout mouse technology as well as biochemical, cell biologic, and molecular biologic studies can reveal pathogenic mechanisms underlying the disorder. The use of DNA microarrays, proteomics, and other related new technologies offers new avenues for identification of disease-linked genes and is providing a wealth of new information for diagnosis and ultimate prevention or cure of cardiovascular disease.
Glossary
Embryonic stem (ES) cells
The preimplanted embryo-derived pluripotent cells that maintain an unlimited differentiation potential and normal karyotype during the cell culture.
Expressed sequence tag (EST)
A partial single-pass sequence from either end of a cDNA clone.
Functional cloning
A technique used for cloning human disease genes based on the availability of information on the target gene and its corresponding protein product.
Genome
All the genes in an organism.
Genotype
Genetic makeup of an individual, often at a single locus.
Heterogeneity
If diseases or other phenotypes have multiple causal factors, they are considered heterogeneous. Allelic or intragenic heterogeneity refers to different genotypes at the same genetic locus that cause a similar phenotype. Locus heterogeneity refers to a situation in which a disease is caused by mutations at different genetic loci.
Linkage analysis
Analysis to determine whether alleles at two or more genetic loci (usually a disease locus and one or more marker loci) are cosegregating within a pedigree.
Linkage disequilibrium (LD)
Non-random association of alleles at two or more loci (genes or genetic markers).
Pedigree
Illustration of the relationships among family members.
Penetrance
Probability that a genotype will yield the predicted phenotype.
Phenotype
An observable biochemical, cellular, or clinical characteristic that is associated with the expression of a gene.
Polymorphisms
If there are at least two identifiable alternative gene forms (alleles) at a locus and none with frequency greater than 0.99, the locus is considered polymorphic. Commonly, any allele with a population frequency greater
than 0.01 is termed a polymorphism. Any allele with a population frequency less than 0.01 is often called a mutation. However, most people often refer to a substitution or variant as a mutation only if it can cause disease.
than 0.01 is termed a polymorphism. Any allele with a population frequency less than 0.01 is often called a mutation. However, most people often refer to a substitution or variant as a mutation only if it can cause disease.
Positional cloning
A technique used to identify the underlying genetic cause of a disease based primarily on finding the location of the defective gene.
Segregation analysis
Analysis to determine the most likely genetic model for an observed trait in a pedigree or a population: autosomal dominant, autosomal recessive, X-linked, or polygenic.
Sequence tagged site
A unique site in the genome defined by two oligonucleotide primers for polymerase chain reaction (PCR). Sequence tagged sites have become the common currency of physical mapping.
Short tandem repeat markers
Polymorphic microsatellite markers that are ideal for genotyping and linkage studies. Short tandem repeat markers can be dinucleotide, trinucleotide, or tetranucleotide repeats. Short tandem repeat markers are widely and evenly distributed across the human genome and can be typed using PCR amplification.
Single nucleotide polymorphisms (SNPs)
SNPs are single nucleotide changes or substitutions that are highly abundant and are perceived as the basis for the high-density genetic marker maps required for unraveling complex genetic traits.
Transcriptome
A set of genes expressed or transcribed from genomic DNA.
General Principles
Genetic factors have long been recognized to contribute to human phenotypes and diseases. As the incidence of nutritional and infectious diseases markedly decreases, investigators are becoming increasingly aware of the role of genes in the pathogenesis of human disease. Some human diseases, such as the long QT syndrome and hypertrophic cardiomyopathy, are strongly determined by genetic mutations. Other diseases, such as infectious diseases, are strongly determined by nongenetic or environmental factors, although it is now realized that host defense genes play an important role in susceptibility to infection. Many of the most prevalent diseases, such as coronary artery disease, hypertension, and diabetes mellitus, are determined in varying degrees by both genetic and environmental factors. Therefore, all diseases are genetic to some extent.
Advances in human molecular genetics, coupled with exponential growth in genomic research, have led to the development of a variety of technologies to identify human disease genes. Positional cloning, a primary tool used for finding disease genes, has become far easier over the years. Newly developed DNA microarray technology and proteomics offer a new alternative for identification of disease-linked genes. Transgenic and knockout mice and other technologies have been developed to assess how a mutation in a given gene affects the function of the protein product and so defines the functional role of a novel gene. The new discipline of bioinformatics, or functional genomics, has become an important tool for the rapid computer-based screening of DNA and protein databases, to identify novel genes from genomic DNA sequences, and to predict gene function. The purpose of this chapter is to review the theory and practice of these key molecular genetic technologies.
Major Types of Genetic Diseases
Genetically determined diseases can be broadly classified into four groups: chromosomal disorders, single-gene disorders, polygenic or multifactorial diseases, and mitochondrial disorders.
Chromosomal disorders are the results of deletions, duplications, or other alterations of entire chromosomes or large segments of them. These disorders include Down syndrome and DiGeorge syndrome.
Single-gene disorders are caused by single mutant genes. Human diseases caused by single genes are inherited in a simple mendelian fashion and are often referred to as Mendelian or monogenic disorders. Monogenic disorders can inherit in autosomal dominant, autosomal recessive, or X-linked fashion (Fig. 94.1). The characteristics of autosomal dominant inheritance include vertical transmission of the disease phenotype, a lack of skipped generations, and approximately equal numbers of affected male and female subjects. Father-to-son transmission may be observed, a feature that can be used to exclude X-linked inheritance. Autosomal recessive inheritance is characterized by clustering of affected individuals in the same generation, absence of disease among parents of the affected offspring, and approximately equal numbers of affected male and female subjects. Consanguinity is often a factor in recessive diseases. A major distinction between dominant and recessive disorders is that heterozygotes are clinically affected in most cases of dominant diseases, whereas they are clinically normal in the case of recessive disorders.
The first human gene to be mapped was the color blindness gene, deduced to be on the X chromosome by the distinct features of X-linked inheritance. In a pedigree with X-linked recessive inheritance, father-to-son transmission is absent, affected individuals are more frequently male, and the disease phenotype skips generations when genes are passed through female carriers. Approximately 50% of sons from the female carrier with a mutant X chromosome develop the disease phenotype.
Polygenic or multifactorial diseases such as coronary artery disease, heart failure, and hypertension result from the joint action of multiple genes and environmental factors. They are the most common human genetic diseases and pose a major challenge to contemporary human geneticists, owing to the complex nature of these conditions in which additive or interactive effects, or both, of many genes and environmental factors create a predisposition to the disease.
Mitochondrial diseases are caused by mutations on the mitochondrial genome. This group of diseases is inherited in a maternal fashion; that is, only affected female subjects can transmit the disease mutation (phenotype) to their offspring.
All the diseases discussed earlier are associated with genetic abnormalities in the DNA of all cells in the body and can be transmitted to future generations. However, a new paradigm of diseases called somatic cell genetic disorders caused by mutations in specific somatic cells has been recognized. Examples of such diseases include cancer and some vascular disorders.
Cytogenetics in Genetic Research and Molecular Diagnostics
Chromosomal (or cytogenetic) abnormalities account for at least 50% of spontaneous abortions or miscarriages and cause congenital malformations, mental disability, infertility, and certain cancers. Chromosomal abnormalities involving one chromosome include a deletion or duplication of a portion of a chromosome, a pericentric (involving the centromere) inversion or a paracentric (not involving the centromere) inversion, or the formation of a ring chromosome or isochromosome. When two or more chromosomes are involved, a translocation or exchange of chromosomal materials between the chromosomes occurs. The chromosomal abnormalities associated with cardiovascular disease include Down syndrome, DiGeorge syndrome or velocardiofacial syndrome, congenital heart defects, and Williams syndrome.
Karyotype Analysis
Karyotyping from peripheral blood is a standard technique used to examine human chromosomes and to identify chromosomal abnormalities (1). A small amount of anticoagulated blood is centrifuged to isolate white blood cells, to which are added tissue culture medium containing plant lectins such as phytohemagglutinin to stimulate them to divide. The dividing cells are then arrested at metaphase with mitotic spindle inhibitors such as colchicine and are lysed in hypotonic buffers to prepare metaphase chromosomes. Preparations of metaphase chromosomes are then stained with dyes such as Giemsa (G-banding) and are examined under a microscope. G-banding involves a series of acid, heat, or gentle trypsin treatment of chromosome preparations followed by staining with Giemsa. The alternation of chromosomal regions with high or low G/C content results in a pattern of light and dark bands that is unique for each human chromosome (Fig. 94.2A). The unique pattern for each human chromosome allows the unequivocal definition of each chromosome, and any deviations from this typical pattern may indicate occurrence of chromosomal abnormalities (Fig. 94.2B). Other chromosome banding techniques producing quinacrine (Q) bands, reverse (R), or centromeric (C) bands can be used to examine special chromosomal domains.
Fluorescence In Situ Hybridization
Fluorescence in situ hybridization (FISH) technology is a major tool for gene mapping and for identifying chromosomal abnormalities (2,3,4). In FISH analysis, DNA probes (any cloned DNA sequences, genes, microdissected fragments, or flow-sorted chromosomes) are labeled by incorporation of chemically modified nucleotides (e.g., 5-bromo-2-deoxyuridine) that fluoresce directly or can be labeled by incorporation of reporter molecules (e.g., digoxigenin or biotin). The DNA probes are then hybridized to metaphase or interphase chromosomes. Chromosomes are then counterstained with 4,6-diamino-2-phenylindole to provide high-definition banding, and hybridization signals are detected by binding a fluorescently tagged molecule to reporter molecules (e.g., anti-digoxigenin-fluorescein isothiocyanate). Chromosomes are then photographed under a fluorescence microscope (Fig. 94.2C). FISH can allow rapid mapping of any gene or DNA sequence cloned into cosmids, bacterial artificial chromosomes (BACs), or yeast artificial chromosomes (YACs) to a particular chromosome (Fig. 94.2C). FISH can also be used to detect numeric chromosomal abnormalities, including the small deletions involved in DiGeorge syndrome and Williams syndrome.
Advances in FISH technology include chromosome painting, which allows the entire chromosome to fluoresce with the chosen color (Fig. 94.2C), and multicolor FISH, which allows each human chromosome to be painted in up to 12 different colors (2). Both chromosome painting FISH and multicolor FISH can be used to identify complex chromosomal rearrangements or abnormalities rapidly. In conclusion, both karyotype analysis and FISH are powerful technologies that can be implemented in many research and diagnostic fields of human genetics.
Overview of Strategies for Disease Gene Discovery
Functional Cloning
Until the mid-1980s, the primary approach to identify the gene responsible for a human disease was through careful analysis of biochemical and physiologic differences between healthy and affected individuals. The deficient protein or enzyme from patients can be purified, and partial amino acid sequences can be obtained or antibodies against the protein can be raised. The antibodies, degenerate oligonucleotides derived from the partial amino acid sequences, or tagged fusion proteins, can then be used to screen various complementary DNA (cDNA) libraries to identify the disease gene. The approach moving from the basic biochemical defect to the disease gene is referred to as functional cloning. Sickle cell anemia caused by mutations in the hemoglobin gene was the first human disease characterized by human molecular genetics. Initially, red blood cells from affected individuals were noticed to be abnormally shaped. Later, it was found that hemoglobin from patients was electrophoretically different from normal hemoglobin, and this difference was the result of the substitution of valine for glutamic acid at the sixth amino acid position of the β-chain of hemoglobin.
Subsequently, the gene for β-hemoglobin was cloned, and the mutation of a single base substitution of A to T was identified at the nucleic acid level (5). However, the genetic origin of most diseases cannot be determined by functional cloning because of the lack of information on biochemical defects.
Subsequently, the gene for β-hemoglobin was cloned, and the mutation of a single base substitution of A to T was identified at the nucleic acid level (5). However, the genetic origin of most diseases cannot be determined by functional cloning because of the lack of information on biochemical defects.
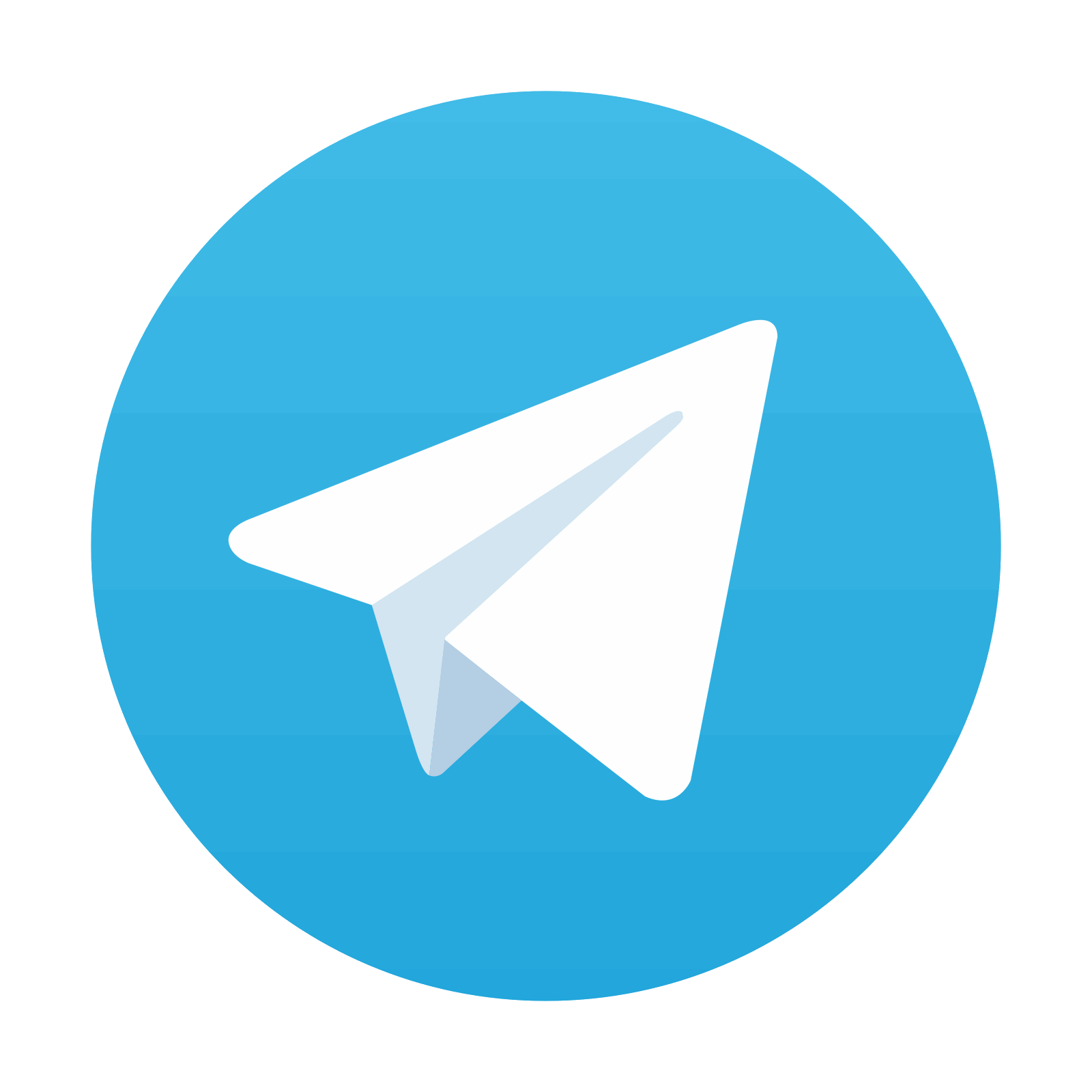
Stay updated, free articles. Join our Telegram channel

Full access? Get Clinical Tree
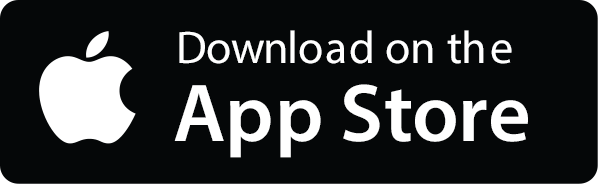
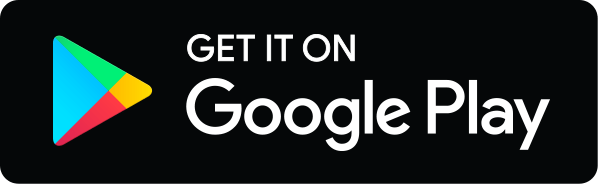