Molecular Basis of Heart Development
Madhu Gupta
Rene A. Arcilla
Mahesh P. Gupta
Overview
Heart development results from a complex but highly integrated series of interactions involving various cell types of diverse origins. These are carried out in a strictly regulated environment at both genetic and epigenetic levels. This chapter focuses on the molecular control of the sequential processes involved in heart development, from cardiac progenitors through the fully developed four-chambered heart. Recent advances in cardiomyogenesis, including commitment, differentiation, and modulation of cardiac muscle gene expression, are described. The critical role of the neural crest to cardiac development and the genetic basis for cardiac malformation are also discussed.
Molecular Changes During Heart Development
The heart is a unique organ. Its development begins shortly after the onset of embryogenesis, and it is functional long before other parts of the embryo are discernible. The process of heart development follows a complex series of events involving highly integrated and programmed morphogenetic interactions among the cells of various origins. In this section, we briefly describe the molecular changes at various morphologic stages, starting from cardiac primordia through the fully developed four-chambered heart. Table 100.1 summarizes the important genes and their functions in vertebrate heart development.
Cardiac Progenitors
For all vertebrates, the heart is derived from cells in the anterior lateral plate mesoderm (LPM), arranged in bilateral fields on either side of the precordial plate and rostral notochord. Heart specification occurs by about Hamburger Hamilton (HH) stages 3 to 4in chicks (1) and by embryonic day (E) 6.5 to 7 in mouse. In the case of humans, it occurs during the third week of embryogenesis, right after gastrulation when three germ layers are formed and simple diffusion alone is no longer adequate for the nutritional requirements of the embryo. The precursor cells of the myocardium and endocardium, and associated extracellular matrix (ECM), are colocalized in the epiblast, which ingresses through the primitive streak until the late streak stages to form bilateral heart-forming regions. In mammals and birds, the bilateral fields merge at their anterior margins to form the so-called cardiac crescent. Recent studies have identified a second type of heart field (anterior heart-forming field) located medially, adjacent to the cardiac crescent, which eventually gives rise to the anterior heart structures of the outflow tract (OT) (2). Both inductive and repressive signaling from adjacent germ layers regulate participation of mesodermal cells in the heart forming regions. The inductive role of the anterior endoderm has been shown in chick embryos; removal of these cells at HH4 prevented heart formation (3). Explants of the anterior endoderm/mesoderm underwent terminal differentiation whereas those from the anterior ectoderm/mesoderm or posterior endoderm/mesoderm did not (4). One of such inducers include bone morphogenetic proteins (BMPs), which belong to the transforming growth factor (TGF)-β superfamily of extracellular signaling peptide of which multiple members are
expressed in highly localized pattern and regulate expression of key transcription factors in cardiogenic mesoderm including Nkx2-5 and T-box (Tbx) factors (5). Fibroblast growth factor (FGF) also plays an inductive role. In the mouse embryo, FGF10 is expressed in the cells of the anterior heart forming region; in association with BMPs, it acts as an inducer for Nkx2-5 expression. The boundaries of the heart-forming regions are also delineated by repressive signals mediated by members of Wnt family that, in vertebrates, restrict the domains in which BMPs and FGFs can elicit cardiogenic response. Overexpression of Wnt3A and Wnt8 in frog embryos, or exposure of the heart field cells in chick embryos to Wnt3A and Wnt1, blocks expression of Nkx2-5, Tbx5, and cardiac differentiation (6,7). There are also endogenous Wnt antagonists, Crescent and Dkk-1, which are diffusible molecules expressed in the anterior endoderm and create a zone of low WNT signaling in the adjacent precardiac mesoderm, thereby delineating bilateral regions of anterior mesoderm capable of responding to the endoderm-derived cardiogenic signals (7).
expressed in highly localized pattern and regulate expression of key transcription factors in cardiogenic mesoderm including Nkx2-5 and T-box (Tbx) factors (5). Fibroblast growth factor (FGF) also plays an inductive role. In the mouse embryo, FGF10 is expressed in the cells of the anterior heart forming region; in association with BMPs, it acts as an inducer for Nkx2-5 expression. The boundaries of the heart-forming regions are also delineated by repressive signals mediated by members of Wnt family that, in vertebrates, restrict the domains in which BMPs and FGFs can elicit cardiogenic response. Overexpression of Wnt3A and Wnt8 in frog embryos, or exposure of the heart field cells in chick embryos to Wnt3A and Wnt1, blocks expression of Nkx2-5, Tbx5, and cardiac differentiation (6,7). There are also endogenous Wnt antagonists, Crescent and Dkk-1, which are diffusible molecules expressed in the anterior endoderm and create a zone of low WNT signaling in the adjacent precardiac mesoderm, thereby delineating bilateral regions of anterior mesoderm capable of responding to the endoderm-derived cardiogenic signals (7).
TABLE 100.1 Role of Gene Products in Different Stages of Heart Development | |||||||||||||||||||||||||||||||||||||||
---|---|---|---|---|---|---|---|---|---|---|---|---|---|---|---|---|---|---|---|---|---|---|---|---|---|---|---|---|---|---|---|---|---|---|---|---|---|---|---|
|
Formation of the Tubular Heart and Looping
As development proceeds, with the closure of the neural tube and subsequent folding of the embryo, cardiogenic area is pulled forward and rotated approximately 180 degrees along a transverse axis (HH9-10 in chicks; E7.5-8 in mouse), bringing bilaterally positioned cardiac primordial in close proximity and eventually fusing together to form the cardiac tube. Fusion of the mesodermal layers begins at the cephalic end and progresses toward the caudal end. The resulting beating tubular heart is composed of an outer myocardial and inner endocardial layer and exhibits anteroposterior (AP) patterning in which the prospective tissues of the aortic sac, OT, right ventricle, left ventricle, and atria are present in an anterior-to-posterior order along the tube. The tube also exhibits dorsoventral patterning such that the Hand1, Chisel, and Cited1 are selectively expressed at the ventral side of the linear heart tube at E8-8.5 of mouse embryo. Although the molecular events during these changes have not been fully characterized, several studies implicate retinoic acid receptor signaling pathways to play a role in processes related to AP patterning (8). Treatment with retinoic acid in chicks inhibited embryonic folding, resulting in persistence of the cardiac progenitors at their posterior and medial positions, and failure of primordial fusion at the midline. The role of cell adhesion molecules has been shown in studies where injection of antibodies against N-cadherins into cultured chick embryos (HH5–12) blocked the primordial midline fusion (9). Additionally, the transcription factor GATA-4 has also been suggested to play a role (10,11).
Subsequent development involves elongation and bending of the cardiac tube in such a manner that the cranial portion bends in a ventral and caudal direction to the right, whereas the caudal atrial portion shifts in a dorsocranial direction to the left. The rightward looping results in placement of the ventricular chambers from an AP position to a left–right orientation. Characterizing the mechanisms of looping is crucial to our understanding of several syndromes that display laterality defects (12).
The process is guided by signaling cascade along the embryonic left–right axial pathway in and around the developing heart, supposedly initiated by unidirectional movement of nodal flow. Leftward ciliary movement of node cells, known as nodal flow is the first sign of molecular left-right (LR) asymmetries. Our molecular understanding of unidirectional nodal flow is still rudimentary. Genes and their functions in this process are summarized in Table 100.2. The unidirectional movement of nodal flow eventually culminates into asymmetric expression of the homeodomain factor Pitx2 in the left LPM. In the mouse heart, the asymmetric expression of Pitx2c is
controlled by Nodal, a TGF-β–like molecule that is transiently expressed in the node and later diffuses to the left LPM where it binds to its receptor–cofactor complex (Alk4, Alk7, activin receptors IIa and IIb, and EGF–CFC cofactor) and results in phosphorylation of Smads (2 and 4). Smads interact with Foxh1 to activate Pitx2 expression only in the left LPM (13). A recent study suggests that Cited2, a transcriptional factor essential for cardiac development, is also required for Pitx2 induction (14). Lefty2 is another asymmetrically expressed molecule induced by Nodal in the left LPM and it rapidly terminates Nodal expression. On the right side, Nodal expression is inhibited by BMP signaling; the left side has no known anti-BMP activity, thus allowing Nodal to be expressed, albeit transiently. Forced expression of Pitx2 on the right side of the developing embryo is sufficient to reverse cardiac looping and heart isomerism (15).
controlled by Nodal, a TGF-β–like molecule that is transiently expressed in the node and later diffuses to the left LPM where it binds to its receptor–cofactor complex (Alk4, Alk7, activin receptors IIa and IIb, and EGF–CFC cofactor) and results in phosphorylation of Smads (2 and 4). Smads interact with Foxh1 to activate Pitx2 expression only in the left LPM (13). A recent study suggests that Cited2, a transcriptional factor essential for cardiac development, is also required for Pitx2 induction (14). Lefty2 is another asymmetrically expressed molecule induced by Nodal in the left LPM and it rapidly terminates Nodal expression. On the right side, Nodal expression is inhibited by BMP signaling; the left side has no known anti-BMP activity, thus allowing Nodal to be expressed, albeit transiently. Forced expression of Pitx2 on the right side of the developing embryo is sufficient to reverse cardiac looping and heart isomerism (15).
TABLE 100.2 Important Gene Products Involved in Unidirectional Movement of Nodal Flow and Left–Right Asymmetries | ||||||||||||||||||
---|---|---|---|---|---|---|---|---|---|---|---|---|---|---|---|---|---|---|
|
Septa and Valve Formation
Formation of endocardial cushion begins at mouse E9.0 in the heart tube, which is composed of endothelial cell layer, separated from myocardial cell layer by an ECM known as cardiac jelly. The ECM thickens in two distinct regions overlying the future atrioventricular canal (AVC) and the OT to form cardiac cushions. Shortly later, endocardial cells that line AVC and OT receive molecular signals from myocardial cells and ECM, and undergo mesenchymal transformation as they invade cardiac jelly. By E10.5, the OT but not the AV cushion is also populated by migrating neural crest cells. Subsequent growth and fusion of the cushions produce central mesenchymal mass, which further develops into mature septum and valve leaflets through a complex remodeling process. The central mesenchymal mass also fuses with the atrial septum primum and the inlet portion of the ventricular septum.
Endothelial–mesenchymal transformation (EMT) is a tightly regulated process initiated by signaling events in the myocardium and the endothelial cells in the cushion-forming regions. Molecular cascade involving TGF-β2, epidermal growth factor, platelet-derived growth factor, vascular endothelial growth factor, and transcription factors NFAT-C, Sox4, and Smad6 have all been implicated to play roles in this complex process. In normal embryos, there is specific expression of Sox4 in the endocardial cushion and ridges, and restricted but transient expression of NF-ATc in endocardial cushion, followed by OT and developing valves (16,17). Targeted disruption of NF-ATc and Sox4 in mice resulted in embryonic death before E14.5 owing to circulatory failure. These mutants showed defective development of the semilunar valves and ventricular septum; the mitral and tricuspid valves had normal development (16) or restricted development (18). Disruption of Smad6, on the other hand, leads to hyperplasia of cardiac valves and the OT (19).
Additionally, hyaluronic acid (Ha) synthetase (Has2)/ErbB2, ErbB3/Ras pathway has been shown to play essential roles (20). Ha is a prominent component of the cardiac jelly in E9.5 embryos and its production depends on Has2. It serves as a substrate for migration; it also phosphorylates ErbB2 and ErbB3 through unknown mechanisms to activate the Ras pathway. Expression of dominant-negative Ras blocks EMT in wild-type embryonic hearts, suggesting that activation of Ras is required for EMT (21). In addition, Wnt/β-catenin signaling has also been suggested to play a role in EMT and subsequent valvulogenesis. Regionally restricted expression of Wnt/β-catenin has been shown in the mouse developing heart (22), and homozygous zebra fish mutants of β-catenin display massive expansion of cardiac cushion throughout the heart owing to uncontrolled EMT of the entire endothelial lining (23).
Myocardial Trabeculation
At the final stages of looping, the myocardium is a compact epithelial tissue layer formed exclusively of myocytes. In the ventricular region, wide intercellular spaces start appearing between the myocardial cells, separating the myocardium into two distinct zones: a compact outer layer and an inner spongy layer. Primitive trabeculae appear as endocardial outpockets at discrete points directed toward the myocardium, resulting in myocardial cell separation and subsequent penetration by the endocardium. Lateral expansion into the previously formed intercellular spaces later assumes a more complex pattern. Most of the primitive trabeculae eventually disappear, but some coalesce to form papillary muscles or trabecula carneae of the mature heart. Continued trabeculae formation is important because it prevents unrestricted myocyte growth during cardiogenesis while at the same time ensuing potential chamber cavity preservation. The net result is a ventricular structure that is neither solid or excessively thick walled and, consequently, able to perform the fundamental pump function during all stages of cardiac development and thereafter.
Signaling events between the endocardium and the myocardium are important for ventricular growth. Cell-surface receptors ErbB2 and ErbB4, both members of the epidermal growth factor receptor family, are involved in ventricular trabeculae formation. Transcripts of these receptors have been detected in the myocardium but not in the endocardium of the developing heart (24). Their ligands, neuregulins, are expressed
in the endocardium. Mice deficient in either receptors or ligands die during midembryogenesis and show aborted development of myocardial trabeculae in the ventricle (25).
in the endocardium. Mice deficient in either receptors or ligands die during midembryogenesis and show aborted development of myocardial trabeculae in the ventricle (25).
Conduction System
Establishment of the cardiac conduction system represents another process of cellular diversification of the developing myocardium. It is not precisely known when this system develops; however, experimental evidence suggests that the conduction system is already functional during the final stages of cardiac loop formation. Conduction fibers express relatively higher levels of Nkx2-5. Tbx-5 has also been implicated in the formation of functional conduction system. Connexin 40, a gap junctional protein, is a downstream target for these transcription factors. A zinc finger transcription factor, HF1b has also been shown to be critical to the establishment of conduction system (reviewed in Moorman and Christoffels [8]).
Neural Crest Cells
The neural crest exists only briefly during early embryonic development. It constitutes a population of cells initially contained in the neural folds, which are released upon closure of the neural plate. The neural crest is divided into two parts: a cranial region and a trunk region. A portion of the cranial region extending between midotic placodes and somite 3 is known as the cardiac neural crest. The cardiac neural crest cells migrate to, and populate, pharyngeal arches 3, 4, and 6 to participate in the development of the major branches of the aortic arch. Ablation of the cardiac neural crest, either surgically or chemically, results in predictable cardiac malformations that include persistent truncus arteriosus and double-outlet right ventricle. Despite the critical role played by the neural crest in cardiovascular development, little is known about its contributions at the molecular level. Suggested molecules include retinoic acid, vitamin A, endothelin-1, or endothelin-A receptor (26). Mice deficient in these molecules display similar cardiovascular abnormalities to those seen after neural crest ablation. Endothelin-3 has been shown to promote neural crest cell proliferation in a cell culture model (27). Candidate genes for neural crest origin belong to the homeodomain family of transcription factors such as Pax 3, Msx1, and Msx2. Transgenic mice deficient in one of these regulatory molecules display normal cardiovascular development, but double homozygous-deficient mice show cardiac defects, suggesting that these transcription factors may cooperate in the regulation of as yet unidentified target genes (reviewed in Epstein [28]). Other genes of cardiac neural crest origin include the bHLH gene products dHAND and eHAND, which are expressed in crest-derived tissue structures (29).
Molecular Regulation of Cardiac Myogenesis
Myogenesis of cross-striated muscle consists of three basic developmental stages: (a) commitment (also known as determination), (b) differentiation, and (c) modulation (or maturation). During commitment, the mesodermal cell is specified to a muscle lineage such that the committed cell has restricted potential. Differentiation consists of expression of cardiac-specific proteins such as myosins, actins, troponins, tropomyosins, and channel proteins. Modulation
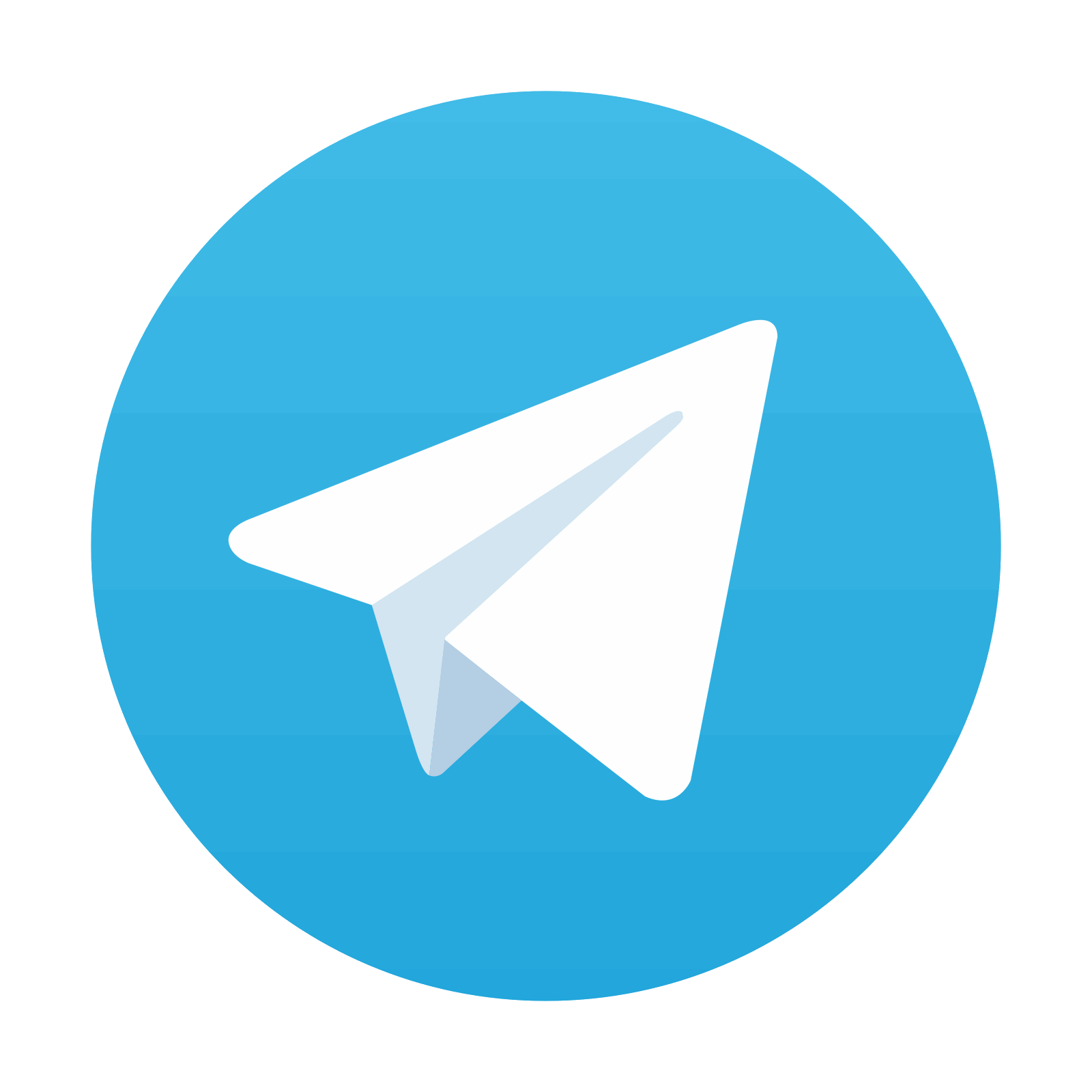
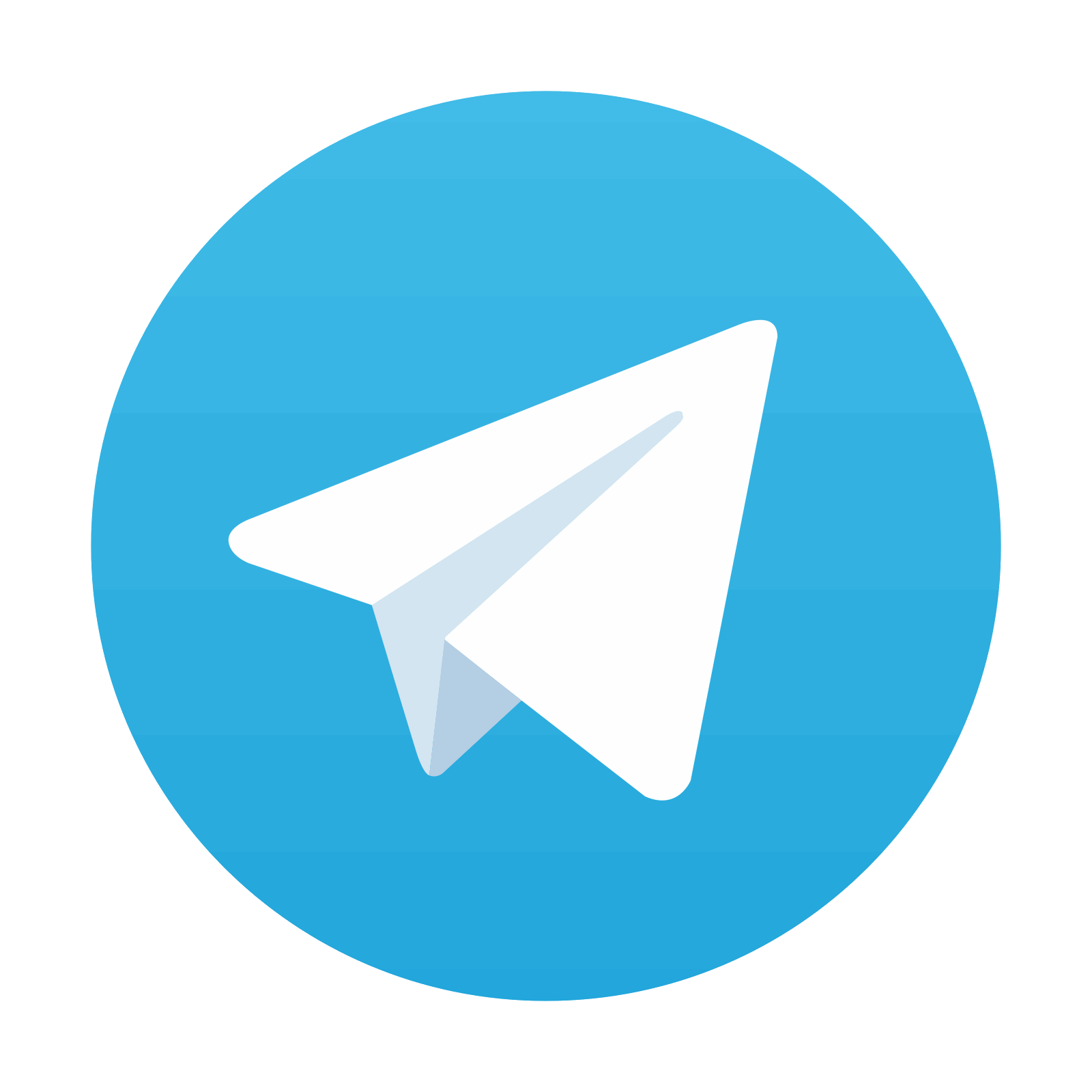
Stay updated, free articles. Join our Telegram channel

Full access? Get Clinical Tree
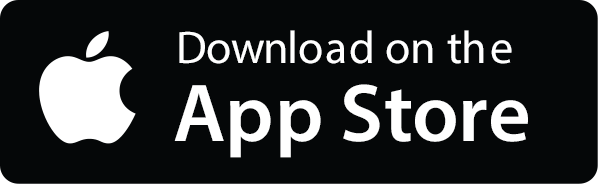
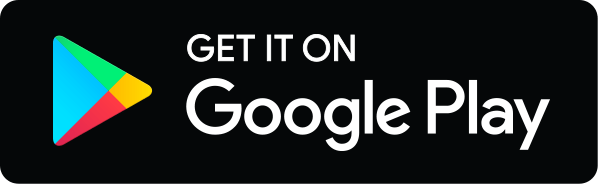