Experimental models and observational studies suggest that homocysteine-lowering therapy with folic acid (FA) may prevent cardiovascular disease (CVD). However, FA also stimulates cell proliferation and might promote progression of atherosclerosis. Our objectives were to perform a meta-analysis of FA supplementation trials on CVD events and to explore a potential interaction between FA supplementation and baseline homocysteine levels on CVD events. We searched MEDLINE for randomized controlled trials of FA supplementation to prevent CVD events (January 1966 to July 2009) and performed meta-analyses using random effects models. For trials that reported responses to FA supplementation stratified by baseline levels of homocysteine, we pooled within-trial estimates of differences in log-relative risks by baseline homocysteine levels using a random effects model. Overall, FA supplementation did not affect primary cardiovascular clinical end points (relative risk 1.02, 95% confidence interval [CI] 0.93 to 1.13, p = 0.66) or stroke (relative risk 0.95, 95% CI 0.84 to 1.08, p = 0.43). However, in trials that reported analyses stratified by baseline homocysteine, effect of FA supplementation differed by strata of baseline homocysteine (p for interaction = 0.030). Specifically, risks of primary clinical CVD events comparing FA supplementation to control were 1.06 (95% CI 1.00 to 1.13) in strata with mean baseline homocysteine levels >12 μmol/L and 0.94 (95% CI 0.86 to 1.03) in strata with baseline homocysteine levels <12 μmol/L. In conclusion, FA had no effect on CVD or stroke. However, analysis of within-trial results stratified by baseline homocysteine suggests potential harm in those with high homocysteine at baseline. This interaction may have important implications for recommendations of FA supplement use. In the meantime, FA supplementation should not be recommended as a means to prevent or treat CVD or stroke.
Premature atherosclerosis is commonly observed in patients with homocystinuria. In epidemiologic studies, increased homocysteine is a risk factor for cardiovascular disease (CVD) independently of traditional CVD risk factors such as age, blood pressure, lipids, or smoking. Evidence from in vivo studies has suggested that homocysteine promotes endothelial damage and thrombus formation, which are precedents of embolic stroke and myocardial infarction. Although these clinical, epidemiologic, and mechanistic studies have provided strong justification for conducting randomized trials of homocysteine-lowering interventions, large clinical trials of folic acid (FA) supplementation have been for the most part disappointing, although it was still possible that FA supplementation decreased the risk of stroke. Since this meta-analysis, several new trials have been published or have released initial data. Two of these trials with overall null results have shown an interaction between FA supplementation and baseline homocysteine levels, namely compared to control FA supplementation increases the risk of CVD and thromboembolic events in participants with higher baseline homocysteine levels. To provide more stable estimates of the efficacy of FA supplementation, particularly for the prevention of stroke, we performed a meta-analysis of FA supplementation and CVD risk and determined whether the effects of FA differ by level of baseline homocysteine.
Methods
We used the MEDLINE search strategy developed by Bazzano et al in a previous meta-analysis of randomized controlled trials testing the effect of FA supplementation on CVD risk (the exact search terms are shown in the Appendix ). Because the search period for the previous meta-analysis was January 1966 to July 2006, we updated the search from July 1, 2006 through June 1, 2009, with no language restrictions. We also conducted a manual search for unpublished results of ongoing trials and presentations at significant scientific meetings.
The prespecified inclusion criteria were as follows: (1) the study design was a randomized controlled trial; (2) the intervention included FA supplementation; (3) the intervention and control groups reported the number of events for CVD, coronary heart disease (CHD), stroke, deep vein thrombus (DVT) or pulmonary emboli (PEs), or all-cause mortality; and (4) the intervention lasted ≥6 months. We excluded trials of pregnant women, children, or patients with end-stage renal disease. We excluded trials of patients with end-stage renal disease because normalization of homocysteine with FA supplementation is not achievable in these patients, extremely high doses are required to achieve modest homocysteine decreases, and these patients are at such high risk for CVD events that any single risk factor modification is likely to have minimal impact.
Three investigators (ERM, SJ, RP-B) independently abstracted the articles. Disagreements or uncertainties were resolved by consensus. The number of events for these clinical outcomes with the longest follow-up was abstracted from the primary publication of each trial. For trials with no composite of total CHD events, we used the number of patients with myocardial infarction.
For those trials reporting results stratified by baseline homocysteine levels, we abstracted the number of participants and primary clinical events in intervention and control groups within each stratum. If these numbers were not reported, we abstracted stratum-specific relative risks (adjusted or unadjusted risk ratios, rate ratios, or hazard ratios) and their 95% confidence intervals (CIs) for the primary clinical end point comparing FA supplementation to control. If available, we also abstracted mean baseline homocysteine levels within each stratum.
All analyses were conducted according to the intention-to-treat principle. Results from factorial trials were based on 2-way analyses, that is, all trial participants receiving FA supplementation were compared to all participants not receiving it regardless of other factorial interventions. For each selected trial, net change in homocysteine was computed as the change in mean homocysteine level from baseline to after intervention in the FA group minus the corresponding change in the control group. Because correlations between baseline and postintervention homocysteine levels were not reported in most trials, variance of the net change was calculated assuming a common pooled correlation coefficient for all trials. Risk ratios for the primary clinical end point, CVD, CHD, stroke, and all-cause mortality, comparing FA supplementation to control were estimated, in addition to their corresponding variances on the log scale, from the reported number of participants and events in each group.
Pooled estimates and 95% CIs of net change in homocysteine and log-transformed risk ratio for each clinical outcome were calculated using inverse-variance weighted random-effects models. Between-study heterogeneity was quantified with the I 2 statistic, which estimates the proportion of total variation in study estimates due to heterogeneity. Publication bias and other small-study effects were assessed using the extended Egger test allowing for residual heterogeneity.
For results stratified by baseline homocysteine levels, we used risk ratios derived from stratum-specific counts or reported relative risks (risk ratios, rate ratios, or hazard ratios) within each stratum because not all trials provided complete information on stratum-specific event numbers. If not explicitly reported, mean baseline homocysteine levels within each stratum were estimated from the appropriate truncated sections of the overall within-trial homocysteine distribution, which was assumed to follow a normal distribution with the reported baseline mean ± SD. To pool stratified results across trials, we performed an inverse-variance weighted random-effects meta-regression of log-transformed stratum-specific relative risks on corresponding mean baseline homocysteine levels. The slope of this model quantifies the interaction between FA supplementation and baseline homocysteine levels as percent change in relative risk for the primary clinical end point comparing FA supplementation to control per 1-μmol/L increase in baseline homocysteine. We also calculated pooled relative risks comparing FA supplementation to control for strata below and above the overall mean baseline homocysteine level of 12 μmol/L.
Sensitivity analyses were performed to evaluate the relative influence of each trial on pooled estimates by removing each study from the analysis. Potential confounding based on country-specific FA food fortification policy was also examined in a subgroup analysis. All analyses were conducted with S-PLUS 2000 (Mathsoft, Inc., Seattle, Washington). We adhered to the QUality Of Reporting Of Meta-analyses (QUOROM) guidelines for reporting meta-analysis.
Results
Fourteen randomized controlled trials of the effect of FA supplementation on CVD risk met our inclusion criteria ( Figure 1 ). Of the 12 trials included in the meta-analysis of Bazzano et al, we excluded 4 trials conducted in patients with end-stage renal disease. The updated 2006 to 2009 search yielded 5 new trials, and 1 additional report of an unpublished study was identified by manual search. Participants’ characteristics and design features of the 14 selected trials are presented in Table 1 . The studies were published from 2002 to 2009, and most were conducted in Europe and the United States. The total number of participants randomized in the 14 trials was 38,941, with individual trials ranging from 240 to 12,064 participants. Nine trials recruited patients after acute CVD events, including acute myocardial infarction or unstable angina, coronary artery stenting or angioplasty, nondisabling ischemic stroke, and DVT or PE ; 4 trials enrolled patients with pre-existing CVD or subjects at high risk of CVD; and 1 trial targeted subjects at low risk of CVD. Mean age ranged from 52.2 to 68.9 years and mean baseline homocysteine levels varied from 9.6 to 13.5 μmol/L.
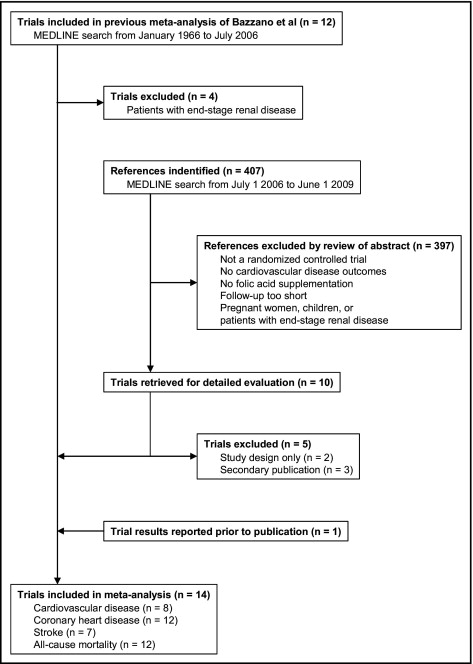
Trial | Country | Target Population | Age (years), Mean ± SD | Men (%) | Baseline Homocysteine (μmol/L), Mean ± SD | Folic Acid Dosage (mg/day) | Vitamin B12/B6 Dosage (mg/day) | Control | Blinding | Mean Follow-up (months) | Primary Clinical End Point |
---|---|---|---|---|---|---|---|---|---|---|---|
Baker et al, 2002 (CHAOS-2) | United Kingdom | recent acute coronary syndrome or stable angina | not reported | not reported | 11.2 ± 6.9 | 5 | None | placebo | double | 20 | composite of revascularization, nonfatal myocardial infarction, and cardiovascular disease death |
Schnyder et al, 2002 (Swiss Heart Study) | Switzerland | recent successful coronary angioplasty | 62.6 ± 10.8 | 80.5 | 11.2 ± 4.7 | 1 | 0.4/10 | placebo | double | 11 | composite of revascularization, nonfatal myocardial infarction, and death |
Lange et al, 2004 | Germany, Netherlands | recent successful coronary stenting | 61.3 ± 10.3 | 77.0 | 12.6 ± 4.9 | 1.2 | 0.06/48 | placebo | double | 8 | composite of revascularization, nonfatal myocardial infarction, and cardiac death |
Liem et al, 2004 (FOLARDA) | Netherlands | recent acute myocardial infarction in patients with hypercholesterolemia | 59.0 | 69.5 | not reported | 5 | None | usual care | open | 12 | composite of recurrent ischemia, recurrent nonfatal myocardial infarction, and cardiovascular disease death |
Toole et al, 2004 (VISP) | United States, Canada, Scotland | recent nondisabling ischemic stroke | 66.3 ± 10.8 | 62.5 | 13.4 ± 4.9 | 2.5 | 0.4/25 | placebo | double | 20 | composite of recurrent ischemic stroke, coronary heart disease events, and death |
Liem et al, 2005 (Goes Extension Study) | Netherlands | stable coronary artery disease in patients on statin therapy | 65.2 ± 9.8 | 78.0 | 12.1 ± 4.3 | 0.5 | none | usual care | open | 42 | composite of recurrent nonfatal acute coronary syndrome, nonfatal stroke, transient ischemic attack, and death |
Bonaa et al, 2006 (NORVIT) | Norway | recent acute myocardial infarction | 63.0 ± 11.7 | 73.9 | 13.1 ± 5.2 | 0.8 | 0.4/40 | placebo | double | 36 | composite of recurrent myocardial infarction, stroke, and cardiac death |
Lonn et al, 2006 (HOPE-2) | 13 countries | history of cardiovascular disease or diabetes | 68.9 ± 7.0 | 71.8 | 12.2 ± 5.4 | 2.5 | 1/50 | placebo | double | 60 | composite of nonfatal myocardial infarction, nonfatal stroke, and cardiovascular disease death |
den Heijer et al, 2007 (VITRO) | Netherlands, Italy, Austria | previous deep vein thrombosis or pulmonary embolism | median 52.2 | 51.6 | geometric mean 11.9 | 5 | 0.4/50 | placebo | double | 30 | recurrent deep vein thrombosis or pulmonary embolism |
Albert et al, 2008 (WAFACS) | United States | history of cardiovascular disease or ≥3 cardiovascular disease risk factors | 62.8 ± 8.8 | 0.0 | median (interquartile range) 12.3 (5.4) | 2.5 | 1/50 | placebo | double | 88 | composite of revascularization, nonfatal myocardial infarction, nonfatal stroke, and cardiovascular disease death |
Collins et al, 2008 (SEARCH) | United Kingdom | history of myocardial infarction and current statin use or indication | 64.0 ± 9.0 | 83.0 | 13.5 ± 5.0 | 2 | 1/none | placebo | double | 80 | composite of revascularization, recurrent nonfatal myocardial infarction, stroke, and coronary heart disease death |
Ebbing et al, 2008 (WENBIT) | Norway | coronary artery disease or aortic valve stenosis | 61.6 ± 10.0 | 79.5 | 10.8 ± 4.6 | 0.8 | 0.4/40 | placebo | double | 38 | composite of nonfatal myocardial infarction, unstable angina, nonfatal ischemic stroke, and death |
Hodis et al, 2009 (BVAIT) | United States | no evidence of cardiovascular disease or diabetes | 61.4 ± 9.8 | 61.1 | 9.6 ± 3.6 | 5 | 0.4/50 | placebo | double | 37 | cardiovascular disease events |
Imasa et al, 2009 | Philippines | recent non–ST-elevation myocardial infarction or unstable angina | 59.4 | 57.9 | 12.8 | 1 | 0.4/10 | placebo | double | 6 | composite of rehospitalization, recurrent nonfatal acute coronary syndrome, and death |
⁎ All trials used a parallel-group design except for Bonaa et al, Albert et al, Collins et al, and Ebbing et al, which used a factorial design. In Bonaa et al and Ebbing et al, 1/2 of participants in the intervention (folic acid plus vitamin B12) and control groups received vitamin B6. In Albert et al, participants in an ongoing 2 × 2 × 2 factorial trial of antioxidant vitamins (C, E, and β-carotene) were subsequently randomized to receive a combination of folic acid, vitamin B12, and vitamin B6 or placebo. In Collins et al, participants in the intervention (folic acid plus vitamin B12) and control groups were also randomized to receive standard-dosage (20 mg/day) or high-dosage (80 mg/day) simvastatin therapy. Albert et al and Hodis et al were conducted in countries with mandatory folic acid fortification programs. Toole et al and Lonn et al included subjects from countries with and without folic acid fortification programs.
The FA doses in the homocysteine-lowering regimens ranged from 0.5 to 5 mg/day. Three trials used FA supplements alone, and the other 11 trials used FA in combination with vitamins B12 and/or B6. Two trials were conducted in countries with mandated FA fortification programs and 2 included countries with and without fortication. All but 2 trials were placebo-controlled and double-blind. Except for 1 trial examining recurrent DVT or PE, the primary clinical outcomes were composite CVD events, including combinations of revascularization, nonfatal myocardial infarction, nonfatal stroke, sudden cardiac death, CVD mortality, and total mortality. Average follow-up ranged from 6 months to 7.3 years.
All trials found a significant effect of FA supplementation on lowering homocysteine levels, with net decreases ranging from 1.5 to 4.5 μmol/L (percent decreases with respect to mean baseline homocysteine levels from 13.0% to 38.2%; Table 2 ). The pooled net decrease in homocysteine comparing FA supplementation to control was 2.9 μmol/L (95% CI 2.4 to 3.4 μmol/L, p <0.001; Figure 2 ), with strong heterogeneity across trials (I 2 = 91%, 95% CI 86% to 94%).
Trial | Number of Participants in FA/Control Groups | Change in Homocysteine From Baseline ⁎ | Number of Events in FA/Control Groups | |||||
---|---|---|---|---|---|---|---|---|
Net (μmol/L) | Percent | Primary End Point | CVD | CHD | Stroke | All-Cause Mortality | ||
Baker et al, 2002 (CHAOS-2) | 942/940 | −1.5 | −13.4 | 23/12 | not reported | 23/12 | not reported | not reported |
Schnyder et al, 2002 (Swiss Heart Study) | 272/281 | −2.9 | −25.7 | 42/64 | not reported | 42/64 | not reported | 4/8 |
Lange et al, 2004 | 316/320 | −3.6 | −28.7 | 53/35 | not reported | 53/35 | not reported | 1/1 |
Liem et al, 2004 (FOLARDA) | 140/143 | not reported | not reported | 43/45 | not reported | 43/45 | not reported | 6/7 |
Toole et al, 2004 (VISP) | 1,827/1,853 | −2.3 | −17.2 | 303/316 | 249/257 | 114/123 | 152/148 | 99/117 |
Liem et al, 2005 (Goes Extension Study) | 300/293 | −2.6 | −21.5 | 49/56 | 49/56 | 23/18 | 8/12 | 18/26 |
Bonaa et al, 2006 (NORVIT) | 1,872/1,877 | −3.5 | −27.0 | 369/347 | 369/347 | 329/314 | 49/49 | 184/181 |
Lonn et al, 2006 (HOPE-2) | 2,758/2,764 | −3.2 | −26.3 | 519/547 | 519/547 | 341/349 | 111/147 | 470/475 |
den Heijer et al, 2007 (VITRO) | 353/348 | −4.5 | −38.2 | 43/50 | not reported | not reported | not reported | not reported |
Albert et al, 2008 (WAFACS) | 2,721/2,721 | −1.6 | −13.0 | 406/390 | 406/390 | 283/280 | 79/69 | 250/256 |
Collins et al, 2008 (SEARCH) | 6,033/6,031 | −3.7 | −27.2 | 1,537/1,493 | 1,537/1,493 | 1,229/1,185 | 269/265 | 983/950 |
Ebbing et al, 2008 (WENBIT) | 1,540/1,550 | −2.6 | −24.5 | 219/203 | 219/203 | 135/113 | 28/39 | 73/58 |
Hodis et al, 2009 (BVAIT) | 254/252 | −2.1 | −21.8 | 9/11 | 9/11 | not reported | not reported | 0/2 |
Imasa et al, 2009 | 116/124 | not reported | not reported | 46/35 | not reported | 15/13 | not reported | 22/20 |
⁎ Net change was the change in mean homocysteine level from baseline to after intervention in the folic acid group minus that change in the control group. Percent change was the net change divided by the mean baseline homocysteine level pooled across groups.
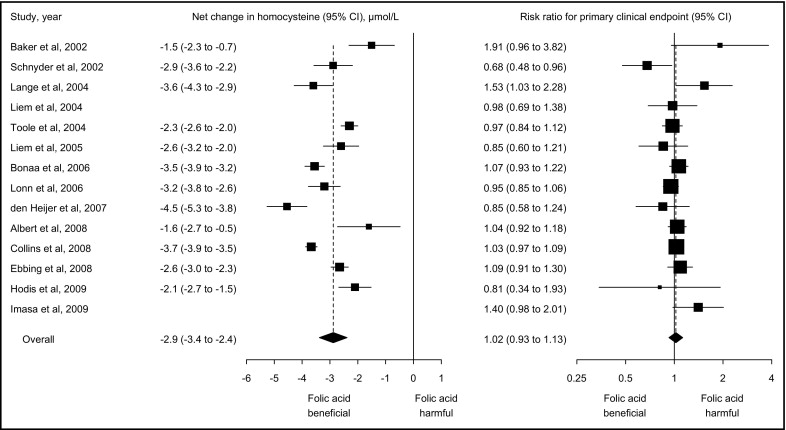
Despite the significant decrease in homocysteine, FA supplementation had no effect on primary clinical end points. Across all 14 trials, average risks for the primary clinical end point were 18.8% (3,661 events in 19,444 participants) in the FA group and 18.5% (3,604 events in 19,497 participants) in the control group ( Table 2 ). The pooled risk ratio for the primary clinical end point comparing FA supplementation to control was 1.02 (95% CI 0.93 to 1.13, p = 0.66; Figure 2 ), with moderate heterogeneity across trials (I 2 = 38%, 95% CI 0% to 67%) and no evidence of publication or related biases (p = 0.63). The pooled risk ratio estimate was insensitive to the exclusion of each trial from the analysis (range of risk ratios 1.01 to 1.03). Subgroup analyses by FA fortification status revealed no significant difference in baseline homocysteine levels (p = 0.83), net homocysteine decrease (p = 0.12), and primary clinical effects (p = 0.17) between countries with (n = 4) and without (n = 8) FA fortification.
Similarly, FA supplementation did not affect risk for CVD, CHD, stroke, and all-cause mortality ( Figure 3 ). Pooled risk ratios comparing FA supplementation to control were 1.02 (95% CI 0.98 to 1.06, p = 0.42) for CVD, 1.04 (95% CI 0.94 to 1.16, p = 0.42) for CHD, 0.95 (95% CI 0.84 to 1.08, p = 0.43) for stroke, and 1.01 (95% CI 0.95 to 1.07, p = 0.78) for all-cause mortality. There was no evidence of heterogeneity (I 2 = 0% for CVD, 31% for CHD, 25% for stroke, and 0% for all-cause mortality) or publication bias (p = 0.46, 0.22, 0.30, and 0.21, respectively) for any of these clinical outcomes. After removing each trial from analysis, pooled risk ratios ranged from 1.01 to 1.03 for CVD, 1.02 to 1.06 for CHD, 0.92 to 1.01 for stroke, and 0.98 to 1.02 for all-cause mortality.
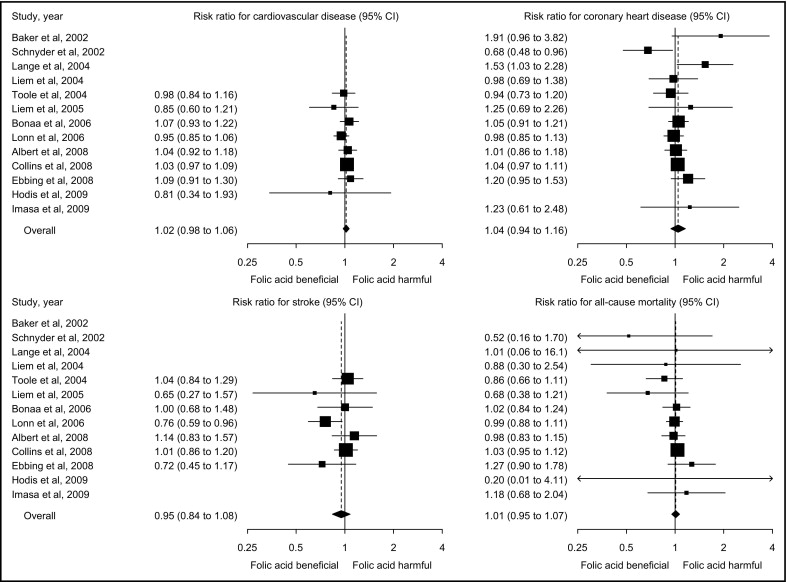
Six trials with a total of 23,986 participants reported results stratified by baseline homocysteine levels ( Table 3 ). In most of these trials, FA supplementation decreased CVD risk in participants with lower baseline homocysteine levels and increased risk in participants with higher baseline homocysteine levels. On average, the pooled relative risk for the primary clinical end point comparing FA supplementation to control increased by 3.9% (95% CI −3.0 to 11.3, p = 0.27; Figure 4 ) per each 5-μmol/L increase in baseline homocysteine, with no heterogeneity of stratum-specific effects with respect to pooled linear trend (I 2 = 8%, 95% CI 0 to 46). The influence of each trial on the pooled trend was assessed by excluding individual trials from analysis. Pooled relative risks comparing FA supplementation to control increased from 2.2% to 10.2% per each 5-μmol/L increase in homocysteine increase after excluding individual trials.
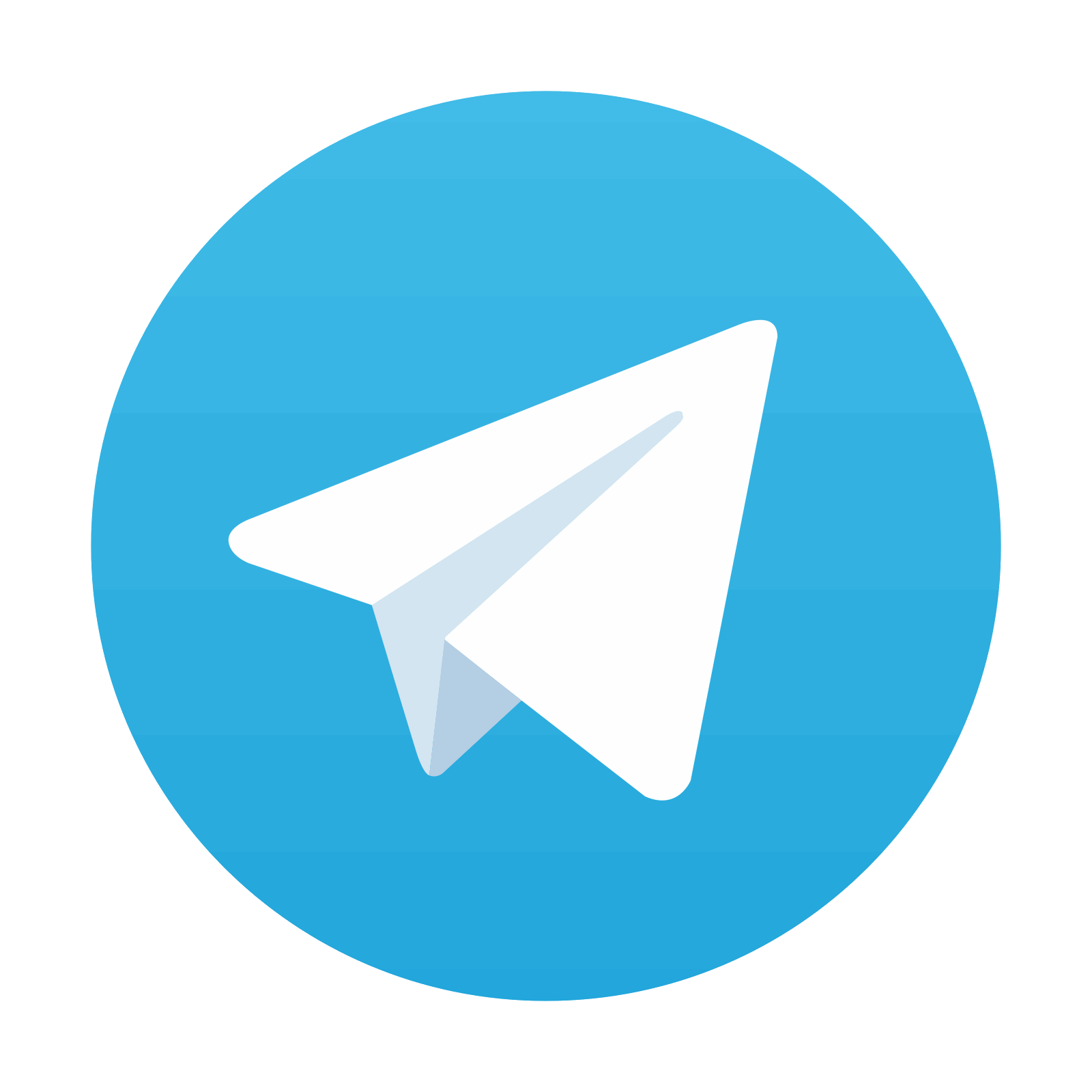
Stay updated, free articles. Join our Telegram channel

Full access? Get Clinical Tree
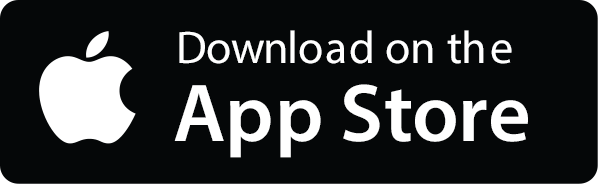
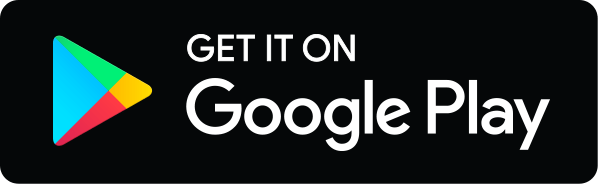
