Newborn
1 year
7 years
Adult
Compliance (ml/cm H2O)
5
15
50
60–100
Resistance (cm H2O/l/s)
40
15
4
2
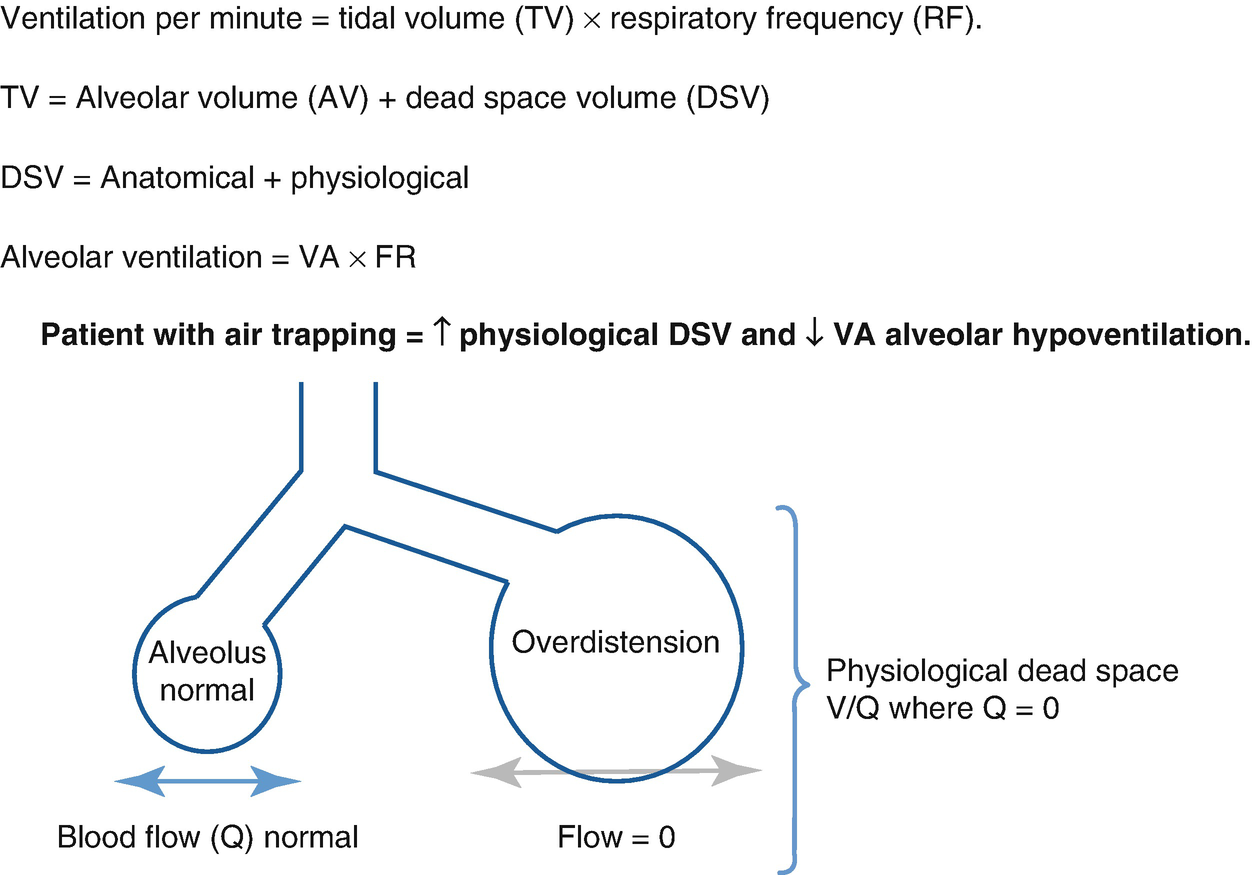
Ventilation per minute and physiological dead space
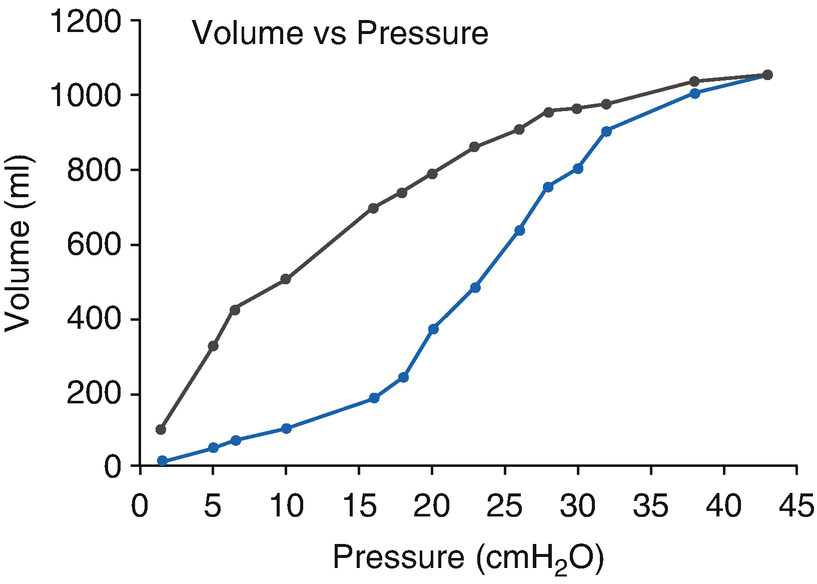
Lung compliance and hysteresis curve
The airway resistance is the pressure difference between the mouth and the alveolus needed to move air through the airway in a constant flow. It is determined by the flow rate, the length of the airway, the physical properties of the inhaled gas, and the radius of the airway, the latter being the most important variable.
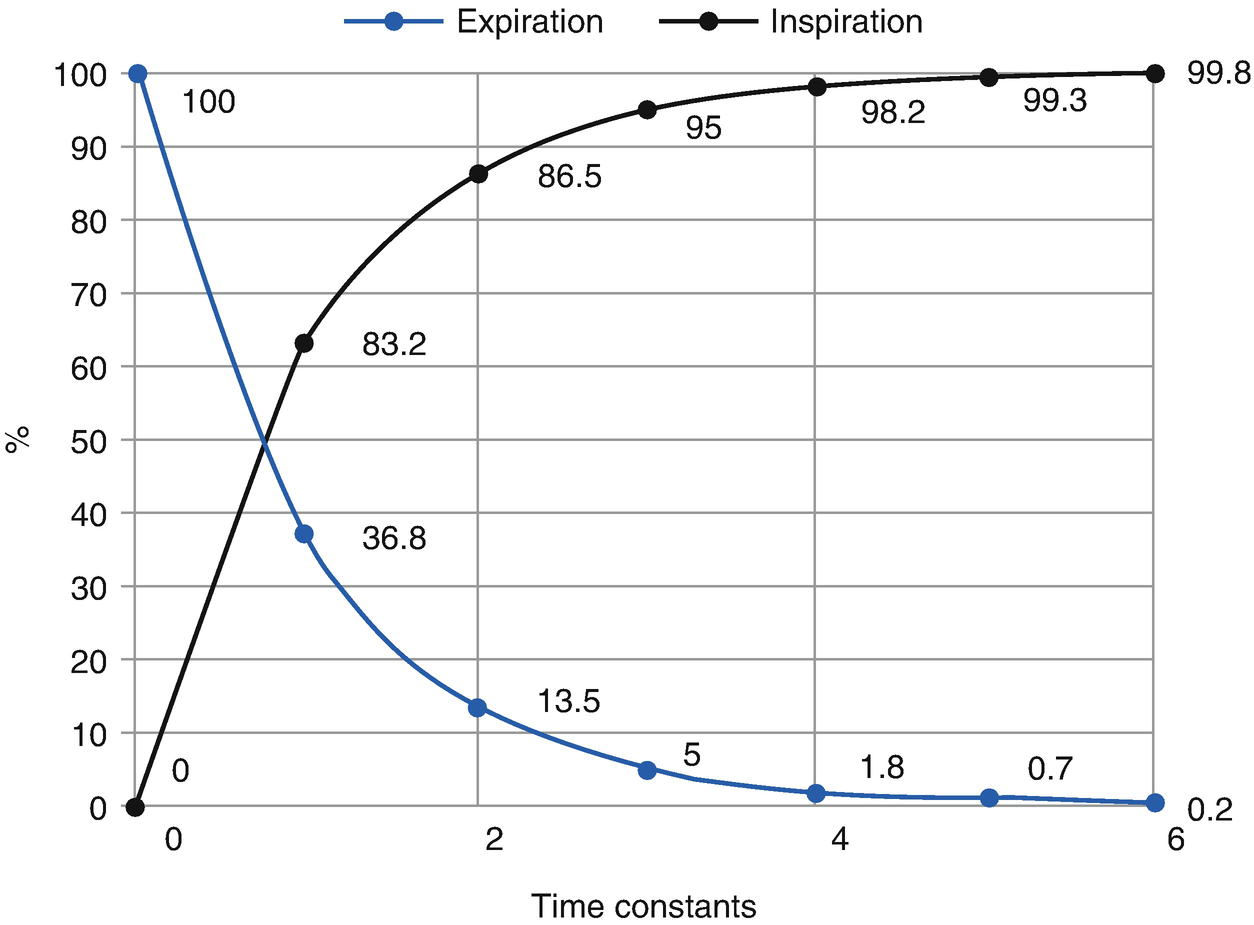
Relationship between pressure equilibrium in the airway and the time constant
All ventilation methods require that a pressure gradient is established between the alveoli and the airway (or atmospheric pressure) to produce a gas movement. Thus, adequate pressure must be generated to open the collapsed airway, so that any method that does not reach that critical pressure point during inspiration will determine the production of atelectasis and hypoxemia. The maximum pressure generated during the inspiratory phase of the mechanical ventilation that allows the airflow to overcome the airway resistance is known as maximum inspiratory pressure (MIP). This is proportional to the resistance and the tidal volume or mobilized volume during inspiration, and it is inversely proportional to lung compliance.
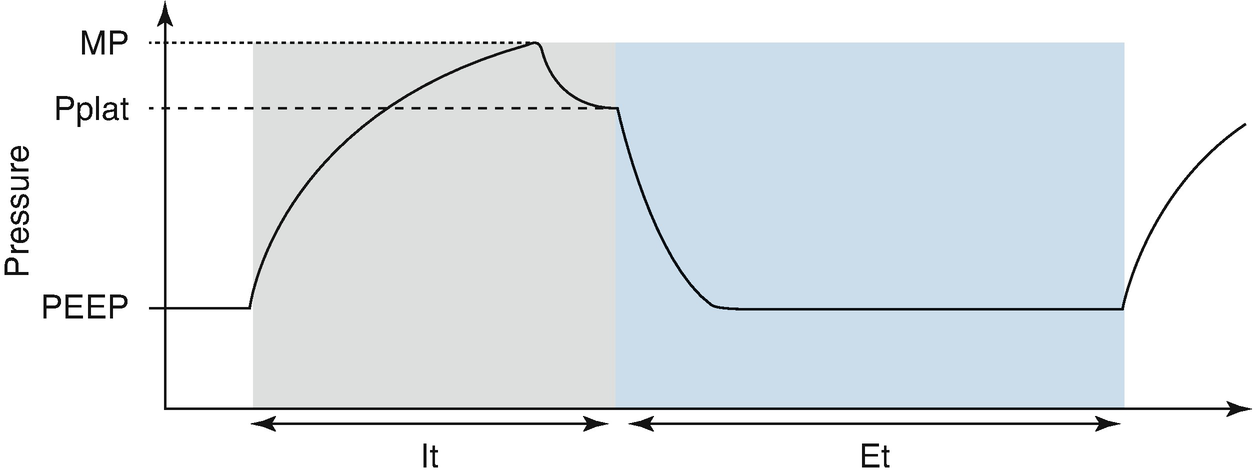
Pressure/volume relationship in volume-control mode. MIP Maximal inspiratory pressure, Pplat plateau pressure, PEEP positive end expiratory pressure, It Inspiratory time, Et Expiratory time
Basic Elements of the Cardiopulmonary Interactions
During mechanical ventilation, a series of interactions are caused between the blood flow that enters and leaves the heart and the moments in which the lung is in inspiration or expiration as a consequence of the intrathoracic pressure variation. This intrathoracic pressure varies with the ventilatory maneuvers, and it affects the pressure gradient between the blood that returns to the heart (venous return) and the blood that leaves the thorax (stroke volume of the left ventricle).
In positive pressure ventilation, the increase of lung volume produces an increase in intrathoracic pressure, which is transmitted to all structures inside the thorax, affecting atria filling and ventricular ejection. During inspiration of positive pressure ventilation, the increase of intrathoracic pressure will determine an increase of pressure in the right atrium and a decrease of the venal return due to the decrease of the pressure gradient between the big veins and the atrium, thus determining a decrease of the preload of the right ventricle.
On the other hand, the positive pressure ventilation determined an increase of alveolar pressure and, consequently, an increase of the pressure of the alveolar vessels, determining a subsequent increase of the lung vascular resistance, meaning an increase in the afterload of the right ventricle and in consequence, a decrease of the lung blood flow. Despite an initial increase of the left atrium filling, after two to three cycles, a diminishing of the venous return appears, and, as a consequence, the preload of the left ventricle is reduced.
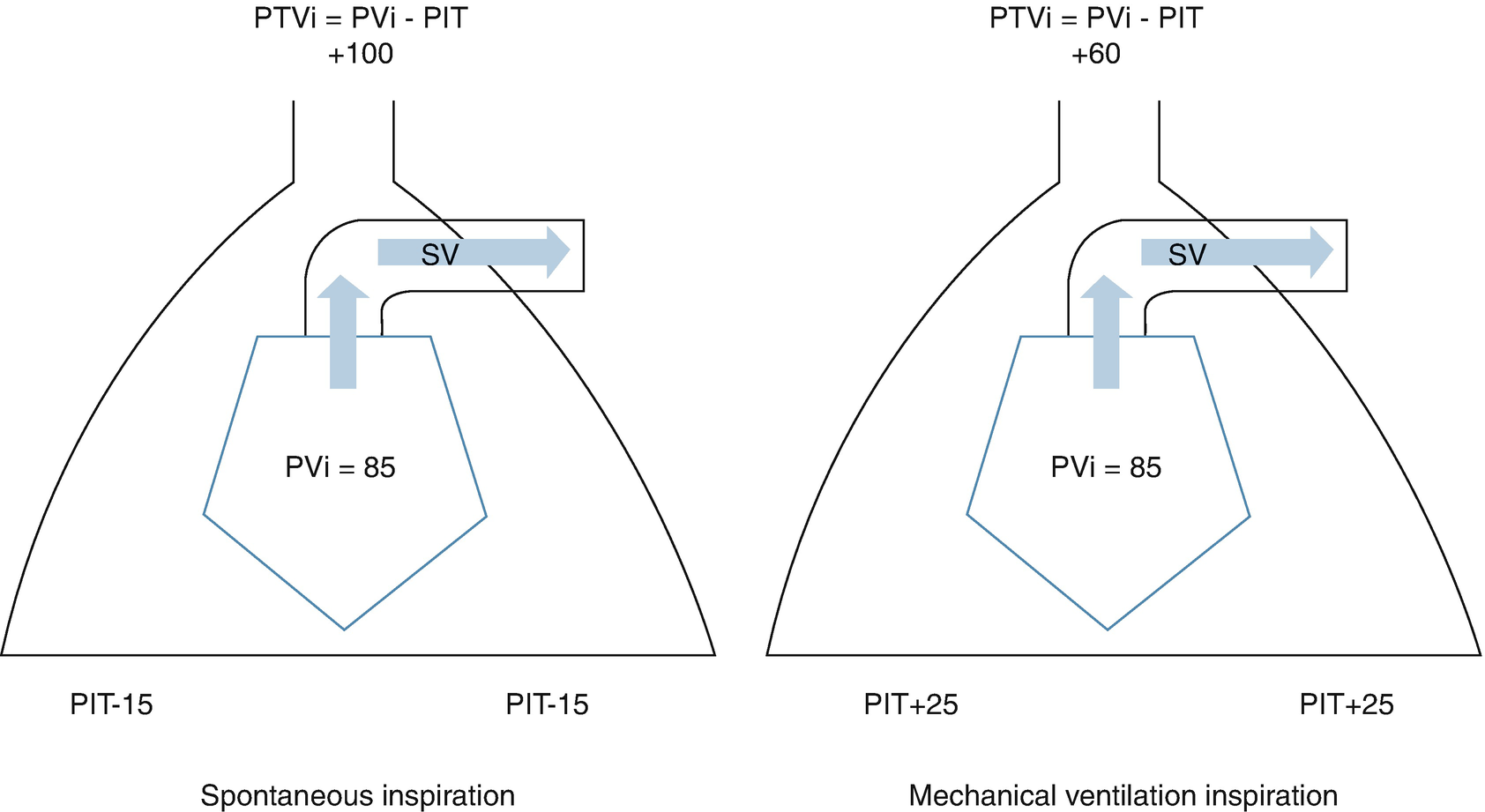
Cardiopulmonary interaction in the left ventricle in positive pressure ventilation. PTVi left ventricular transmural pressure, PVi left ventricle peak pressure, PIT intrathoracic pressure, SV stroke volume
The understanding of cardiopulmonary interactions is essential when considering the mechanical ventilator as another therapeutic tool, for example, in heart failure, the use of invasive mechanical ventilation can be essential when diminishing the left ventricle afterload and improving cardiac output. The same considerations must be taken in patients with severe air trapping or a significant restrictive illness that could imply the use of mPaw that will deteriorate the output and worsen the prognosis of the patient.
Mechanical Ventilation Indications
Indications to initiate mechanical ventilation
Alveolar hypoventilation: PaCO2 > 60 |
Failure in arterial oxygenation (PaO2 < 70 con FiO2 ≥ 60) |
Severe obstructive symptoms |
Apnea or respiratory arrest |
Neuromuscular condition |
Decrease of metabolic consumption: shock |
Cardiogenic shock |
Severe ECT |
Complicated polytrauma |
Substitution of breathing work |
Thoracic wall stabilization |
Surgery, ICU procedures |
The most common cause of mechanical ventilation corresponds to the maintenance of gas exchange in the patient with respiratory failure, because an adequate arterial oxygenation was not achieved (PaO2 < 70 with FiO2 > 60) or an adequate alveolar ventilation was not achieved (PaCO2 > 55–60 in the absence of chronic lung disease). Another indication of mechanical ventilation is in those situations that require a decrease or substitution of the breathing work, either because spontaneous breathing work is ineffective on its own, or because the respiratory system is incapable of performing its function due to a muscular or skeletal failure: scoliosis, trauma, thoracic cavity malformations, neuromuscular diseases, etc.
Decrease of the oxygen consumption (VO2) constitutes another general indication of mechanical ventilation, because in pathological circumstances, oxygen consumption by the respiratory musculature can reach up to 50% of the total consumption. Thus, mechanical ventilation allows an oxygen reserve to be used by other tissues, which can be crucial in certain pathologies, such as septic shock, cardiogenic shock, endocranial hypertension, among others.
Finally, other indications of mechanical ventilation are the stabilization of the thoracic cavity in cases of polytrauma, flail thorax, thoracic surgery, or allowing sedation, analgesia, or muscle relaxation during the postoperative care of complex surgery, or in invasive procedures in critical pediatric care.
Most Used Ventilatory Modes and Initial Parameters of Ventilation in Pediatric Procedures
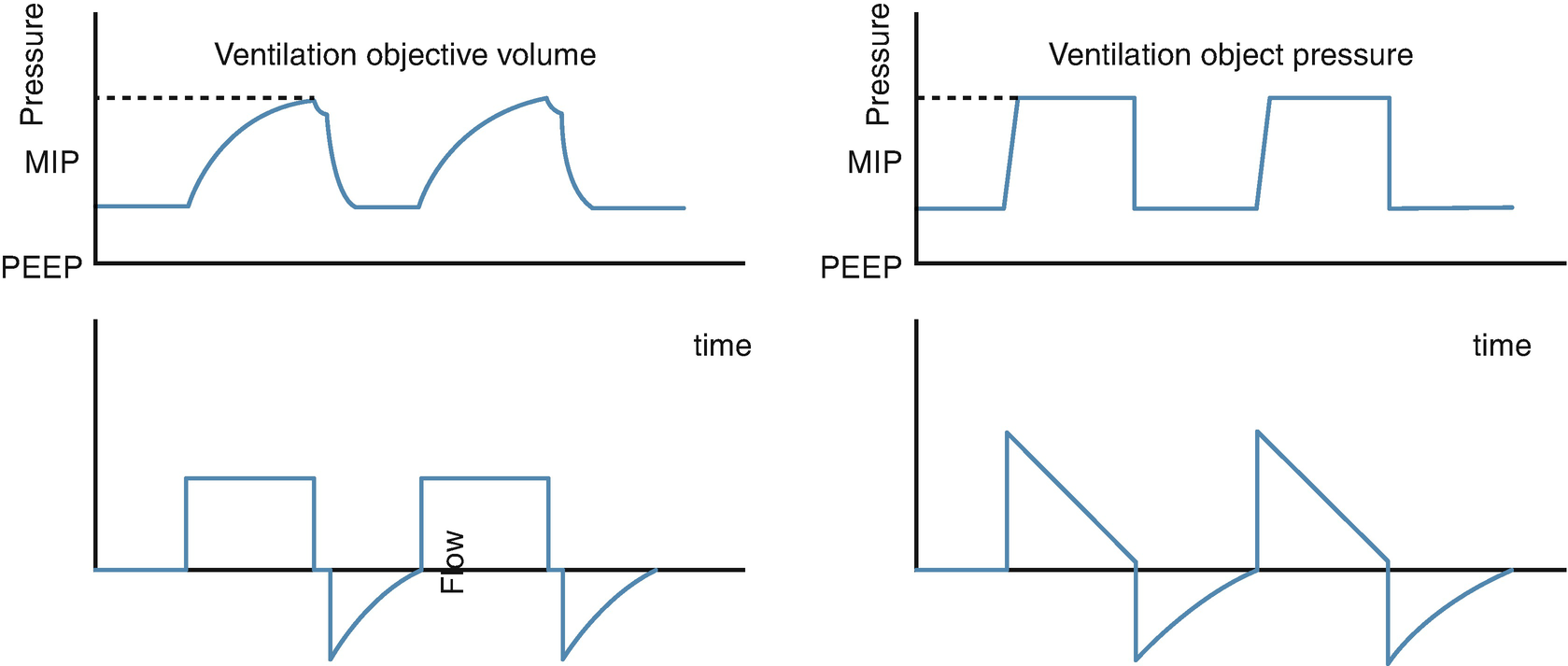
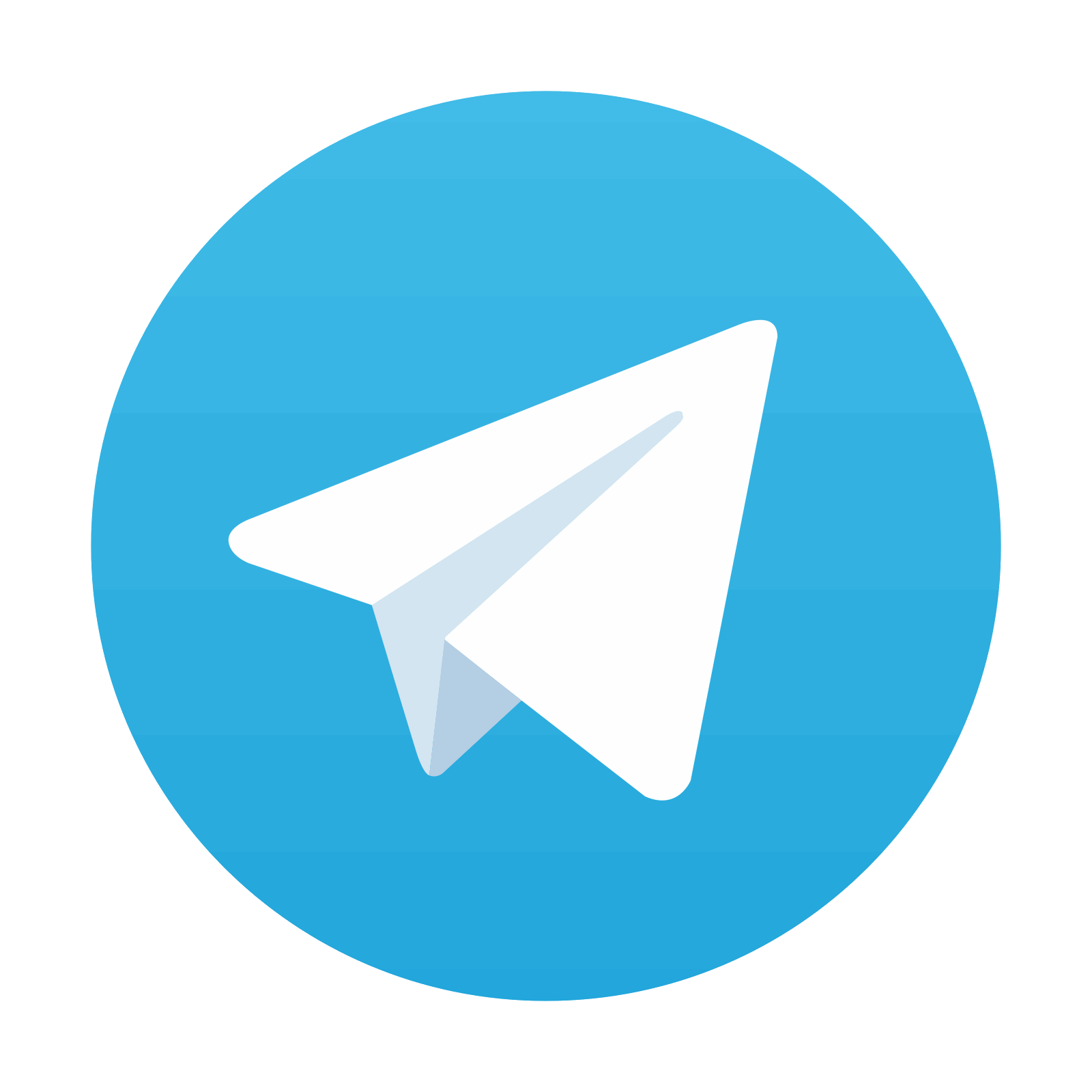
Stay updated, free articles. Join our Telegram channel

Full access? Get Clinical Tree
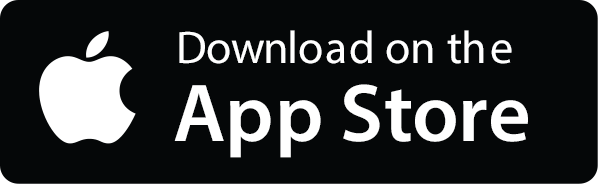
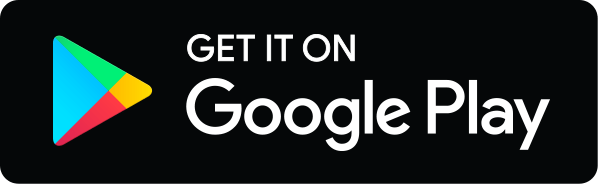