Chapter 18 Management of Acute Trauma
Overview and History
Treatment of the injured patient has been a predominant mission of the surgeon since the origin of medical care. Few other surgical disciplines incorporate such a wide range of skills as those required by the surgeon who is managing severe injury. The treatment of injuries predates recorded history, with evidence of neurosurgical procedures discovered from approximately 10,000 BC. Although the science of improving how injuries are managed progresses continuously, it has been during wartime that many of the greatest advancements were achieved because of the high burden of injury during these relatively short periods. Box 18-1 lists some major contributions to trauma care that were developed during wartime. Common themes include improvements in wound management, resuscitation, and rapid access to care. Recently, the research of trauma care in the military theater has been formalized, which has allowed even greater advances to be achieved.
Box 18-1 Advances and Discoveries in Trauma Care During War
French and Indian War (1754-1763)
American Revolutionary War (1775-1783)
American Civil War (1861-1865)
Iraq wars (Iraq, 2003 to present)
The organization of trauma care has also evolved over the last century as the field matured into a distinct surgical specialty. After the formation of the American College of Surgeons (ACS) in 1913, the leadership of the organization appointed a committee to report on the management of fractures. Created in 1922, the Committee on Fractures evolved to become the Committee on Trauma (COT) in 1949 as the need to formally influence formally how trauma care is provided became evident. Beginning with the publication of the Early Care of the Injured, the COT has been instrumental in advancing trauma care throughout the world via initiatives such as the Advanced Trauma Life Support (ATLS) course, verification of trauma centers, and development of trauma systems that improve access to care. The COT has defined appropriate structure, process, and outcomes, as outlined in Resources for the Optimal Care of the Injured Patient, which is used extensively by trauma centers worldwide.1 The COT also has developed the National Trauma Data Bank (NTDB), which is the largest database of trauma patients in existence, currently including over 3 million patients from 567 trauma centers. Just as the COT was commissioned by the ACS at the national level, it has also formed individual committees on trauma at the state level that work under the direction of the national committee. This structure has proven to be powerful by allowing many of the endeavors that advance the care of the injured patient to occur at the state level, because leadership infrastructure and politics differ regionally. Activities of the state committees frequently include trauma system development with the creation of triage documents, maximizing the use of prehospital and hospital resources, injury prevention initiatives, maintenance of statewide trauma registries, and advancement of performance improvement efforts. Frequently, a major part of this work is the ongoing pursuit of reliable funding mechanisms to pay for the improvement in trauma care throughout the state. Within the infrastructure of the national COT, states are also grouped into regions, which allows for the sharing of information pertaining to successful statewide initiatives and the discussion of issues involving bordering states.
Trauma Systems
Historically, the provision of trauma care was centered around the large academic hospitals that provided the vast majority of injury management. Prehospital efforts focused on getting all patients to the trauma center, regardless of the degree of injury. Although initially found to be beneficial to those patients who were transported to the trauma center, this exclusive type of system failed to address the needs of patients who were geographically distant from the trauma center. Furthermore, this type of system did not capitalize on the resources that could be provided in non–trauma center hospitals. The solution was the development of inclusive trauma systems designed to address the needs of all injured patients, regardless of their severity of injury or geographic location. Inclusive trauma systems capitalize on the resources of all hospitals from critical access facilities to the large levels I and II trauma centers. Guided by predeveloped triage protocols, injured patients are transported to facilities that can provide the level of care necessary to manage injuries of varying severity. At times, this requires transferring patients from smaller hospitals to larger hospitals or trauma centers. Box 18-2 lists the common components of an inclusive trauma system that must be coordinated to maximize the efficiency of getting the injured patient to the care location that he or she needs most. The benefits of this approach include the efficient use of all available resources, reduction in potentially overwhelming trauma centers with patients of lower acuity, and allowing most patients to receive appropriate care within their own community. Finally, it is essential to recognize that legislation plays a large role in the establishment and maintenance of trauma systems. Only through ongoing legislative support can the systematic approach to trauma care grow to eliminate the possibility of a patient lacking access to appropriate, high-quality care for his or her injuries.
Box 18-2 Components of Comprehensive Inclusive Trauma System
Trauma center designation, verification
Post–acute care, rehabilitation
The establishment of trauma systems is a relatively new development, with Illinois and Maryland first creating a system for addressing injuries in the early 1970s. Congress recognized the need for a coordinated approach to the management of injuries and passed the Trauma Care Systems Planning and Development Act of 1990, which formally addressed the need for trauma systems. The development of trauma systems was further advanced with the release of the Model Trauma Care System Plan by the Health Resources and Services Administration (HRSA).2 Originally released in 1992 and then revised in 2006, the newly titled Model Trauma System Planning and Evaluation document applied a public health approach to trauma and provided valuable direction for developing and evaluating trauma systems. This public health approach identified trauma as a disease, the impact of which can be prevented or decreased by applying already established systems that address other health-related issues, such as infectious diseases. Finally, the American College of Surgeons’ COT established the Trauma Systems Consultation Program in 1996 to guide states or regions in the process of developing a systematic approach to trauma care.
The impact of trauma systems on the care people receive after injury has been well studied and provides support for ongoing societal investment in this approach. In 2000, Nathens and colleagues published their evaluation of more than 400,000 patients treated over a 17-year period.3 During the study period, trauma systems were established and developed in many of the regions evaluated. After correcting for all identifiable injury prevention and management improvements, the development of a trauma system over an approximately 10-year period resulted in a reduction in mortality by 8%.3 Several others have also reported this effect, demonstrating an improvement in outcomes in areas that establish a systematic approach to injury management. Most recently, the National Study on Costs and Outcomes of Trauma (NSCOT) was performed to evaluate variations in injury care and outcomes between trauma centers and non–trauma centers. Supported by the National Center for Injury Prevention and Control of the Centers for Disease Control and Prevention (CDC), NSCOT represents one of the largest epidemiologic studies ever to evaluate trauma care; it included more than 5000 patients from 69 hospitals. NSCOT established that injured patients treated at a trauma center experienced improved outcomes over those treated at non–trauma centers. After correction for injury severity, care at a trauma center was associated with a reduction in mortality of 20% in-hospital and 25% at 1 year.4
Injury Scoring
To characterize injuries accurately for the purposes of clinical management, benchmarking, and research, several injury scoring systems have been developed. These typically are based on the anatomy of the injury or the resulting physiology, with several types systems created and evaluated over the last 40 years. One of the first anatomy-based scoring systems was the Abbreviated Injury Scale (AIS), initially published in 1971. The AIS characterizes injuries using a six-digit taxonomy that describes the body region, type of anatomic structure, and specific anatomic detail of the injury. As seen in Table 18-1, the first digit of the AIS score defines the affected body region, allowing clinicians and researchers to identify the location of described injuries quickly. The AIS also assigns a severity code to the injury as a seventh digit, which ranges between 1 (minimal severity) and 6 (presumably fatal). The AIS has been updated six times since the original publication and remains one of the most commonly used injury coding systems.
Table 18-1 Abbreviated Injury Scale (AIS) Body Regions
AIS FIRST DIGIT | BODY REGION |
---|---|
1 | Head |
2 | Face |
3 | Neck |
4 | Thorax |
5 | Abdomen |
6 | Spine |
7 | Upper extremity |
8 | Lower extremity |
9 | Unspecified |
Although the AIS successfully describes individual injuries, it fails to reflect the impact of multiple injuries sustained by the same patient. In 1974, Baker and colleagues5 presented the Injury Severity Score (ISS), calculated by summing the squares of the AIS severity codes for the three most severely injured body regions. Minor injury has been defined as an ISS less than 9, whereas moderate injury is between 9 and 16, serious injury is between 16 and 25, and severe injury is suggested by a score more than 25. The ISS has been used extensively since its development as a way of quantifying the overall injury burden sustained by a given patient. Many other anatomic injury coding systems have since been developed, each with their own merits, although a comprehensive discussion of these exceeds the scope of this chapter. One of the most recent advances in injury coding has been the development of the Organ Injury Scales (OIS) by the American Association for the Surgery of Trauma (AAST), which have been incorporated into the most recent update of the AIS.6 The OIS provides greater anatomic detail to specific organs that was lacking in the AIS. They also have introduced the concept of injury grades, which provide a standard way of describing organ injury severity and the associated risk of morbidity and mortality. Another attribute of the OIS taxonomy is that the severity suggested by the injury grade has been validated using the NTDB.6
Although anatomic scoring methods are more readily used to compare groups of like injuries, physiologic scoring systems may have more real-time clinical value. Physiologic scores provide a better indication of the injured patient’s condition and can therefore be used to make treatment decisions or develop a prognosis. Probably the most commonly used is the Glasgow Coma Scale (GCS), which reflects a patient’s level of consciousness. With scores ranging from 3 to 15, the GCS is composed of a measure of eye opening, verbal response, and motor function. The GCS and more specifically the motor score alone have been found to be reflective of patient outcome after traumatic brain injury.7 Other examples of physiologic scores commonly used are the Trauma Score (TS) and the Revised Trauma Score (RTS), which are composed of the GCS as well as physiologic variables, such as systolic blood pressure, respiratory rate, and capillary refill time to quantify the injured patient’s condition. The GCS and the RTS are depicted in Tables 18-2 and 18-3. These scores have been of value for research purposes and have been used for making triage decisions with some success.
PARAMETER | SCORE |
---|---|
Eye Opening | |
Spontaneous | 4 |
To voice | 3 |
To pain | 2 |
None | 1 |
Verbal Response | |
Oriented | 5 |
Confused | 4 |
Inappropriate | 3 |
Incomprehensible | 2 |
None | 1 |
Motor Response | |
Obeys commands | 6 |
Localizes pain | 5 |
Withdraws to pain | 4 |
Flexion | 3 |
Extension | 2 |
None | 1 |
Total Glasgow Coma Score | 3-15 |
Prehospital Trauma Care
The success of the prehospital trauma management hinges on immediately making a triage and transport decision. Severely injured patients should be immediately transported to an appropriate hospital for definitive care using the “Load and go” philosophy, with all remaining care provided en route. Valuable prehospital care including a head to toe examination, continuous monitoring, placement of subsequent intravenous access, and environmental control can be provided while the patient is being transported. Although the decision to depart the scene rapidly is often simple, where to go and how to get there can be much more challenging. These decisions should be made well in advance and then implemented in the form of well-outlined protocols and agreements that were developed during trauma system planning endeavors. To guide this process, the COT and the CDC have developed a Field Triage Decision Scheme, which is included in the updated COT document1 (Fig. 18-1). This systematic approach to triage uses physiologic status, mechanism of injury, and identification of high-risk patients to assist in deciding who might benefit from immediate transfer to a trauma center. Finally, the value of rapidly making a triage and transport decision within minutes of patient arrival and then quickly departing to keep scene times less than 10 minutes cannot be overemphasized.
One of the primary goals of prehospital trauma care is maintaining control of the injured patient’s airway. The gold standard for airway maintenance in severely injured patients remains oral endotracheal intubation, typically using a rapid-sequence technique with spine stabilization. There has been some controversy recently that has questioned whether advanced airway management in the prehospital setting is more harmful than basic airway support with a bag valve mask and basic airway adjuncts. The existing literature has been unable to address this question adequately. For example, Eckstein and colleagues8 have retrospectively evaluated 496 injured patients and found that endotracheal intubation was associated with a greater mortality compared with bag valve mask support. Studies such as these are limited by selection bias and at best can suggest that this question be studied prospectively. There has also been work specifically in brain-injured patients that argues the converse and thus supports the use of prehospital endotracheal intubation.9 Finally, it is of value to have several airway rescue techniques and methods available because many have been found to facilitate intubation or provide a bridge to a definitive airway. Two examples that are well studied are the gum elastic bougie and blind insertion airway devices.
Resuscitation with isotonic crystalloid solution is initiated in the prehospital phase of care for patients in shock. Although this principle remains well accepted, the need for intravenous (IV) fluid resuscitation in some patient groups has been questioned and the concept of hypotensive resuscitation has been introduced. The rationale is that overresuscitation before management of bleeding could potentially increase the rate of blood loss by disrupting areas that had become hemostatic. Bickell and associates10 have performed a prospective trial that compared standard crystalloid administration with the concept of withholding prehospital fluid resuscitation in patients with penetrating torso trauma. The group of patients who had resuscitation withheld until reaching the hospital had a lower mortality than the immediate resuscitation group. These results are intriguing, but the study represented a unique cohort of penetrating trauma patients in an urban setting, with short transport times to definitive care. Nevertheless, it suggests that prehospital fluid resuscitation be judiciously administered to support some minimal level of perfusion to support the maintenance of hemostasis.
Finally, recent military experience has reintroduced the use of tourniquets for prehospital extremity hemorrhage control. For some time, tourniquets were infrequently used because of concern about muscle and nerve injury. Recent advances in device development and provider education have lessened the associated risk and have again demonstrated the potential benefit of tourniquets in certain situations. Several series now have demonstrated improved outcomes related to the use of tourniquets in the military theater.11 Many prehospital agencies are now including tourniquets on standard equipment lists so that they may be used when confronted with a patient with devastating extremity injuries with uncontrolled arterial hemorrhage. Although many commercial devices are now available, Figure 18-2 illustrates a tourniquet that can be used in the prehospital setting.
Initial Assessment and Management
Since its inception over 30 years ago, the Advanced Trauma Life Support (ATLS) course has presented a safe approach to the initial assessment and management of the injured patient.12 ATLS has been widely adopted as the standard approach in most trauma centers. All physicians who provide initial care to trauma patients should complete the ATLS course to become familiar with the concept of rapidly identifying and addressing life-threatening conditions during the initial patient assessment. Furthermore, ATLS teaches three important concepts that greatly enhance the ability to manage injured patients, regardless of where care is provided:
The initial assessment follows a well-defined order that is based on the patient’s risk of death. At this time, the identification of life-threatening conditions requires immediate intervention. This initial assessment and management, also termed the primary survey, follows the mnemonic ABCDE (Fig. 18-3):
Airway
Several recent developments have broadened the capabilities of airway physicians when challenged with a difficult airway. The gum elastic bougie has been shown to improve the rate of successful intubation, especially in the setting of a challenging airway. For injured patients who cannot undergo cervical extension, require cricoid pressure, or have upper airway injuries, the normal view of the glottis may be obscured. In this setting, the bougie can be placed with a limited view of the vocal cords, resulting in an improved rate of appropriately placing an endotracheal tube. Another rescue technique that should be remembered in the setting of an inability to intubate successfully is the use of a blind insertion airway device. Some commonly used devices include the laryngeal mask airway (LMA), multilumen esophageal airway (Combitube), and laryngeal tube airway (King LT-D). These are typically placed blindly and function essentially by occluding the esophagus and the posterior pharynx, allowing assisted ventilation to pass selectively down the trachea. These have been found to be easy to place and are valuable tools in a rescue situation.13
If a difficult airway requires the intubating physician to progress to a backup plan, preparation for a surgical airway should begin. A cricothyroidotomy can be performed with limited equipment and should commence prior to cardiovascular collapse. The inability to maintain oxygenation with a bag valve mask between intubation attempts is a reasonable indication for establishment of a surgical airway. To perform a cricothyroidotomy (Fig. 18-4), the front portion of the cervical collar is removed and in-line stabilization of the cervical spine is maintained. After preparation, a transverse incision is made over the cricothyroid membrane, which can be palpated between the thyroid cartilage and cricoid ring. Spreading the overlying soft tissue reveals the cricothyroid membrane. The membrane is transversely incised and the cricothyroidotomy is spread longitudinally. A tracheostomy or endotracheal tube is then advanced through the incised membrane and down the trachea. After balloon inflation, tube position is immediately confirmed with lung auscultation and end-tidal carbon dioxide determination. Finally, patients suspected of having a laryngeal injury might have involvement of the airway in the vicinity of the cricothyroid membrane and therefore could benefit from a tracheostomy instead of a cricothyroidotomy.
Circulation
After respiratory stabilization, an immediate assessment for cardiovascular compromise is completed. Simply, the physician must determine whether the injured patient is in shock. Box 18-3 lists the most common immediate indicators of shock. It is important to recognize that a patient can be in shock before developing hypotension because this is one of the last findings before complete cardiovascular collapse. Cardiovascular dysfunction in injured patients is secondary to hemorrhage in most patients. In less common situations, a spinal cord injury (neurogenic shock), or preinjury heart failure or sepsis could be the cause. On recognition of shock, resuscitation is immediately initiated with 1 to 2 liters of warm crystalloid solution infused through two large-bore, short, peripheral IV catheters. A rapid assessment for the source(s) of blood loss is then completed. It may be valuable to approach this assessment by recognizing the five major locations for exsanguinating blood loss: chest, abdomen, retroperitoneum (often a pelvic fracture), multiple long bone fractures, and external sites. Immediately, a brief physical examination will identify long bone fractures and sources of external hemorrhage. A chest radiograph will evaluate for thoracic blood loss and a pelvic radiograph will identify a pelvic fracture. The focused abdominal sonography in trauma (FAST) scan or diagnostic peritoneal lavage (DPL) for gross blood can be obtained to evaluate for intra-abdominal bleeding. The FAST scan is a rapidly obtainable ultrasound that assesses for fluid within the abdomen. The FAST scan assesses the hepatorenal, splenorenal, and pelvic spaces for fluid which in the setting of trauma most likely represents blood. A FAST scan can be performed quickly in the trauma bay by the surgeon and can be rapidly repeated if necessary. Figure 18-5 demonstrates blood in the hepatorenal space on FAST scan.
Resuscitative Thoracotomy
Rarely, select patients who experience cardiac arrest in the emergency department may be candidates for a resuscitative thoracotomy. There are four main goals of a thoracotomy in the emergency department: opening of the pericardium to relieve cardiac tamponade, internal cardiac massage, cross-clamping of the distal thoracic aorta, and management of intra-thoracic bleeding. Although potentially lifesaving for some patients, a resuscitative thoracotomy can be dangerous for providers and costly to perform. Therefore, studies have attempted to identify patient groups that have a chance of survival following resuscitative thoracotomy to establish when the procedure is indicated. Patients who have demonstrated more favorable results are those with penetrating thoracic injuries who have signs of life on reaching the emergency department. An assessment of all applicable studies yielded a survival rate of 11.2% after resuscitative thoracotomy for penetrating chest injury.14 Blunt trauma patients have uniformly dismal results and therefore are not candidates, except in the most select situations. Survival rates of 1.6% were noted when appropriate studies were pooled, and most of these survivors had a poor neurologic outcome.14 Patients with stab wounds demonstrate better outcomes than gunshot wounds because of the higher incidence of pericardial tamponade without major cardiac injury which may be more likely to respond to pericardial decompression. Signs of life suggesting potential response to resuscitative thoracotomy include pupillary reactivity, spontaneous respiratory effort, palpable pulses, extremity movement, and cardiac electrical activity. Performing a resuscitative thoracotomy mandates having surgical support personnel who can perform definitive repair of thoracic injuries if spontaneous circulation is successfully resumed.
Management of Specific Injuries
Damage Control Principles
Historically, in many centers, trauma patients who required immediate operation for life-threatening injuries would undergo surgery until all injuries were definitively repaired. Some patients would experience progressive physiologic derangement during these operations, often developing hypothermia, coagulopathy, and metabolic acidosis, a combination that has become labeled as the deadly triad or the bloody vicious cycle. To avoid certain death, this cycle must be halted by quickly managing bleeding and providing aggressive resuscitation. In 1993, Rotondo and associates15 coined the term damage control to describe an approach to managing patients who were progressing rapidly toward death as their injuries were being definitively repaired. Damage control includes immediate surgical control of hemorrhage and contamination without immediate definitive reconstruction. All surgical bleeding is controlled and intra-abdominal or intrathoracic packing is frequently required to achieve hemostasis. Hollow visceral resection with temporary GI discontinuity is also commonly used. The chest or abdomen is then temporarily closed, frequently using a vacuum-type closure method. The patient is aggressively resuscitated in the intensive care unit with the goal of achieving adequate cardiopulmonary function and metabolic hemostasis. On improvement in body temperature, coagulopathy, and acidosis, definitive reconstruction of injuries can occur, followed by chest or abdominal closure when feasible. Initially, the concept of damage control was used for severe abdominal injuries but the principles have been applied to injuries of the chest, pelvis, and extremities. Using a similar approach, some have even advocated damage control orthopedics, which is based on the theory that rapid fracture stabilization may reduce the inflammatory response to injury and result in fewer long-term sequelae.
Recently, traditional methods of resuscitation immediately after injury have been questioned. Born out of military experience, an approach now called damage control resuscitation has evolved, with promising early results. In damage control resuscitation, replacement of lost blood is provided as a more equivalent distribution of all blood components. The military experience found that resuscitating with equal amounts of packed red blood cells, plasma, platelets, and cryoprecipitate resulted in preventing severe coagulopathy, which was associated with less physiologic derangement after severe injury.16 Although some studies have reported improved outcomes with this approach, others have questioned the validity of these findings when applied to civilian patient populations. Nevertheless, many trauma centers have implemented this approach. Prospective trials are greatly needed to definitively evaluate this practice.
Injuries to the Brain
Traumatic brain injuries (TBIs) remain one of the greatest causes of death and disability despite significant improvements in the care of these injuries over the last several decades. Injuries to the brain are common with a substantial percentage resulting in death or permanent disability. Commonly, the outcome of a patient with multiple injuries is dictated by the impact of the brain injury. According to data from the CDC, 1.4 million brain injuries are sustained each year, with 1.1 million evaluated in U.S. emergency departments.17 Falls are the most common cause of brain injuries, with those at the extremes of age being the most vulnerable to this mechanism. The mortality rate for the 235,000 patients with TBI that require hospitalization is 21.3% and the number of people experiencing permanent TBI-related disability exceeds 80,000 each year.
Mechanism and Pathophysiology
Injuries to the brain result from direct transmission of energy to the cranium and the underlying brain tissue, as well as damage resulting from movement of the brain within the rigid cranial vault. Compression of brain tissue can also result from tearing of intracranial blood vessels, which causes accumulation of blood. Secondary brain injury can occur following the initial insult and results from ischemia and compression by adjacent tissue edema. Because of the rigidity of the bony cranium, the volume within the skull remains constant. The Monro-Kellie doctrine states that any increase in the volume of intracranial contents results in an elevation of intracranial pressure with an associated decrease in the volume of other tissues, such as the brain parenchyma and cerebrospinal fluid. Figure 18-6 depicts the relationship between intracranial volume and pressure, which explains why injury resulting in increased intracranial blood and edema can have such a detrimental effect on the surrounding brain tissue. Epidural hematomas (Fig. 18-7) typically result from a lateral fracture of the cranium causing bleeding from the middle meningeal artery or a nearby vessel. The classic clinical course includes a brief loss of consciousness followed by a lucid interval, during which time the hematoma is expanding. Ultimately, symptoms again develop and can be profound without intervention. When identified and treated early, patients with epidural hematomas can have favorable outcomes because the hematoma itself is usually not associated with underlying brain parenchymal injury. This is in distinction to subdural hematomas, which commonly are associated with severe underlying brain tissue injury (Fig. 18-8). Subdural hematomas are believed to result from tearing of the bridging veins between the dura and cerebral cortex. The hematoma can be compressive but it is frequently the underlying brain contusion and axonal injury that dictate the outcome after these injuries. Subarachnoid hemorrhage after TBI is common and in itself has little deleterious effect. The presence of blood in the subarachnoid space likely is a reflection of the presence of a TBI, which should prompt surveillance. Parenchymal contusions of brain tissue result from a direct blow to the cranium or from movement of the brain within the rigid cranial vault, resulting in injury on the opposite side, also described as a contra-coup injury. Typically, the blood and hematoma associated with these contusions are not overly large but the edema that develops over the subsequent days can be profound and a major source of secondary brain injury. Finally, diffuse axonal injury describes the phenomenon of disruption of the axon from the neuronal body secondary to severe rotational forces that are believed to create a shearing effect. Frequently, the magnitude of this type of injury cannot be appreciated on imaging and the ultimate severity is determined clinically during the weeks that follow. Diffuse axonal injury may be suggested on imaging by the presence of scattered punctuate hemorrhages within the parenchyma and, at times, a loss of the differentiation of gray and white mater.
Management
Most patients with intracranial hemorrhage require close monitoring of neurologic function and vital signs, which is usually best performed in a higher level of care setting, such as the intensive care unit. Guidelines published by the Brain Trauma Foundation provide an excellent assessment of the literature and represent the most comprehensive, evidence-based recommendations available.18 Secondary brain injury should be prevented by ensuring adequate cardiovascular and pulmonary function. Many patients with severe TBI require measurement of the intracranial pressure (ICP) to guide management, which is aimed at reducing associated brain tissue edema. Cerebral perfusion pressure (CPP), which is the difference between the mean arterial pressure and the ICP, is also commonly used to guide severe TBI management. Although many physicians preferentially use ICP or CPP to direct management, it has been determined that neither one is superior to the other. It has been recognized that the overaggressive treatment of CPP may be deleterious. Figure 18-9 demonstrates an approach to the management of the patient with a severe TBI.
Injuries to the Spinal Cord and Vertebral Column
Injuries to the spinal cord can occur after blunt or penetrating mechanisms. Blunt trauma to the spine can result in cord injury through direct impingement or indirect manipulation. Fractures and dislocations can reduce the size of the spinal canal and cause direct tissue damage or secondary injury through ischemia, bleeding, or edema. The spinal cord can also sustain injury through mechanisms that distract or severely rotate the cord, causing neuronal damage. Penetrating mechanisms directly lacerate the spinal cord tissue or cause adjacent injury and indirect damage. Occasionally, injury to the spinal cord can occur without abnormality of the vertebral column identified on imaging. The phenomenon known as spinal cord injury without radiographic abnormality (SCIWORA) can be extremely frustrating because the lack of bony injury can result in missed opportunities to prevent neurologic injury. Fractures of the vertebral column can occur after almost any form of physical force. Common mechanisms include flexion and extension, especially in the cervical spine, as well as compressive forces that commonly affect the lumbar spine. A Chance fracture is a well-described pattern with transverse disruption through all vertebral elements that occurs most commonly during an MVC. During a high-speed frontal crash, an occupant wearing a seatbelt above the iliac crest experiences flexion and distraction of a lumbar vertebrae, resulting in this fracture pattern (Fig. 18-10).
Evaluation
Further evaluation of the spine typically involves CT of the cervical, thoracic, and lumbar vertebral bodies. Although plain radiographs of the spine are acceptable, the high-quality images and rapid availability associated with CT have made this the modality of choice in most emergency departments. Visualization of the cervicothoracic junction on plain radiographs can be extremely challenging, especially in larger patients, and can often require numerous repeat studies. For this reason, many have transitioned to obtaining a dedicated cervical spine CT during the initial imaging of the patient. CT also provides the ability to reconstruct the images into sagittal and coronal planes to provide even better anatomic visualization. SCIs are less well delineated on CT than bony injuries but are suggested by the presence of spinal canal compromise and soft tissue edema identified adjacent to the spinal cord. Figure 18-11 demonstrates a severe cervical spine fracture with subluxation and anterior displacement.
Management
As noted, the spine requires protection with strict immobilization throughout the entire evaluation until injuries can be ruled out. Typically, this includes the use of a hard collar and maintenance of the log roll technique when movement is required. Although a long spine board is generally used during the transport of patients in an ambulance, it is important to remove it as soon as possible to prevent the development of pressure wounds, which can develop quickly when a patient is lying on a rigid device. On recognizing the presence of a SCI, a spine surgeon consultation should be obtained promptly. In facilities that have no spine surgery services available, arrangements for transfer should begin immediately. Further studies and interventions should only occur if the results will have an immediate impact on the care provided. For example, imaging to identify an associated vertebral column fracture will have no effect on care if a spine surgeon is not available and therefore transfer should not be delayed. SCIs with neurogenic shock, occurring most commonly with cervical injuries, require resuscitation because of a loss of sympathetic tone. Neurogenic shock frequently responds to volume expansion with crystalloid solution but occasionally requires vasopressor agents such as dopamine or epinephrine. Hypotension should be avoided because it may contribute to cord ischemia and progression of the SCI. The value of corticosteroid administration has been extensively studied but remains controversial. Several large randomized trials have demonstrated small improvements in recovery after methylprednisolone administration, especially when initiated early after injury.19 Others investigators have been unable to reproduce these results and have found an increased incidence of steroid-related complications with methylprednisolone therapy. Therefore, most authors agree that steroids remain an option that should be considered after consultation with the spine surgeon. When administered, methylprednisolone is provided as a bolus of 30 mg/kg body weight followed by an infusion of 5.4 mg/kg/hr for 23 hours if the bolus was given within 3 hours of injury. The infusion duration is extended to 48 hours if the bolus was administered between 3 and 8 hours after injury whereas SCIs that occurred more than 8 hours prior should not be treated.
Cervical fracture-dislocation injuries may benefit from the application of traction in the emergency department to restore vertebral column alignment. Based on the injury pattern and associated injuries, some SCIs benefit from early operative decompression to reduce cord impingement, as determined by the spine surgeon. Other injuries may require fixation because of instability on a semielective basis after immediate patient care needs are addressed. Fractures without instability may require only immobilization with a hard collar or brace over a several-week time period. Table 18-4 lists commonly encountered vertebral column fractures with management options. It is important that any patient with an SCI or significant vertebral column injury be monitored closely for changes in neurologic examination that might prompt urgent intervention.
Table 18-4 Fractures of the Vertebral Column
FRACTURE | DESCRIPTION | TYPICAL MANAGEMENT |
---|---|---|
C1 Jefferson fracture | Disruption of C1 ring in multiple locations; blow-out of ring | Stable transverse ligament: Hard collar |
Unstable transverse ligament: Traction or surgery | ||
Odontoid fractures | Type I: Tip of odontoid | Type I: Hard collar |
Type II: Through base | Type II: Halo vest or surgery | |
Type III: Involves C2 body | Type III: Halo vest | |
C2 hangman fracture | Bilateral C2 pedicles with spondylolisthesis | Halo vest or surgery if displacement severe |
Cervical vertebral body fractures | Compression or burst of vertebral body, with or without retropulsion into canal | Mild loss of height: Hard collar |
Involvement of multiple columns or presence of retropulsion into canal: | ||
Surgical stabilization | ||
Thoracic vertebral body fractures | Compression or burst of vertebral body, with or without retropulsion into canal | Anterior column only: TLSO |
Anterior and posterior columns: Surgical stabilization | ||
Lumbar vertebral body fractures | Compression or burst of vertebral body, with or without retropulsion into canal | Anterior column only: TLSO |
Anterior and posterior columns: Surgical stabilization | ||
Chance fracture | Avulsion of posterior elements of lumbar vertebrae seen with high seatbelt use | Surgical stabilization |
TLSO, Thoracolumbosacral orthosis.
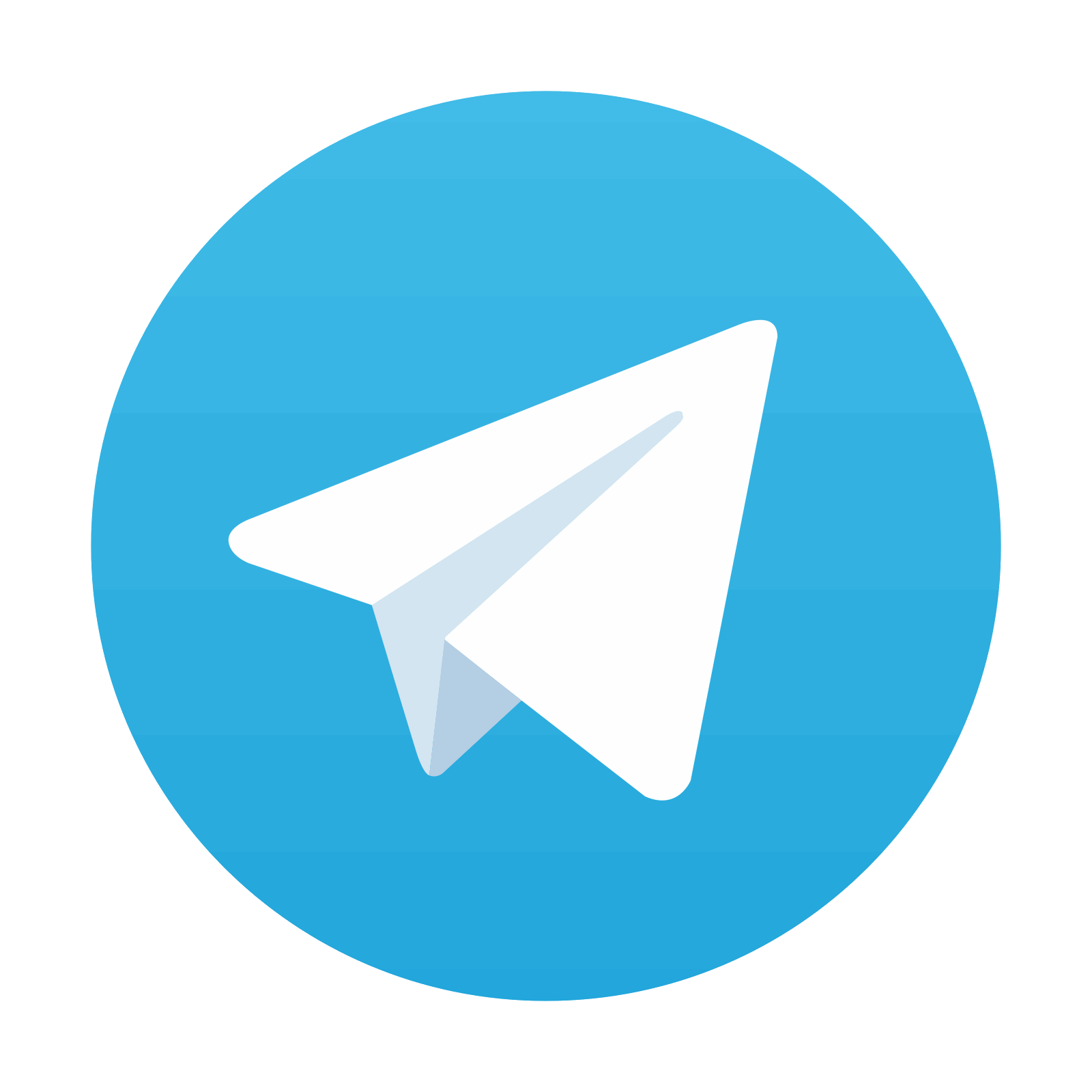
Stay updated, free articles. Join our Telegram channel

Full access? Get Clinical Tree
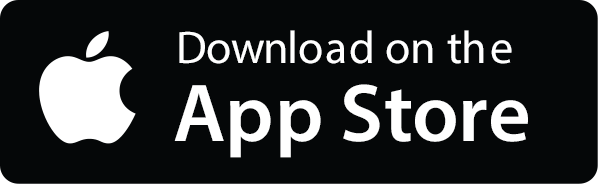
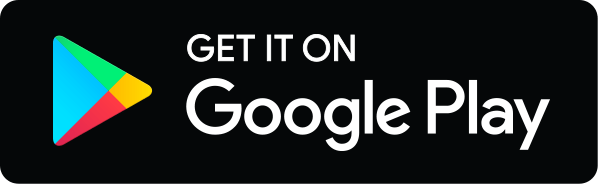