Abstract
Lung injury from hydrocarbon aspiration continues to account for a significant proportion of accidental poisonings in children. Severe pneumonitis can occur. Treatment remains largely supportive, and some suggest that early administration of intratracheal surfactant may be beneficial. Prevention is key to reducing its occurrence. Significant morbidity and mortality from smoke inhalation occurs in victims of fire. In addition, lung injury can be caused by chemical and thermal insults. A variety of noxious gases, irritants, and asphyxiants are generated depending on the material burnt. Carbon monoxide is the predominant cause of death among fire victims. Treatment should be directed at reversing hypoxemia as a result of asphyxia or carbon monoxide poisoning. However, there is no evidence that the routine use of corticosteroids or prophylactic antibiotics is beneficial. The role of hyperbaric oxygen therapy remains controversial. Treatment is generally supportive. Prevention is paramount in reducing fire-related injuries.
Keywords
hydrocarbon aspiration, smoke inhalation, lung injury
Epidemiology
In 2014, in the United States, 31,903 total hydrocarbon exposures were reported to poison control centers, and 9546 of these occurred in children less than 5 years old. There were 118,207 exposures to household cleaning substances in children less than 5 years old, resulting in the third leading cause of poisoning deaths in this age group in the United States. The highest morbidity and mortality result from accidental ingestion in children less than 5 years of age.
Etiology
Hydrocarbon toxicity resulting from the ingestion of petroleum solvents, dry-cleaning fluids, lighter fluids, kerosene, gasoline, and liquid polishes and waxes (mineral seal oil) continues to be a common occurrence in small children. This is in addition to the potential harm from additives such as heavy metals, pesticides, and camphor, all of which have potential systemic toxicity. Kerosene heaters, cleaning fluids, furniture polishes, and liquid floor waxes are present in homes, too frequently within the easy reach of toddlers, and they account for the persistence of hydrocarbon poisoning. In one series of hydrocarbon ingestions, the material was stored in a standard beverage container almost one-third of the time. The most commonly ingested materials are household cleaning products, solvents, and fuels; in some areas, kerosene ingestion is more common. In 2001, the US Consumer Product Safety Commission required child-resistant packaging for products with low viscosity and a significant concentration of hydrocarbons (>10% by weight). Similar attempts were made by the European Union in 1997. Unfortunately, these regulations do not seem to have had a significant impact on the frequency of such poisonings.
Although central nervous system (CNS) abnormalities (e.g., weakness, confusion, and coma), gastrointestinal irritation, cardiomyopathy, and renal toxicity all occur, the most common and the most serious complication is pneumonitis. Deaths from hydrocarbon poisoning are almost always from respiratory insufficiency.
Pathology
Lung pathology in fatal cases includes necrosis of bronchial, bronchiolar, and alveolar tissue, atelectasis, interstitial inflammation, hemorrhagic pulmonary edema, vascular thromboses, necrotizing bronchopneumonia, and hyaline membrane formation.
Experimental studies in rats reveal an acute alveolitis that is most severe at 3 days, subsides at 10 days, and is followed by a chronic proliferative phase that may take weeks to resolve. Rats develop hyperemia and vascular engorgement of both large and small blood vessels within 1 hour of aspiration. At 24 hours, there is a focal bronchopneumonia with microabscess formation. By 2 weeks, the process largely resolves, although some vascular engorgement and rare peribronchial inflammation persist. In humans, chest roentgenographic abnormalities persist for some time after physical findings have cleared, suggesting a similar prolonged recovery.
Dogs show different responses, which also vary with the amount of kerosene ingested. A low dose causes destruction of alveolar epithelium, resulting in emphysema but insignificant airway involvement. More intense airway obstruction is seen in the high-dose group. At 1 hour after high-dose ingestion, intraalveolar edema and hemorrhage occur most prominently in the subpleural air spaces and those adjacent to large bronchi, suggesting induced interstitial inflammation. By 24 hours after ingestion, many airways show severe destruction and desquamation of the lining epithelium, resulting in near complete obliteration of the airways. The lumens are filled with degenerating neutrophils and scattered macrophages and lymphocytes. The surrounding lung parenchyma and the interstitia of the blood vessels show intense infiltration by macrophages and neutrophils, with microabscesses in the lung parenchyma. While most of the findings heal within a week, scattered areas of bronchiolitis obliterans may persist. By 2 weeks, much of the lung tissue has returned to normal, with rare areas of bronchiolitis obliterans. The severity of these dose-related changes can be explained by two characteristics of kerosene. First, it is a solvent that can dissolve cell walls and/or lipid lining layers, resulting in the desquamation of cells and fluid influx into the airways. Second, as a foreign substance that can penetrate the interstitium, it can provoke an intense inflammatory reaction. Since there are dose-related responses to kerosene with interspecies differences, accurate prediction of the process in humans is difficult.
Pathophysiology
The risk for aspiration varies with the inherent properties of the hydrocarbon. Aspiration is much more likely to occur with a substance that has low surface tension, low viscosity, and high volatility, and low surface tension allows the substance to spread throughout the tracheobronchial tree. Low viscosity allows the substance to spread more readily and to seep deeper into the tissues. High volatility increases the likelihood of CNS involvement, presumably due to more rapid absorption into the bloodstream; highly volatile substances may also rapidly spread to the alveoli and immediately interfere with gas exchange.
Hydrocarbons increase surface tension by inhibiting surfactant, which predisposes to alveolar instability and atelectasis. The instillation of artificial surfactant into the trachea of sheep following kerosene exposure resulted in significant improvement in oxygenation and mortality. There is one case report of a 17-month-old child with ARDS from hydrocarbon aspiration who survived after treatment with surfactant.
Pulmonary lesions are believed to be caused by direct aspiration of the hydrocarbon into the airways. This may occur either during the initial ingestion or during subsequent emesis. Small amounts of aspirated hydrocarbons may produce more serious disease than larger amounts in the stomach. Evidence that hydrocarbons are removed by the first capillary bed they encounter reinforces the notion that pulmonary damage occurs from aspiration. Indeed, the liver and the lungs filter out sufficient amounts of kerosene to help prevent CNS damage. Fatalities are rarely attributed to CNS involvement per se.
Clinical Findings
Cough may appear within 30 minutes of aspiration or may be delayed for hours. Initially, auscultation of the chest may be normal or may reveal only coarse or decreased breath sounds. When severe injury occurs, hemoptysis and pulmonary edema develop rapidly, and respiratory failure may occur within 24 hours. Radiographic signs of chemical pneumonitis, when present, will develop within 2 hours after ingestion in 88% of cases and within 6–12 hours in 98%. The findings vary from punctate, mottled densities to pneumonitis or atelectasis and tend to predominate in dependent portions of the lung ( Fig. 40.1A ). Air trapping, pneumatoceles, (see Fig. 40.1B ), and pleural effusions may also develop. The radiographic abnormalities reach their peak within 72 hours and then usually clear within days. A review of 16 children showed that children whose chest radiographs were to become abnormal did so by 24 hours after the ingestion, and most cleared within 2–3 weeks.

Occasionally, the radiographic findings persist for several weeks. There is a poor correlation between clinical symptoms, physical findings, and radiographic abnormalities. In general, the radiographic changes are more prominent than the findings on physical examination and persist for a longer period. When pneumatoceles occur, they are likely to do so after a patient has become asymptomatic. They require several months for spontaneous resolution.
Blood gas studies reveal hypoxemia without hypercapnia, suggesting ventilation-perfusion mismatch or diffusion block. Destruction of the epithelium of the airways together with bronchospasm adds to the ventilation-perfusion abnormalities, and displacement of alveolar gas by the hydrocarbon vapors adds to the hypoxemia.
Management and Treatment
Initial management should comprise a history, physical examination, and chest radiograph. Because hydrocarbons do less damage when swallowed than when aspirated into the lungs, it is important to avoid emetics or gastric lavage. Some toxicologists recommend nasogastric lavage through a large bore tube in patients with a large amount of hydrocarbon with the potential for systemic toxicity if the patient presents within 1 hour of ingestion. If the child had no symptoms at the scene or in the emergency room and has a normal chest radiograph, observation for 6–8 hours is important. If no symptoms develop, the child may be safely discharged home. If an abnormality is detected by history, examination, or radiography, arterial blood gas analysis should be performed. Even if no symptoms develop, a repeat chest radiograph at 24 hours is a prudent measure. If results of examination and radiography are normal at 24 hours and the child has no further symptoms, discharge can occur after providing education and reassurance. Supplemental oxygen must be given if the child is hypoxemic. Adequate hydration should be maintained, but excessive fluid administration may be counterproductive, as the pulmonary pathology evolves. Case reports of rapid recovery of lung function and beneficial effects of lung mechanics and gas exchange were noted after early administration of intratracheal surfactant as early as initial presentation to the emergency room. In animal studies, nebulized β2-agonist administered to sheep with combined smoke inhalation and burn injury resulted in improved lung function, airway clearance, and reduced pulmonary edema. Although clinical data in humans is lacking on the use of β2-agonist in inhalation injury, some have suggested a trial of bronchodilators if respiratory symptoms progress. Hypoxemic respiratory failure should be treated with mechanical ventilation and positive end-expiratory pressure. If the child fails conventional mechanical ventilation, both extracorporeal membrane oxygenation (ECMO) and high-frequency jet ventilation have been successful in improving oxygenation and allowing survival.
Although superimposed bacterial infection is a potential concern, there is no evidence that this is a common occurrence. Because leukocytosis and fever are common after hydrocarbon aspiration, it is often difficult to detect bacterial superinfection. One thoughtful review concluded that bacterial complications are rare. Until there is further evidence to the contrary, antimicrobial therapy should be reserved for patients who are severely compromised by malnutrition, debilitation, or an underlying disease or in whom the pneumonia is especially severe. Evolving evidence of infection on serial Gram stains of secretions from the endotracheal tube could be an indication for antimicrobials. Because airway closure and collapse are a significant part of the disease, continuous distending airway pressure is desirable to maintain functional residual capacity (FRC) and to keep the concentration of inspired oxygen in safe ranges. Studies in animals and humans show no therapeutic or prophylactic role for corticosteroids.
Prevention
Prevention of the accidental ingestion of products containing hydrocarbons must be a high priority. Educating parents to keep potentially toxic materials out of the reach of young children seems obvious. Education about storage of such materials and avoiding containers that children associate with potable liquids must be stressed. Prevention of nonintentional poisonings includes clearly labeling containers that contain hydrocarbons. If kerosene heaters are used in the home, the kerosene must be kept out of the reach of children.
Prognosis
Prognosis and clinical improvement depends on the type, volume, and pH of the substance aspirated. Long-term follow-up studies of pulmonary function in patients with hydrocarbon pneumonitis indicate residual injury to the peripheral airways. A study of 17 asymptomatic children 8–14 years after a hydrocarbon pneumonitis showed abnormal lung function in 14 (82%). The most common abnormalities were an elevated ratio of residual volume to total lung capacity, an increased slope of phase III, reduced forced expiratory volume in 1 second (FEV 1 ) and a high volume of isoflow ; these results indicate small airway obstruction and gas trapping. When radiographic changes accompany the ingestion of hydrocarbons, this same pattern of abnormal lung function is detected 10 years later, even in otherwise asymptomatic subjects. However, the frequency of airway reactivity appears to be normal. Most children survive without serious complications, but some may progress to respiratory failure and death.
Hydrocarbon “Sniffing”
Deliberate inhalation of volatile hydrocarbons to induce a state of euphoria is common among adolescents. It is estimated that 13% of adolescents have used inhalants at one time or another. Unlike other forms of drug abuse, this is more common among those in the seventh to ninth grades. The euphoria of mild intoxication may be accompanied by mild nausea and vomiting. Prolonged exposure may lead to violent excitement followed by CNS depression, unconsciousness, and coma. Large doses of halogenated hydrocarbons, especially when combined with exertion, excitement, and hypercapnia, may be associated with dysrhythmias and death. Medullary depression and respiratory paralysis are generally accepted as the mechanism of death in most gasoline inhalation fatalities. Strong psychological dependence may develop in some sniffers. Acute hypoxemia at the time of inhalation and shortly thereafter is not uncommon as a result of displacement of alveolar gas by the inhaled substance. However, this is usually transient and not significant. Lung injury per se from hydrocarbon sniffing has not been described. A toluene embryopathy has been described in infants born to mothers who sniffed toluene, although the “polypharmacy” often used by the mothers may confound the data.
Respiratory Complications of Smoke Inhalation
Epidemiology
In 2014, there were 494,000 structural fires in the United States; of these, 386,500 were residential structural fires. Death from fire is the seventh leading cause of unintentional injury death in the United States in 2014. The United States ranks 10th out of 24 developed countries in fire deaths. Those at greatest risk are children younger than 4 years of age; African-American and Native American males; those over 85 years of age, and the poor. In 2014, 3275 civilians died in fires in the United States. A great deal of the morbidity and mortality in victims of fires results from pulmonary injuries due to smoke inhalation. The severity of the lung injury depends on (1) the nature of the material involved in the fire and the products of incomplete combustion that are generated and (2) whether the victim has been confined in a closed space. The subject’s minute ventilation also plays a role. The deeper and more rapidly the victim breathes, the greater the amount and degree of deposition of toxic materials into the airways and alveoli. The decreased inspired oxygen concentration that results from the fire stimulates compensatory hyperapnea in humans.
Etiology
Fire-related injuries and death occur as a result of structural and outside fires. Isolated smoke inhalation injury can occur but may be complicated by thermal or chemical burns to the airways.
Pathogenesis
The pathogenesis of lung injury from smoke inhalation includes thermal and chemical factors. Because the upper airway is such an effective heat exchanger, most of the heat from inhaled smoke is dissipated before the inhaled material reaches the carina. Direct thermal damage, therefore, primarily affects the supraglottic airways. Only with steam, which is unusual in most fires, or with prolonged exposure to high ambient temperatures, will there be thermal injury to the intrathoracic airways.
Depending on the material involved in fires, a wide variety of noxious gases may be generated. These include the oxides of sulfur and nitrogen, acetaldehydes, hydrocyanic acid, and carbon monoxide (CO). Irritant gases such as nitrous oxide or sulfur dioxide may combine with lung water to form corrosive acids. Aldehydes from the combustion of furniture and cotton materials induce denaturation of protein, cellular damage, and pulmonary edema. The combustion of wood generates considerable quantities of CO and carbon dioxide. Plastics, if heated to sufficiently high temperatures, may be the source of very toxic vapors. Thus, chlorine and hydrochloric acid may be generated from polyvinyl chloride; hydrocarbons, aldehydes, ketones, and acids from polyethylene; and isocyanate and hydrogen cyanide from polyurethane. Although the particulate matter carried in the smoke (soot) probably does not in itself produce injury, toxic gases may be absorbed on the surface of the carbon particles and carried into the lungs; the soot particles may also be responsible for inducing reflex bronchoconstriction.
Carbon Monoxide Poisoning
CO poisoning is an especially serious complication of smoke inhalation that occurs soon after exposure. While smoke inhalation is the most common cause of inadvertent CO poisoning, other causes include poorly functioning home heating systems; inadequate ventilation for fuel burning systems (e.g., gas grills, kerosene heaters, and camp stoves); and motor vehicles idling in poorly ventilated areas. Some of these can also be the sources for intentional poisoning. The toxicity results from the combination of CO with hemoglobin to form carboxyhemoglobin (COHb), leading to severely impaired tissue oxygenation. The CO displaces oxygen from hemoglobin, reducing the delivery of oxygen to the tissues. CO not only has a higher affinity for hemoglobin than oxygen but also shifts the oxyhemoglobin dissociation curve to the left ( Fig. 40.2 ). For this reason, the toxicity of CO poisoning is greater at high altitude and in the presence of anemia. It is critical to remember that although the oxygen content of the arterial blood is low in CO poisoning, the partial pressure of oxygen in arterial blood (Pa o 2 ) is not reduced. Because the carotid body responds to Pa o 2 , ventilation may not be stimulated until acidosis develops. Together with the fact that COHb is bright red, this makes the clinical diagnosis very difficult. The bright red color of the blood also makes the currently available oximeters unreliable. If there is a suspicion of CO poisoning, an arterial blood gas must be obtained along with COHb levels. CO also binds to myoglobin, resulting in anoxia of muscle cells. With prolonged exposure, CO binds to cytochrome oxidase, impairing mitochondrial function and reducing production of adenosine triphosphate. Both of these actions impair normal cellular function.

Pathology
Various pathologic lesions are found in smoke inhalation. Part of the variability in pathology may be attributed to differences in the toxic products generated in fires. However, many of the pulmonary changes may not result simply from the direct chemical injury to the respiratory tract. Rather, they may reflect secondary circulatory, metabolic, or infectious complications of surface burns or may be induced by the administration of oxygen, mechanical ventilation, and the administration of excessive volumes of intravenous fluids.
Animal models of the pathology have been of limited value, because steam rather than pure smoke was usually used, and the modifying effects of the upper air passages were eliminated by using tracheal cannulas. Experimental evaluation of the response to smoke has been performed in anesthetized and intubated sheep, kept light enough to breathe spontaneously. Even in these animals, any protective effects of the upper airway were bypassed. Smoke was generated by burning material such as dyed cotton toweling. The smoke was then insufflated into the lungs. In sheep, a volume of 20 cc/kg for 12 breaths and an inspiratory time of 3–4 seconds produced physiologic effects similar to natural smoke inhalation, including mean COHb levels of 45%.
The initial pathologic changes are tracheobronchitis. The greater the tidal volume of the insufflated smoke, the more intense the tracheobronchitis. With sufficient smoke quantity, denudation of the tracheal epithelium occurred, most likely from disruption of the cell-cell and the cell–basal layer adhesions. With much larger volumes, acute pulmonary edema caused by increased pulmonary vascular permeability occurred within 30 minutes. The degree of atelectasis also correlated with the amount of smoke insufflated. Subsequent airway edema only occurred in those animals given large tidal volumes of smoke. The degree of hypoxemia over the 24 hours studied did not correlate with the tidal breath size but was constant for a given total smoke exposure. This suggests that the gas exchange deterioration was based more on the airway pathology than on the alveolar atelectasis or edema.
A group of infants studied postmortem after accidental exposure to smoke in a newborn nursery was found to have necrosis of bronchial and bronchiolar epithelium with vascular engorgement and edema together with the formation of dense membranes or casts that partially obstructed the large and small airways. Bronchiolitis and bronchopneumonia were present in some, as were interstitial and alveolar edema. There was carbonaceous material in the alveoli with alveolar hemorrhage.
Electron microscopic studies of 10 fatal cases of smoke inhalation following a hotel fire revealed interstitial and alveolar edema as well as engorgement of alveolar vessels. Carbon particles were seen within alveolar macrophages. Type I pneumocytes showed more injury than was seen in the pulmonary endothelial cells. Patients who died after severe surface burns have had necrotizing bronchitis and bronchiolitis with intraalveolar hemorrhage, hyaline membrane formation, and massive pulmonary edema. In these patients, it is difficult to know how much to attribute to direct pulmonary injury from smoke or to the complex metabolic, infectious, chest wall, and circulatory derangements that complicate surface burns and result in acute respiratory distress syndrome (ARDS). However, sheep exposed to surface burns plus smoke inhalation sufficient to raise the COHb levels to 25%–30% fared much worse than the sheep exposed to surface burns alone. The burn/inhalation group had greater amounts of lung lipid peroxidation products in bronchoalveolar lavage and at autopsy even without evidence of pulmonary infection or ARDS.
Pathophysiology
Severe damage to the upper air passages leads to stridor with increased extrathoracic resistance. Upper airway obstruction usually occurs within 24 hours of injury. This increases the work of breathing and can lead to alveolar hypoventilation. Inflammatory changes in the airways lead to ventilation/perfusion mismatch, exaggerating the hypoxemia. Depending on the severity and distribution of the airway obstruction, there may be atelectasis or air trapping. The latter is especially likely with premature closure of the small airways. Altered surfactant function predisposes to atelectasis. Although reflex bronchoconstriction may contribute to the increase in airway resistance, it is difficult to assess the magnitude of its contribution, because airway resistance is already high as a result of bronchial and bronchiolar edema and inflammation.
Smoke is a mixture of gases and particulate matter generated from burning substances. The toxic effects of smoke are primarily seen when animals are exposed to whole smoke. When the particle phase of the smoke was filtered out, there were neither acute nor delayed toxic effects on lung function or gas exchange. Since many of the oxidants are in the gas phase as well in whole smoke, it is possible that only those toxins carried on the smoke particles remain in the airway long enough to elicit an inflammatory response.
Pulmonary edema plays a prominent role in the pathophysiology of lung injury from smoke inhalation. Studies in adults demonstrate increased extravascular lung water without a concomitant increase in pulmonary capillary wedge pressure. Studies in sheep reveal increased lung lymph flow and an increase in the lymph/plasma ratio of protein, suggesting increased permeability of the alveolar capillary membrane. Increased bronchial circulation contributed to the pulmonary edema. Nitric oxide at extremely elevated levels acts as a free radical and potentiates the inflammatory response. Inhibition of inducible nitric oxide synthase reverses the loss of hypoxic pulmonary vasoconstriction and attenuates acute respiratory distress syndrome.
Other products of combustion increase the damage. In dogs, acrolein found in herbicides and cigarette smoke results in delayed-onset pulmonary edema. Pure smoke, and smoke with added hydrochloric acid, did not have the same effects. An animal model showed increased pulmonary vascular resistance and decreased cardiac output concomitant with increased secretion of leukotrienes C4, D4, and E4. Pretreatment with a leukotriene antagonist markedly attenuated and delayed those cardiovascular changes. Additional studies in sheep suggest that the acrolein-induced pulmonary edema and pulmonary hypertension are mediated by cyclooxygenase products, because pretreatment with cyclooxygenase inhibitors blocks the cardiorespiratory changes. The acute pulmonary edema associated with very high-dose cotton smoke exposure in sheep is blocked by a combined cyclooxygenase and leukotriene antagonist, but not by indomethacin, a cyclooxygenase inhibitor. Both agents block the elevated airway resistance and hypoxemia that presumably occur as a result of acute bronchoconstriction. Prolonged exposure to acrolein in a kitchen resulted in severe respiratory distress in a previously healthy 27-month-old child who developed bronchiectasis 18 months after the initial exposure.
Oxidants directly from the products of combustion also contribute to airway damage, closure, and atelectasis. Early therapy with aerosolized deferoxamine-pentastarch in sheep exposed to cotton smoke attenuated these findings. It is not clear whether the deferoxamine was acting directly as an antioxidant or reducing the free iron released in the airway.
Neutrophil-mediated proteolytic activity has been implicated in the airway pathology. Sheep treated intravenously with the synthetic protease inhibitor gabexate mesilate after insufflation with cotton smoke had a significant reduction in transvascular fluid and protein flux and were able to maintain better gas exchange than a vehicle-treated control group. Impaired chemotactic and phagocytic function of the alveolar macrophage after smoke inhalation increases the risk for pulmonary infection several days after the acute event.
Any inhaled cyanide binds to the intracellular cytochrome system, inhibiting cell metabolism and the production of adenosine triphosphate. While all cells contain the enzyme rhodanese, which can convert hydrocyanide to thiocyanate, this capability is outstripped by continued or high levels of cyanide. The thiocyanate is excreted in the urine, assuming normal renal blood flow and urine output.
Clinical Findings
The initial respiratory assessment of a victim of a fire should focus on hypoxemia. Patients may have facial burns, singed nasal hairs, carbonaceous sputum, or soot in the proximal airway. Blistering or edema of the oropharynx, hoarseness, stridor, and upper airway mucosal lesions may be evident. Symptoms include tachypnea, dyspnea, cough, and cyanosis. Chest retractions may be present, and auscultation may reveal wheezing, crackles, or rhonchi. Oxygen should be administered while arterial blood gas studies, including CO levels, are drawn. The clinical manifestations of CO poisoning vary with the level of COHb. Mild intoxication leads to headache, diminished visual acuity, irritability, and nausea. COHb levels greater than 40% produce confusion, hallucination, ataxia, and coma. CO may increase cerebral blood flow, the permeability of cerebral capillaries, and the cerebrospinal fluid pressure. CO may also have long-term effects on the CNS, and myocardial dysfunction and irritability can result directly from CO, as well as from hypoxemia.
Patients assessed immediately at the scene of a fire have elevated blood cyanide concentrations, and the cyanide levels of victims who die because of smoke inhalation are significantly higher than the levels of survivors. Blood cyanide levels correlate with CO levels, and plasma lactate levels were better correlated with cyanide levels than with CO level. A plasma lactate concentration above 10 mM in the emergency department is a sensitive indicator of cyanide poisoning.
Maximum inspiratory and expiratory flow-volume curves and flexible fiberoptic nasopharyngoscopy or bronchoscopy have been helpful in the early assessment of the extent of supraglottic or tracheobronchial injury and the likelihood of subsequently developing airway obstruction. Although there may be some delay in the clinical evidence of respiratory tract injury resulting from smoke inhalation, manifestations of respiratory disease will usually develop within 12–24 hours. Absence of roentgenographic pulmonary disease is not very helpful in early diagnosis, because abnormal findings may lag several hours or more behind auscultatory or physiologic evidence of damage.
Respiratory insufficiency may also occur as the result of airway obstruction anywhere from the supraglottic airways to the alveoli. It may be difficult to localize the level of obstruction; therefore, whenever there is clinical evidence of severe obstruction, the upper airways should be assessed by direct laryngoscopy before swelling of the head, neck, or oropharynx make this examination difficult. Fiberoptic bronchoscopy may be very useful to evaluate the extent of mucosal damage, but intense vasoconstriction in hypovolemic patients may mask the findings.
Acute CO poisoning is also associated with acute myocardial injury and a delayed neuropsychiatric syndrome, which in children will lead to cognitive defects and sometimes to focal deficits. However, the mechanism of action of these complications has not been elicited.
Diagnosis and Differential Diagnosis
Diagnosis of smoke inhalation injury is largely based on history of smoke exposure in a closed space. Facial burns, singed nasal hairs, soot in the nasopharynx, or voice changes may help support the diagnosis. Patients with smoke inhalation with superimposed altered mental status require a careful evaluation for hypoxia secondary to either pulmonary injury or impaired oxygen utilization at the cellular level. Differential diagnoses include acute respiratory distress syndrome, aspiration pneumonitis, pneumonia, asthma, pulmonary embolism, and cyanide poisoning.
Management and Treatment
The initial treatment should focus on reversing CO poisoning, if present, by the administration of high concentrations of humidified oxygen. CO levels may be reduced by half in about an hour when the patient breathes 100% oxygen. If severe CO poisoning is suspected, it is helpful to administer oxygen by nonrebreathing mask at flow rates higher than the victim’s minute ventilation to achieve concentrations close to 100%. If results of clinical examination or arterial blood gas studies suggest alveolar hypoventilation, mechanical ventilation is necessary. Subsequently, the administration of oxygen may be important because of the hypoxemia resulting from bronchiolitis and alveolitis with premature closure of small airways. Constant positive distending airway pressure may also be necessary to maintain reasonable levels of Pa o 2 without using excessively high and potentially toxic concentrations of inspired oxygen for prolonged periods of time.
Controversy exists regarding the importance of, and need for, hyperbaric oxygen in the management of CO poisoning. Hyperbaric oxygen at 2–3 atmospheres markedly hastens the decline of COHb levels, reducing the half-life of COHb to 20–25 minutes. However, the advantages of hyperbaric oxygen therapy may be offset by complications during transfer to the hyperbaric chamber. While some clinicians claim that all patients with significant COHb levels should be sent to a hyperbaric chamber, there is scant evidence for this approach. There are no clear guidelines on the use of hyperbaric oxygen therapy in the treatment of CO poisoning. One review suggests using hyperbaric therapy for children with COHb levels of 25% or greater if symptomatic and for any with levels of 40% or higher. The potential risks of the hyperbaric chamber include oxygen toxicity to the lung, the fact that patients are not amenable to appropriate observation and intervention while they are in the chamber, and the need for tympanotomy. More data are necessary to adequately assess the risks and benefits of hyperbaric oxygen in the therapy of CO poisoning in children related to fires. Systematic reviews have not established whether hyperbaric oxygen therapy given to adult patients with CO poisoning reduces the incidence of adverse neurological outcomes ; consequently, its use in children remains controversial.
Endotracheal intubation may be necessary for a patient with any of the following conditions: (1) severe burns of the nose, face, or mouth, because of the likelihood that nasopharyngeal edema and obstruction will develop; (2) edema of the vocal cords with laryngeal obstruction; (3) difficulty handling secretions; (4) progressive respiratory insufficiency requiring mechanical ventilation; and (5) altered mental status that decreases minute ventilation and diminishes the protective reflexes of the airway. Regardless of whether the glottis is bypassed by endotracheal tube or tracheostomy tube, constant positive airway pressure or positive end-expiratory pressure helps minimize edema and improve oxygenation. Noninvasive positive pressure ventilation may be attempted in patients with acute respiratory decompensation who are otherwise hemodynamically stable, conscious, and without extensive and deep facial burns or trauma. This mode of ventilation has been reported to be effective in avoiding intubation/reintubation in a group of patients with burn-associated acute respiratory failure. However, the risk of upper airway obstruction must be taken into account when selecting patients for noninvasive ventilation.
In addition to the increased airway resistance resulting from edema in and around the walls of airways, it is likely that some reflex bronchoconstriction occurs from irritation of airway receptors. This is more likely to occur in subjects with preexisting lung disease such as asthma or cystic fibrosis or in cigarette smokers. For this reason, it may be reasonable to administer inhaled bronchodilators.
Airway obstruction by cast material containing fibrin often accompanies smoke inhalation injury. In sheep with acute lung injury following burn and smoke inhalation injury, a combination of nebulized heparin and recombinant antithrombin has been demonstrated to improve pulmonary gas exchange and lung compliance, with decreased pulmonary edema, and airway obstruction. In children with burn and smoke inhalation injury, nebulized heparin and N -acetylcysteine significantly decreased incidence of reintubation for progressive pulmonary failure, decreased incidence of atelectasis, and reduced mortality. Similarly, in adults with smoke inhalation injury, nebulized heparin and N -acetylcysteine reduced lung-injury scores.
Coagulation factors should be monitored as significant coagulopathy with prolonged partial thromboplastin time (PTT) has been associated with aerosolized heparin and N -acetylcysteine therapies for inhalation injury. As in many other respiratory conditions, the role of chest physiotherapy is poorly defined. Nevertheless, mucociliary clearance is clearly impaired. The encouragement of deep breathing and cough, or gentle endotracheal suction in the presence of endotracheal intubation, coupled with postural drainage would seem to be reasonable. Using a cough-assist device might be considered.
Although corticosteroids are frequently advocated in the hope of suppressing inflammation and edema, most controlled studies fail to demonstrate a significant effect. Thus, it is difficult to marshal strong support for their use. Furthermore, long-term steroid therapy in victims of fires increases the susceptibility to infection. Until further evidence is available, the empirical use of corticosteroids is discouraged. One review suggests using corticosteroids only for evidence of peripheral airway obstruction, other illnesses requiring steroids, or recent use of steroids. At the experimental level, ibuprofen (but not indomethacin) when given immediately after smoke inhalation injury prevented the development of pulmonary edema. As animal studies further elicit the basic mechanisms of the events occurring within the airways and parenchyma, specific agents may become available to correct the pathophysiology.
Available evidence indicates that antimicrobial agents do not prevent subsequent infection and may only predispose to infection with resistant organisms. Because fever, elevated white blood cell count, and increased erythrocyte sedimentation rate may all result from smoke inhalation and because the chest roentgenogram may show nonspecific opacities that represent either atelectasis or edema, it may be extremely difficult to establish the presence of an infection in the absence of positive blood cultures or a positive Gram stain of airway secretions. It would seem preferable to reserve antimicrobial therapy for patients in whom there is clinical deterioration despite supportive therapy. Changes in the amount, nature, or color of the secretions should raise the suspicion of a bacterial superinfection, which should be confirmed by Gram stain or culture. Because the prevention of infection is clearly an important part of the therapy in victims of fires, aseptic care of the trachea and humidifying equipment is essential. In addition, in a retrospective analysis of children, high-frequency percussive ventilation was associated with a lower incidence of pneumonia.
While dogs benefited from surfactant after smoke exposure, rabbits did not. To our knowledge, there are no data in human subjects. Smoke-exposed sheep did worse with ECMO support than with conventional mechanical ventilation. The ECMO was associated with pulmonary sequestration of leukocytes, increased pulmonary thromboxane B 2 , increased blood-free wet/dry lung weight ratios, and more significant hypoxemia at 24 hours after identical degrees of smoke exposure. Two children with inhalation injury and resultant respiratory failure refractory to conventional mechanical ventilation were successfully treated with ECMO.
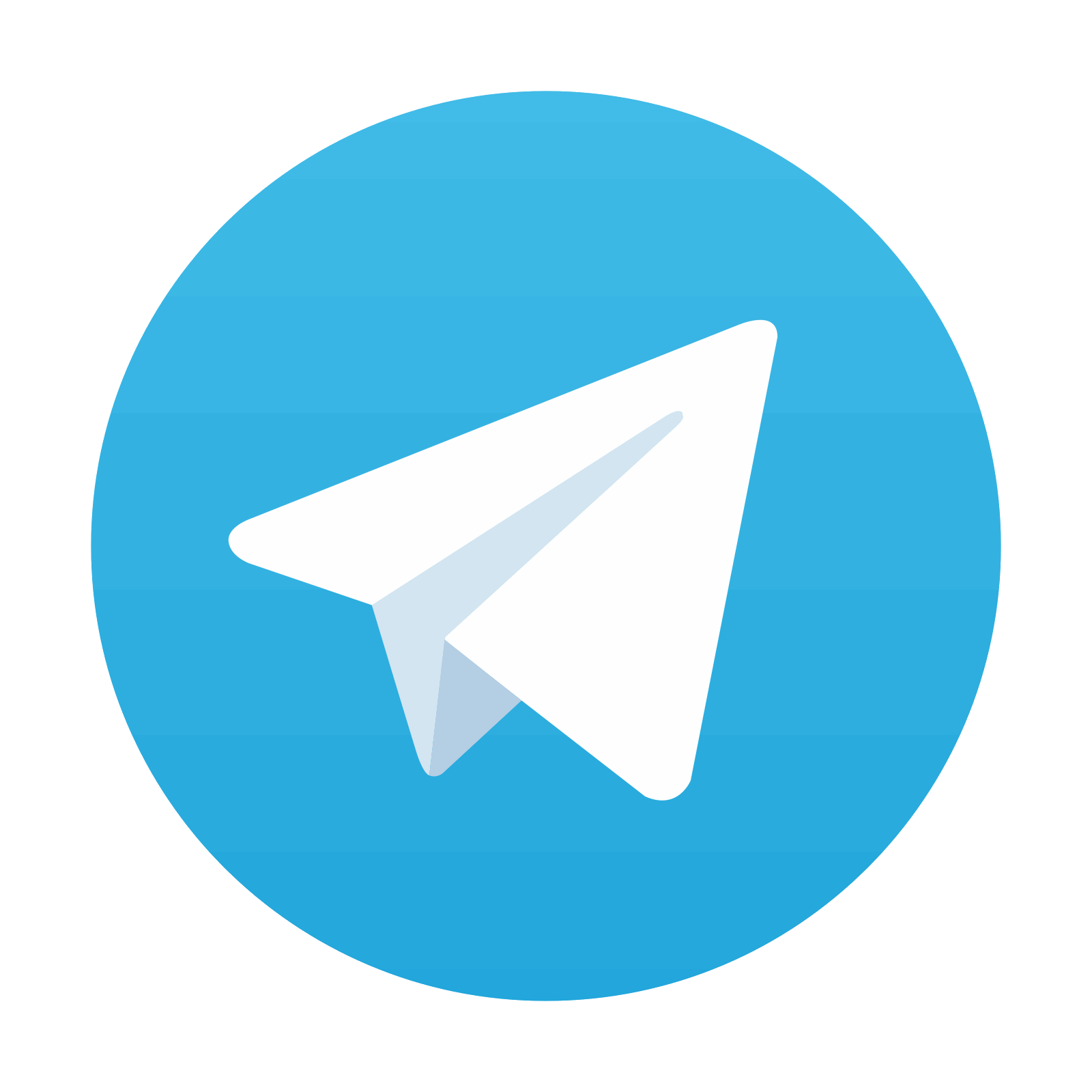
Stay updated, free articles. Join our Telegram channel

Full access? Get Clinical Tree
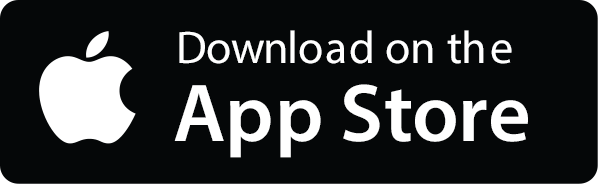
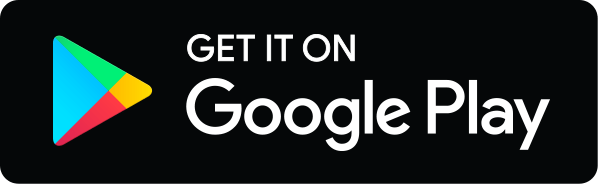